What are carbohydrates?
- Carbohydrates are a class of biomolecules composed primarily of carbon (C), hydrogen (H), and oxygen (O). They are characterized by a hydrogen-to-oxygen atom ratio of 2:1, resembling the composition of water. The general empirical formula for carbohydrates is expressed as Cm(H₂O)n, where the values of m and n can vary. However, it is important to note that not all substances fitting this formula are classified as carbohydrates, nor do all carbohydrates adhere strictly to this ratio. For instance, compounds such as uronic acids and deoxy-sugars may not conform to the typical stoichiometric definition.
- In biochemical contexts, the term “carbohydrate” is often synonymous with “saccharide,” derived from the Ancient Greek word σάκχαρον (sákkharon), meaning “sugar.” Saccharides are further categorized into four chemical groups: monosaccharides, disaccharides, oligosaccharides, and polysaccharides. Monosaccharides and disaccharides, representing the simplest forms of carbohydrates, are commonly referred to as sugars. Typically, names for monosaccharides and disaccharides end in the suffix “-ose,” which is derived from the word glucose (γλεῦκος, gleûkos) and applies to many sugars, including fructose (fruit sugar), sucrose (table sugar), ribose, and lactose (milk sugar).
- The biological functions of carbohydrates are diverse and critical for living organisms. Polysaccharides, for example, serve both as energy reserves—such as starch in plants and glycogen in animals—and as structural elements, with cellulose providing rigidity to plant cell walls and chitin fulfilling similar roles in fungi and arthropods. Monosaccharides like ribose are integral to coenzymes such as ATP (adenosine triphosphate) and play a vital role in the structure of RNA, while deoxyribose is a key component of DNA.
- Carbohydrates are ubiquitous in both natural and processed foods, forming an essential part of human nutrition. Starch, a polysaccharide, is particularly abundant in staple foods like cereals (wheat, maize, rice) and tubers such as potatoes. Sugars in the human diet primarily come from table sugar (sucrose), which is extracted from sugarcane or sugar beets, lactose found in milk, and naturally occurring sugars like glucose and fructose present in honey and various fruits and vegetables.
- Additionally, cellulose is a significant polysaccharide in plant cell walls and constitutes a major component of insoluble dietary fiber. Although humans cannot digest cellulose, it plays a crucial role in promoting digestive health by facilitating bowel movements. Other polysaccharides associated with dietary fiber, including resistant starch and inulin, serve as prebiotics, nourishing specific bacteria in the large intestine. These bacteria metabolize these fibers, yielding beneficial short-chain fatty acids that contribute to overall gut health.
Types of carbohydrates
Carbohydrates can be broadly categorized into three primary types: monosaccharides, disaccharides and oligosaccharides, and polysaccharides.
- Monosaccharides: These are the simplest form of carbohydrates and can be further classified into two categories based on their functional groups:
- Aldoses: These monosaccharides contain an aldehyde group (-CHO). Examples include ribose and erythrose.
- Ketoses: These contain a ketone group (C=O). Dihydroxyacetone phosphate (DHAP) and fructose are prominent examples.
- Monosaccharides are typically characterized by their sweetness and solubility in water, making them readily available for energy metabolism.
- Disaccharides and Oligosaccharides: This category consists of two to ten monosaccharide units linked together by glycosidic bonds. They can be classified based on their reducing properties:
- Reducing Sugars: Maltose and lactose fall into this category as they can donate electrons in redox reactions.
- Non-Reducing Sugars: Sucrose is a prime example and is considered the predominant form of sugar in most vascular plants. Sucrose is often referred to as “the sugar of commerce” due to its widespread use and economic significance. It is commonly found in ripened fruits such as pineapples, apples, mangoes, and grapes, as well as in vegetables like sugarcane, beet roots, tapioca, and sweet potatoes.
- Polysaccharides: These are complex carbohydrates that consist of long chains of monosaccharide units and can be categorized into two types:
- Homo-polysaccharides: Composed of only one type of monosaccharide. Common examples include starch, inulin, cellulose, and glycogen. Starch serves as a primary energy storage form in plants, while glycogen is utilized by animals.
- Hetero-polysaccharides: Made up of different types of monosaccharides or yield a mixture of monosaccharides and derived products upon hydrolysis. Notable examples include hyaluronic acid, chondroitin, vegetable gums, and agar-agar.
- Polysaccharides can serve various functions; some act as storage polysaccharides (like starch and glycogen), while others fulfill structural roles (such as cellulose, hemicelluloses, pectic compounds, chitin, and mucilage).
What Is Sucrose?
Sucrose is the most prevalent disaccharide found in nature and serves as a principal product of photosynthesis in plants. This sugar is classified as a non-reducing sugar, which limits its chemical reactivity, making it an effective molecule for transport and storage within plant systems. Sucrose plays a vital role in plant metabolism and development due to its ability to facilitate the movement of energy and carbon skeletons throughout the plant.
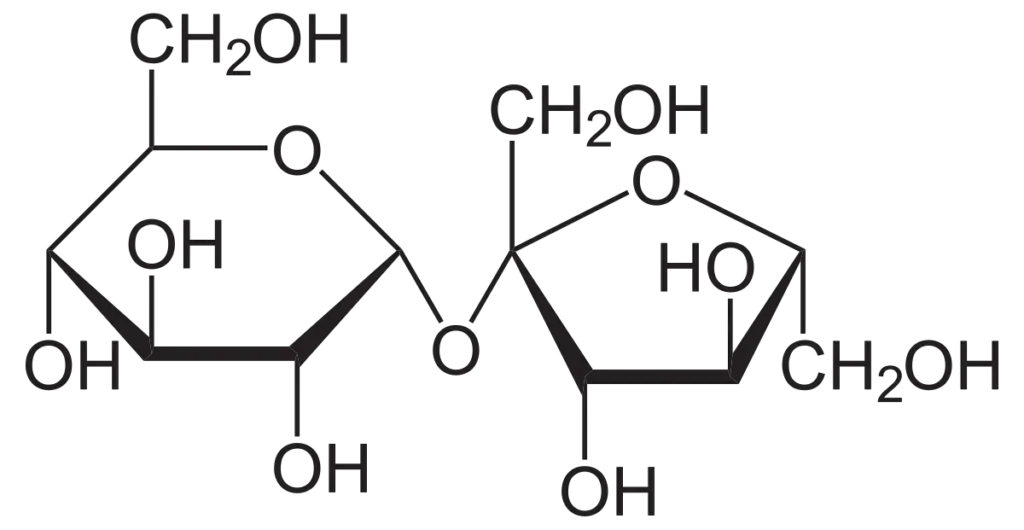
- Synthesis:
- The synthesis of sucrose predominantly occurs in the leaves, although many plant cells possess the capability to produce this sugar.
- The process occurs in the cytosol and involves the sequential action of two key enzymes:
- Sucrose-Phosphate Synthetase (SPS): This enzyme catalyzes the reversible transfer of glucosyl units from UDP-glucose to fructose 6-phosphate, resulting in the formation of sucrose 6-phosphate.
- Sucrose Phosphatase (SPP): SPP then dephosphorylates sucrose 6-phosphate to yield sucrose in an irreversible reaction.
- Therefore, SPS represents the critical regulatory step in the biosynthesis of sucrose. It is subject to allosteric activation by glucose 6-phosphate and inhibition by inorganic phosphate (Pi). Furthermore, SPS contains multiple regulatory phosphorylation sites that modulate its activity.
- Functions:
- Sucrose synthase (SucS), which exists in both soluble and membrane-bound forms, facilitates the synthesis and degradation of sucrose, emphasizing its dynamic role in carbohydrate metabolism.
- The translocation of sucrose from source tissues (where it is produced) to sink tissues (where it is utilized or stored) is crucial for the growth and development of plants.
- Sucrose can be transported symplastically, through plasmodesmata, or apoplastically, via the apoplast with the aid of sucrose transporters.
- Storage and Hydrolysis:
- In sink tissues, sucrose can be stored within cell vacuoles using transporters located at the tonoplast, or it can be hydrolyzed by invertase. This hydrolysis process releases glucose and fructose, which can be readily utilized by the cell for energy and metabolic activities.
- Notably, the translocation of sucrose from source to sink tissues is particularly active during grain development, a stage characterized by the synthesis of storage carbohydrates within the grains.
Biosynthesis of Sucrose
Sucrose biosynthesis is a crucial metabolic process in plants, responsible for synthesizing the disaccharide sucrose, which serves as a primary carbohydrate for transport and energy storage. This process primarily occurs in the cytosol of photosynthetic tissues and germinating seeds, where sucrose is synthesized from two monosaccharides: α-D-glucopyranose and β-D-fructofuranose. The following outlines the step-by-step process of sucrose biosynthesis.
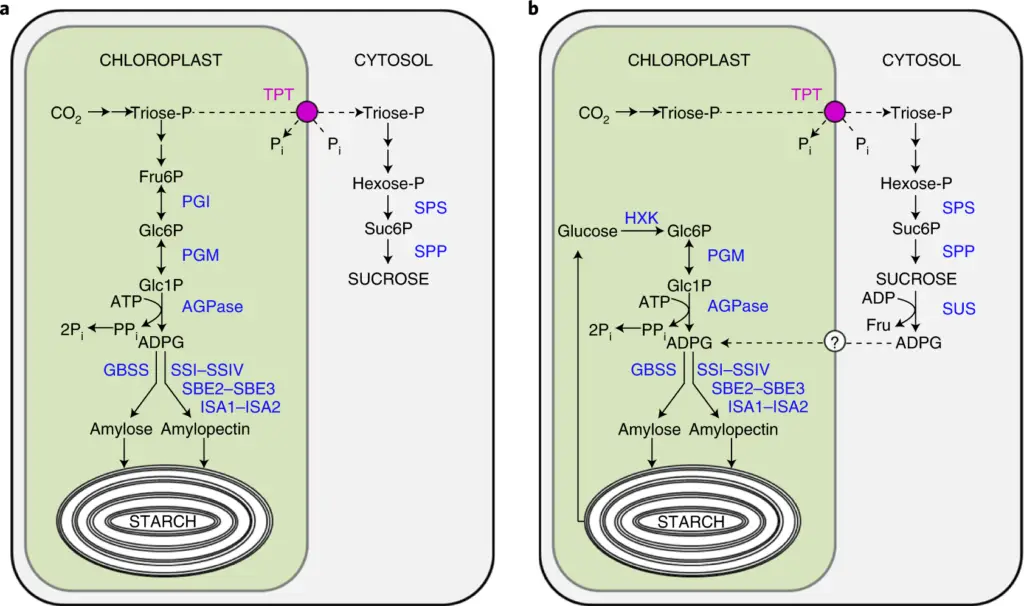
- Formation of Triose Phosphates:
- Sucrose synthesis begins in the chloroplasts through the Calvin cycle, where triose phosphates, specifically glyceraldehyde 3-phosphate (G3P) and dihydroxyacetone phosphate (DHAP), are produced.
- Approximately 1/6 of the triose phosphates formed are transported from the chloroplast to the cytosol, exchanging them for inorganic phosphate (Pi), while the remaining 5/6 are utilized for regenerating ribulose 1,5-bisphosphate (RuBP) to sustain the Calvin cycle.
- Transport of Triose Phosphates:
- The transport of triose phosphates from the chloroplast to the cytosol is mediated by the triose phosphate transporter (TPT), an antiporter located in the inner envelope of plastids.
- TPT facilitates the export of DHAP in exchange for cytosolic inorganic phosphate, maintaining a balance between the Calvin cycle and sucrose biosynthesis.
- Condensation of Triose Phosphates:
- Once in the cytosol, the enzyme aldolase catalyzes the condensation of DHAP and G3P, yielding fructose 1,6-bisphosphate.
- This step is critical as it marks the transition from simple triose phosphates to a more complex sugar structure.
- Conversion to Fructose 6-Phosphate:
- The enzyme fructose 1,6-bisphosphatase then catalyzes the removal of a phosphate group from fructose 1,6-bisphosphate, resulting in fructose 6-phosphate.
- This reaction is irreversible and is exothermic, driving the biosynthetic pathway forward.
- Formation of Glucose 6-Phosphate:
- Fructose 6-phosphate is subsequently converted to glucose 6-phosphate by the enzyme phosphohexoisomerase.
- This reversible reaction is vital for providing the necessary substrates for sucrose synthesis.
- Conversion to UDP-Glucose:
- Glucose 6-phosphate is then converted to glucose 1-phosphate via phosphoglucomutase, a reversible reaction.
- UDP-glucose is generated from glucose 1-phosphate through the action of UDP-glucose pyrophosphorylase, creating a high-energy molecule crucial for subsequent reactions.
- Synthesis of Sucrose 6-Phosphate:
- The activated glucose in the form of UDP-glucose is then transferred to fructose 6-phosphate, catalyzed by sucrose-phosphate synthase (SPS), resulting in the formation of sucrose 6-phosphate.
- This reaction is reversible, depending on the availability of substrates and the energy status of the cell.
- Hydrolysis to Sucrose:
- The final step involves the hydrolysis of sucrose 6-phosphate to produce sucrose and release inorganic phosphate, catalyzed by sucrose-phosphate phosphatase.
- This reaction is irreversible and exothermic, contributing to the driving force of sucrose biosynthesis.
- Regulatory Mechanisms:
- The activities of key enzymes involved in sucrose biosynthesis, such as fructose 1,6-bisphosphatase and sucrose-phosphate phosphatase, are regulated based on the plant’s metabolic needs.
- The overall free energy change (ΔG⁰) for the pathway is approximately 25 kJ/mol, with a significant negative energy change associated with the dephosphorylation of sucrose 6-phosphate (ΔG⁰ = 16.5 kJ/mol).
Regulation of Sucrose Synthesis
The regulation of sucrose synthesis is a complex process that ensures the appropriate production and distribution of sucrose within plant cells. This regulation is influenced by various metabolic factors and involves key enzymes that modulate the biosynthetic pathway. The following outlines the mechanisms and factors involved in the regulation of sucrose synthesis.
- Role of Inorganic Phosphate (Pi) and Triose Phosphates:
- During daylight, photosynthesis occurs actively in chloroplasts, resulting in an accumulation of triose phosphates.
- Simultaneously, the active synthesis of ATP leads to a depletion of inorganic phosphate (Pi) in the chloroplasts.
- A short supply of Pi can inhibit photosynthesis, thereby impacting the production of triose phosphates.
- Exchange Mechanism through TPT:
- The triose phosphate transporter (TPT) operates as an antiporter that facilitates the one-to-one exchange of triose phosphates with cytosolic Pi, maintaining a balance between these metabolites in the chloroplast.
- This exchange is crucial for ensuring a steady supply of triose phosphates for sucrose synthesis in the cytosol.
- Hexose Monophosphate Pool Status:
- The synthesis of sucrose occurs in the cytosol and is regulated by the status of the hexose monophosphate pool.
- Variations in sucrose concentrations throughout the day primarily result from changes in the activity of sucrose-phosphate synthase (SPS).
- Allosteric and Covalent Modulation of SPS:
- SPS activity is subject to both allosteric modulation and covalent modification through phosphorylation.
- Glucose 6-phosphate acts as an allosteric activator of SPS, enhancing its activity, while Pi serves as an inhibitor.
- As the concentration of cytosolic hexose monophosphates increases, the levels of Pi decrease, thereby promoting SPS activity.
- Phosphorylation and Dephosphorylation Dynamics:
- The enzyme’s activity is regulated by the phosphorylation state of specific serine residues.
- The phosphorylation of Ser158, catalyzed by SPS kinase, results in the inhibition of SPS, while dephosphorylation of Ser158 by SPS phosphatase activates the enzyme.
- Notably, the activity of SPS kinase is inhibited by glucose 6-phosphate, whereas SPS phosphatase activity is inhibited by high concentrations of Pi.
- Additional Phosphorylation Sites:
- SPS can also be inhibited by phosphorylation at Ser229 due to its interaction with a 14-3-3 regulatory protein.
- Conversely, phosphorylation at Ser424 leads to the activation of the enzyme.
- Therefore, multiple phosphorylation sites in the SPS protein confer differential regulatory effects on the enzyme’s activity.
- Role of Fructose 2,6-Bisphosphate (F2,6-BP):
- Fructose 2,6-bisphosphate plays a significant role in the regulation of sucrose metabolism by modulating the activity of fructose 1,6-bisphosphatase.
- F2,6-BP is structurally similar to fructose 1,6-bisphosphate but differs in the position of one phosphate group, influencing its regulatory capacity.
- This compound inhibits sucrose biosynthesis by blocking fructose 1,6-bisphosphatase activity.
- Activation of Pyrophosphate-Dependent Enzyme:
- Conversely, pyrophosphate-dependent fructose 6-phosphate kinase, which converts fructose 6-phosphate to fructose 1,6-bisphosphate, is activated by F2,6-BP.
- The concentration of F2,6-BP in plant cells is typically low, with its synthesis being catalyzed by ATP-dependent fructose 6-phosphate 2 kinase (F6P2K).
- Influence of Metabolites on F6P2K Activity:
- The activity of F6P2K is inhibited by triose phosphate, whereas fructose 6-phosphate and Pi serve as activators.
- As a result, lower concentrations of F2,6-BP coincide with the accumulation of triose phosphates, while higher levels of Pi and fructose 6-phosphate promote the synthesis of F2,6-BP, thus regulating sucrose biosynthesis.
- Regulatory Mechanisms of Sucrose Synthesis:
- Sucrose synthesis regulation occurs through both feed-forward and feedback mechanisms.
- The feed-forward mechanism is mediated by triose phosphates, which enhance sucrose synthesis by reducing F2,6-BP levels.
- Conversely, the feedback mechanism inhibits sucrose biosynthesis through the action of fructose 6-bisphosphate and Pi.
Sucrose Catabolism
Sucrose catabolism is a crucial metabolic process in plants, allowing for the efficient utilization of this carbohydrate as an energy source or for storage purposes. The breakdown of sucrose is facilitated by specific enzymes that convert it into simpler sugars, which can be further metabolized or stored as needed. The following outlines the key components and processes involved in sucrose catabolism:
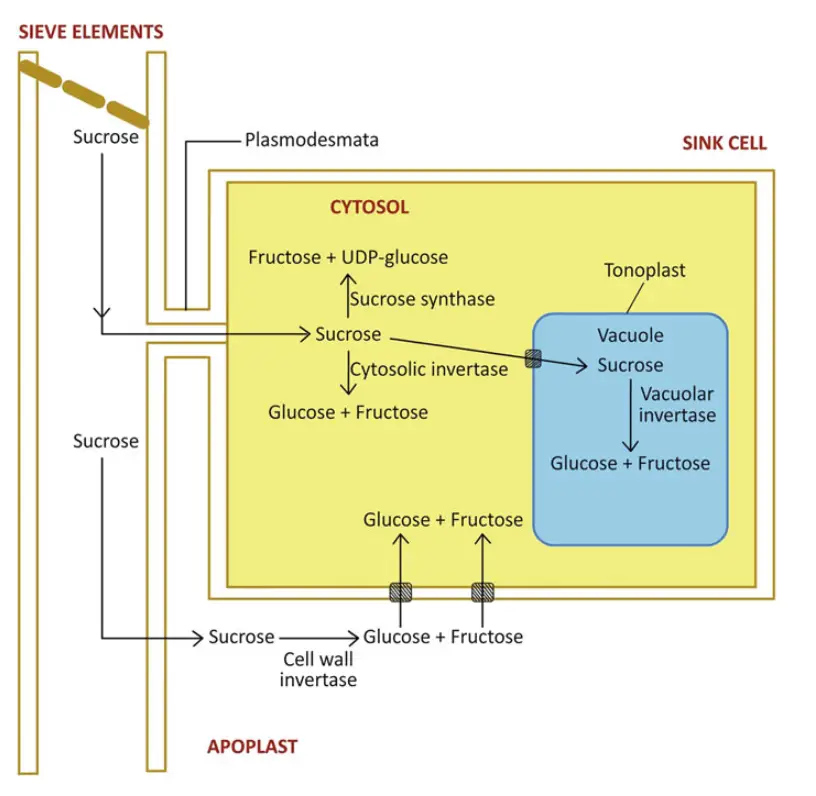
- Role of Sucrose in Plants:
- Sucrose serves as the primary form of carbohydrate transported from photosynthetic tissues (sources) to non-photosynthetic tissues (sinks), where it is either metabolized for energy or stored for later use.
- In plant cells, sucrose is predominantly stored in vacuoles, which offer a larger storage capacity. This is particularly advantageous during periods of increased metabolic demand, such as during environmental stress, when the rate of sucrose consumption surpasses the rate of its entry into metabolism.
- The extensive vacuolar pool of sucrose helps buffer these demands, allowing for a steady supply of carbohydrates to the cytosolic pool, which is consumed more rapidly.
- Transportation and Translocation of Sucrose:
- Sucrose can be transported via apoplastic or symplastic pathways, depending on the plant type and the tissues involved.
- In the phloem, sucrose translocation occurs in sieve-tube elements and is driven by a concentration gradient established by sucrose loading at the source and unloading at the sink.
- In developing grains or germinating seeds, the apoplastic mode of transport is utilized due to the absence of plasmodesmata connections between the embryo and maternal tissues, necessitating the release of sucrose into the apoplast before its transport across the cell membrane.
- Enzymes Involved in Sucrose Catabolism:
- The primary enzymes responsible for sucrose catabolism are invertase and sucrose synthase (SS).
- Invertase hydrolyzes sucrose into glucose and fructose, while SS catalyzes a reversible reaction that can synthesize sucrose as well as break it down. However, SS’s role in sucrose synthesis is less significant compared to sucrose-phosphate synthase (SPS).
- SS plays a more critical role during sucrose catabolism, particularly for energy utilization or for the biosynthesis of starch.
- Isoforms of Invertase:
- Multiple isoforms of invertase exist within plant cells, each with distinct functions and optimal pH levels.
- Apoplastic and vacuolar invertases typically operate optimally at an acidic pH of around 5.0, while cytosolic invertase functions optimally at a neutral to alkaline pH of approximately 7.5.
- Vacuolar invertases are classified as soluble acid invertases, whereas cell wall invertases are categorized as insoluble acid invertases.
- The expression of these isoforms is temporally or spatially regulated during plant development, with high levels of vacuolar invertases observed in tissues that accumulate hexoses, such as developing fruits and rapidly growing regions like root elongation zones.
- Function of Invertase in Metabolism:
- Vacuolar invertases facilitate cell expansion, sugar storage, and the regulation of cold-induced sweetening.
- Upon demand, sucrose is hydrolyzed by vacuolar invertase into glucose and fructose, which are then transported out of the vacuole.
- In apoplastic transport scenarios, sucrose is released into the apoplast and hydrolyzed by apoplastic invertase before being actively transported across the cell membrane.
- Additional Mechanisms of Sucrose Breakdown:
- Sucrose synthase (SS) catalyzes the breakdown of sucrose, utilizing UDP as a substrate to produce UDP-glucose and fructose.
- This process contrasts with the action of invertase, as it does not require ATP for the phosphorylation of the products.
- The UDP-glucose generated by SS is subsequently converted to glucose 1-phosphate by UDP-glucose pyrophosphorylase, utilizing inorganic pyrophosphate (PPi) in the reaction and generating UTP in the process.
- Importance of Phosphorylation and Substrate Channeling:
- The SS-catalyzed reaction produces phosphorylated hexoses through an ATP-independent pathway, which is particularly important in storage tissues like amyloplasts of developing grains and potato tubers.
- Invertase activity is notably low in potato tubers, whereas SS activity is significantly higher, indicating the enzyme’s essential role in tuber development.
- Mutants deficient in SS exhibit poor development in potato tubers and grains, underscoring the enzyme’s functional significance.
- Role in Cellulose Synthesis:
- Beyond sucrose breakdown, SS is also implicated in the synthesis of cellulose and callose.
- Proposed models suggest a direct correlation between SS and the cellulose synthase complex, with UDP-glucose produced by SS being channeled directly to the active site of cellulose synthase.
- The phosphorylation status of SS influences its solubility and localization; phosphorylation renders the enzyme soluble, while dephosphorylation exposes hydrophobic amino acids, leading to membrane binding.
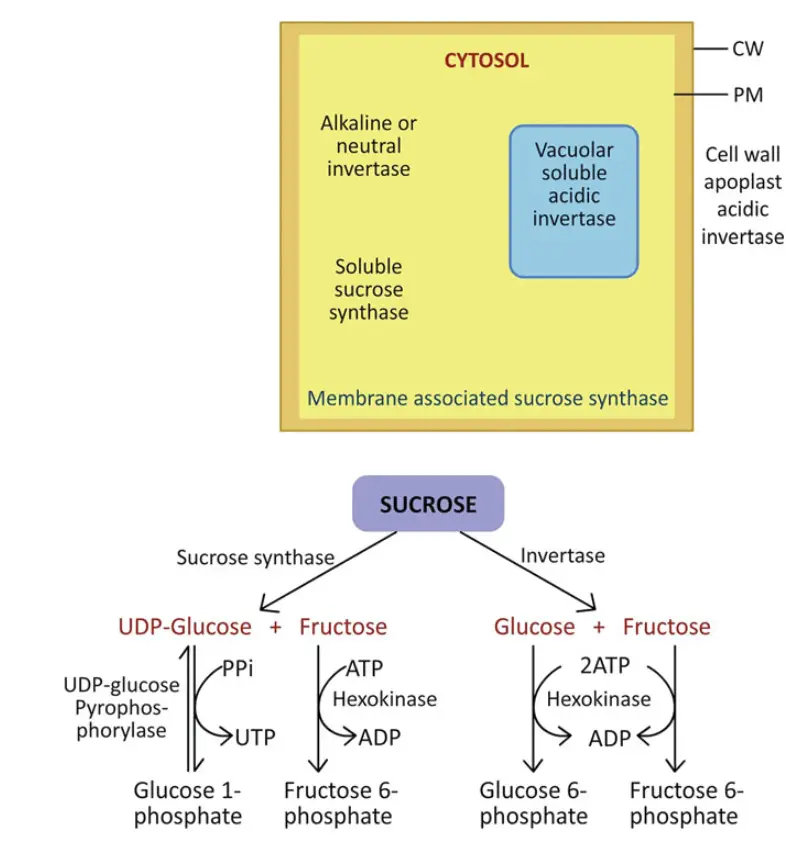
What is Starch?
Starch is a crucial polysaccharide that serves as an essential form of carbohydrate storage in plants. Composed primarily of glucose units, starch exists in two structural forms: amylose and amylopectin. This carbohydrate plays a vital role in energy storage and management within plant cells.
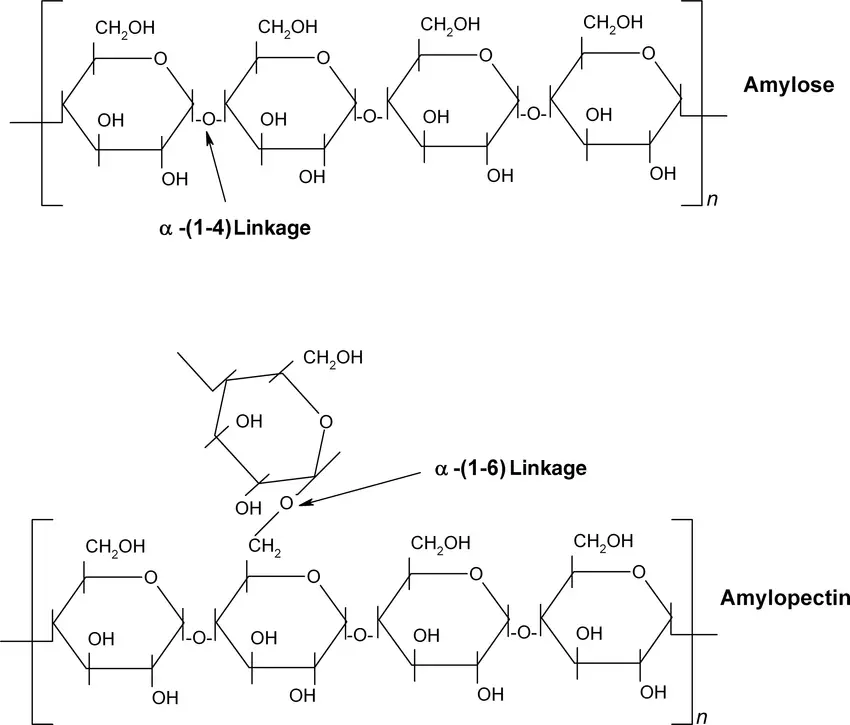
- Structural Composition:
- Starch is an osmotically inert polymer of glucose, which means it does not affect osmotic pressure within cells. This characteristic is significant because it allows for the safe storage of energy without causing excessive water influx that could lead to cell swelling or bursting.
- The granules of starch are massive, compact, and insoluble, often described as semi-crystalline structures. They contain two distinct glucose polymers:
- Amylose: A linear polymer primarily linked by α(1,4) glycosidic bonds.
- Amylopectin: A branched polymer consisting of both α(1,4) and α(1,6) linkages, which create branch points throughout the molecule.
- Functional Importance:
- The presence of starch as a storage product is critical in plants. If an equivalent quantity of hexose units were stored as sucrose in the plastid, the osmotic pressure would increase significantly. This condition would draw water from the cytosol into the plastid, potentially causing it to swell and burst. Therefore, starch serves as a more efficient means of carbohydrate storage.
- Common sources of starch in the plant kingdom include staple crops such as potatoes, wheat, and rice. These plants accumulate starch in various tissues, making it a vital component of the human diet.
- Comparison of Amylose and Amylopectin:
- Amylose:
- It is characterized as a linear polymer of glucose with a smaller molecular size compared to amylopectin, typically comprising 1,000 to 20,000 glucose units.
- Accounts for 30% or less of the total starch content.
- The linear structure gives amylose a tendency to form helical structures.
- Amylopectin:
- It is a branched molecule with a much larger size, containing 100,000 to 1,000,000 glucose residues.
- The branching pattern in amylopectin is not random, with branching points occurring every 24 to 30 glucose residues.
- Comprises 70% or more of the total starch content and is structurally similar to glycogen, which is the energy storage polysaccharide found in animals.
- Amylose:
Starch Biosynthesis
Starch biosynthesis is a critical biochemical process in plants that involves the synthesis of amylose and amylopectin, the two main constituents of starch. These polysaccharides serve as essential energy storage molecules, allowing plants to store glucose units efficiently. The synthesis occurs in the plastids and is a highly regulated process that employs various enzymes to facilitate the formation of glycosidic linkages.
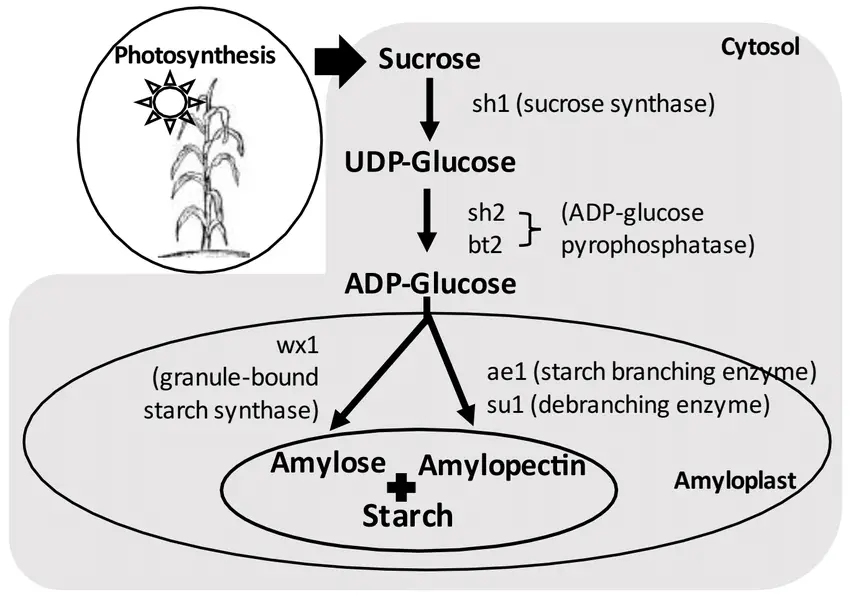
- Synthesis of Amylose (α-(1:4) Glycosidic Linkages):
- The formation of amylose can occur through several enzymatic pathways:
- Hanes (1940) proposed that amylose synthesis involves the enzyme starch phosphorylase, which catalyzes the reaction using glucose-1-phosphate. In this process, an acceptor molecule composed of 3 to 20 glucose units, linked by α-(1:4) glycosidic bonds, acts as the substrate.
- Another pathway involves UDP-glucose transglycosylase (also known as amylose synthetase), which transfers glucose from uridine diphosphate glucose (UDP-G) to an acceptor molecule. This acceptor may contain 2 to 4 glucose units already linked by α-(1:4) bonds or even a pre-existing starch molecule.
- According to Akazawa et al. (1964), glucose derived from the hydrolysis of sucrose by the enzyme sucrase can be transferred to a UDP molecule to form UDP-G. This glucose is subsequently transferred to starch, facilitating amylose synthesis.
- The synthesis of amylose can also occur through the action of D-enzyme, which catalyzes the transfer of two or more glucose units from maltodextrins (polymers of glucose) to a variety of acceptors, such as maltotriose or maltotetrose.
- The formation of amylose can occur through several enzymatic pathways:
- Synthesis of Amylopectin (α-(1:6) Glycosidic Linkages):
- The formation of amylopectin is facilitated by the Q-enzyme, which transfers small chains of glucose units linked by α-(1:4) glycosidic bonds to an acceptor molecule that must consist of at least four α-(1:4) linked glucose units. This process is crucial for establishing the branched structure of amylopectin.
- The α-(1:6) glycosidic bond is formed between the C-1 position of the terminal glucose unit of the donor molecule and the C-6 position of one of the glucose units of the acceptor molecule. This branching is vital for the structural integrity and functional capacity of starch.
Catabolism of Starch
The catabolism of starch is a critical metabolic process through which plants and various organisms convert stored starch into usable glucose units. This breakdown occurs through hydrolysis, involving several enzymes that specifically target different linkages within the starch molecule, ultimately yielding glucose, which serves as an essential energy source.
- Enzymatic Breakdown of Starch:
- The term “diastase” refers to a complex of four enzymes that facilitate starch hydrolysis: α-amylase, β-amylase, R-enzyme, and maltase. Each of these enzymes plays a distinct role in the breakdown of starch.
- Role of Specific Enzymes:
- α-Amylase and β-Amylase:
- These enzymes target the α-(1:4) glycosidic linkages present in both amylose and amylopectin. Their action leads to the degradation of starch into disaccharide units, primarily maltose.
- R-Enzyme:
- This enzyme specifically hydrolyzes the α-(1:6) linkages found in amylopectin. By targeting these branching points, R-enzyme contributes to the complete breakdown of starch into simpler sugars.
- α-Amylase and β-Amylase:
- Conversion of Disaccharides to Monosaccharides:
- After the action of α-amylase and β-amylase, maltose is produced as a key disaccharide product. Subsequently, the enzyme maltase catalyzes the conversion of maltose into individual glucose molecules. This conversion is crucial for mobilizing stored energy in the form of glucose.
- Alternative Pathway via Starch Phosphorylase:
- Another method of starch catabolism involves the enzyme starch phosphorylase, which catalyzes the following reaction:
- Starch + Phosphate — (Phosphorylase) —> Glucose-1-Phosphate.
- In this reaction, starch is phosphorylated to yield glucose-1-phosphate, which can be further utilized in metabolic pathways.
- Another method of starch catabolism involves the enzyme starch phosphorylase, which catalyzes the following reaction:
- Conversion of Glucose-1-Phosphate:
- The glucose-1-phosphate produced can then be converted into glucose through the action of the enzyme phosphatase. This conversion ensures that glucose is available for cellular processes, such as glycolysis or energy production through respiration.
- Mondal, Dr Sumanta. (2017). Carbohydrates metabolism. 10.13140/RG.2.2.34187.39209.
- https://faculty.ksu.edu.sa/sites/default/files/cho5.pdf
- https://www.deshbandhucollege.ac.in/pdf/resources/1585200541_BT(H)-VI-Plant_Metabolism-Carbohydrate_metabolism.pdf
- https://www.slideshare.net/slideshow/starch-sucrose-metabolismpdf/251854237
- https://redox-college.s3.ap-south-1.amazonaws.com/kmc/2020/Apr/14/wqi0z1fQtGJJZ9MSiuoe.pdf
- https://people.wou.edu/~guralnl/gural/330Sucrose%20&%20Starch%20synthesis.pdf
- https://mgcub.ac.in/pdf/material/2020042810553621e311a9a5.pdf
- https://conursing.uobaghdad.edu.iq/wp-content/uploads/sites/20/2019/09/Biochemistry-2-CHOmetabolism-Lec-1234.pdf
- https://global.oup.com/us/companion.websites/fdscontent/uscompanion/us/static/companion.websites/9780199730841/McKee_Chapter8_Sample.pdf
- https://gmcsurat.edu.in/lib/exe/fetch.php?media=biochemistry:carbohydrate_metabolism_compatibility_mode_.pdf
- https://uomustansiriyah.edu.iq/media/lectures/3/3_2022_05_30!09_25_42_AM.pdf
- https://nios.ac.in/media/documents/dmlt/Biochemistry/Lesson-03.pdf