What is Bacterial Conjugation?
- Bacterial conjugation is a process through which genetic material is transferred directly from one bacterial cell to another. This mode of genetic exchange involves cell-to-cell contact facilitated by structures known as conjugative pili or sex pili. These extracellular appendages serve as bridges, enabling the transfer of DNA from a donor cell to a recipient cell. Unlike other mechanisms of horizontal gene transfer, such as transformation and transduction, conjugation uniquely relies on direct physical contact between cells.
- During conjugation, the donor cell transmits a conjugative genetic element, which is most commonly a plasmid, though transposons or parts of the chromosomal DNA may also be transferred. In Escherichia coli, a well-studied model organism for this process, conjugation is frequently mediated by the F plasmid (fertility plasmid). This genetic material is either advantageous, providing traits like antibiotic resistance or metabolic capabilities, or occasionally parasitic in nature, potentially burdening the host.
- Conjugation was first described in 1946 by J. Lederberg and E. L. Tatum in E. coli. They demonstrated that genetic material could be exchanged between bacterial cells, resulting in progeny with genetic markers from both parental cells. This discovery was pivotal in understanding bacterial genetic transfer and its implications for genetic diversity and adaptability.
- The conjugation process is a programmed sequence of events. Initially, the donor cell recognizes a compatible recipient and forms a pilus. Once contact is established, a relaxase enzyme initiates the transfer by nicking the DNA at a specific origin of transfer. A single strand of the DNA is then guided through the pilus into the recipient cell. The donor and recipient replicate their respective single-stranded DNA copies to produce double-stranded forms, completing the process. This unidirectional transfer, from donor to recipient, typically takes several minutes but can extend to hours depending on the size of the genetic material.
- Conjugation is not limited to a single species. It occurs in both Gram-positive and Gram-negative bacteria and can even bridge the genetic exchange between bacteria and other organisms, such as plants. Broad-host-range plasmids, which facilitate this process, are valuable tools in molecular biology for introducing recombinant genes into bacteria resistant to other transformation methods.
- Variants of conjugation, such as those observed in Mycobacterium smegmatis (distributive conjugal transfer) and spontaneous zygogenesis in E. coli, differ from classical models. These involve more extensive mixing of parental genomes, suggesting that conjugation can be highly adaptable and complex across different bacterial species.
- Through conjugation, bacteria acquire genetic traits that enhance survival, including resistance to antibiotics and the ability to metabolize novel compounds. This process underscores the adaptability of bacterial populations and their capacity for genetic innovation in response to environmental pressures.
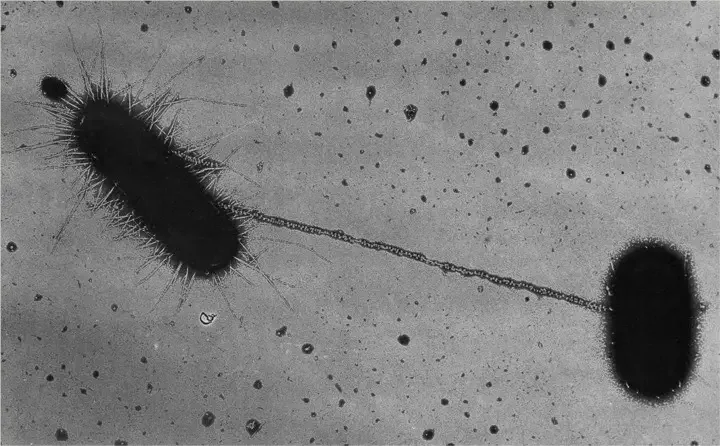
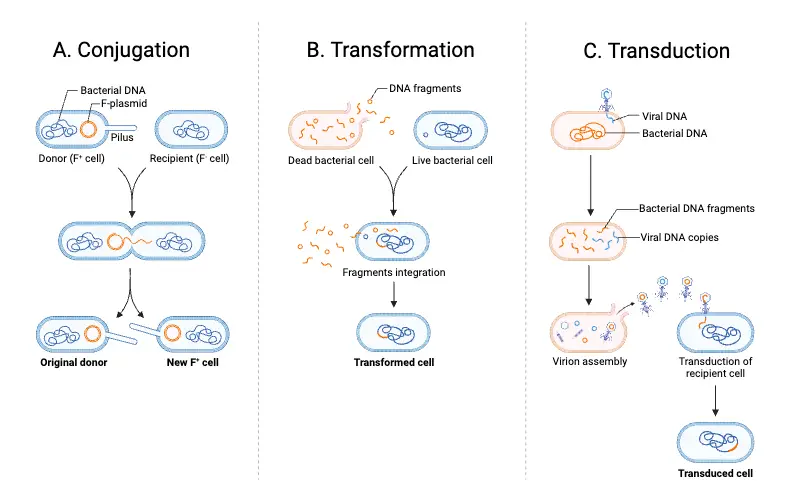
Bacterial Conjugation Definition
Bacterial conjugation is a process of genetic exchange where DNA is transferred from a donor cell to a recipient cell through direct contact, typically mediated by a pilus. It is a key mechanism of horizontal gene transfer, often involving plasmids, and contributes to genetic diversity and the spread of traits like antibiotic resistance.
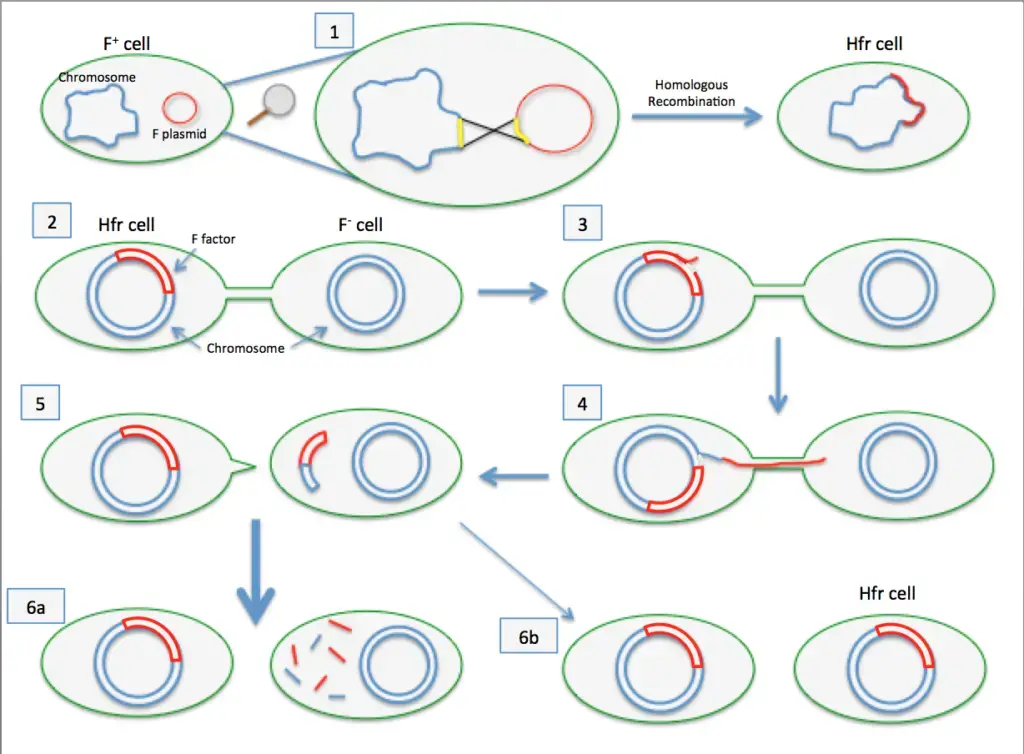
Principle of Bacterial Conjugation
The principle of bacterial conjugation lies in the direct transfer of genetic material from a donor cell to a recipient cell through physical contact. This process is mediated by a conjugative pilus, a structure specialized for forming a bridge between the two cells. The donor cell contains a specific genetic element, such as a plasmid, that encodes the machinery necessary for conjugation.
One of the best-studied examples is the F (fertility) plasmid in Escherichia coli. Cells containing the F plasmid are referred to as donor cells (F+), while those lacking it are recipient cells (F–). The F plasmid is a large DNA molecule, approximately 100 kilobases in size, present in one or two copies per donor cell. It encodes the genes responsible for pilus formation, DNA transfer, and the initiation of replication within the recipient cell.
For conjugation to occur, donor and recipient cells must establish close contact through the pilus, enabling the formation of a mating pair. Enzymatic actions facilitate the fusion of the membranes of the two cells. During this process, the donor’s DNA undergoes replication, with a single-stranded copy of the plasmid being transferred into the recipient cell. The recipient cell then synthesizes a complementary strand to form a complete, double-stranded plasmid.
This transfer mechanism is unidirectional, moving DNA from the donor to the recipient. The recipient, now harboring the F plasmid, gains the ability to function as a donor in subsequent conjugative events. This process not only ensures the propagation of genetic material but also enhances genetic variability and adaptability within bacterial populations.
Stages of Bacterial Conjugation
Bacterial conjugation is a well-defined process that involves several crucial steps. These stages are vital for the effective transfer of genetic material between donor and recipient cells. The process primarily involves direct contact between cells, followed by DNA transfer, which can be broken down into distinct phases.
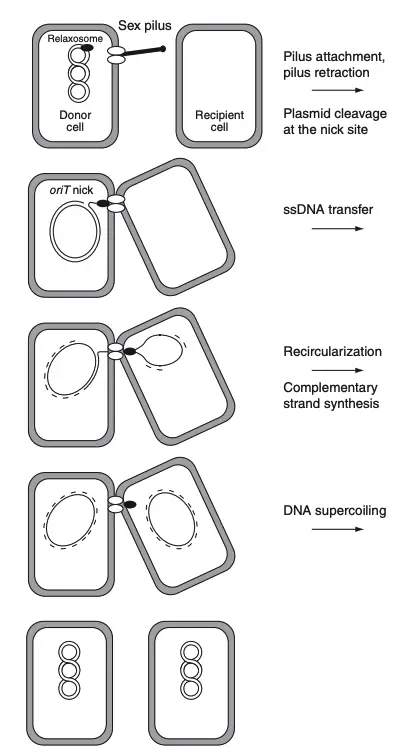
1. Cell-to-Cell Contact
- The first step in conjugation is the physical attachment of the donor and recipient cells.
- In Gram-negative bacteria, this contact is facilitated by specialized pili, which are protein structures that connect the two cells. The genes responsible for producing these pili are typically part of a large operon, such as the tra/trb operon in E. coli.
- For the F plasmid in E. coli, the pili are produced by around 15 gene products. These pili have an outer diameter of 5-12 nm and may contain a hollow central core.
- In Gram-positive bacteria, like Enterococcus faecalis, pili are not involved. Instead, a pheromone produced by the recipient cell triggers the donor cell to produce an aggregation substance, causing the cells to clump together.
2. Formation of the Relaxosome
- Once the cells are in close contact, the next step is the preparation of the DNA for transfer.
- This preparation involves the formation of a complex known as the relaxosome, which occurs at a specific DNA region called oriT.
- At oriT, the DNA strand is nicked by a nicking enzyme, which attaches covalently to the 5′ end of the nicked strand. This complex, sometimes referred to as relaxase, destabilizes the DNA duplex to allow the strand to be transferred.
- Helicase activity also plays a role in unwinding the DNA, further facilitating the transfer.
3. DNA Transfer
- In this phase, a single strand of DNA is transferred from the donor to the recipient cell.
- The transfer is mediated by genes encoding for mobilization (Mob) and type IV secretion system (T4SS), which help move the DNA strand into the recipient.
- The transferred strand contains the 5′ end of the DNA, which is covalently linked to the relaxase. Once the transferred DNA reaches the recipient cell, a second nick is made, and rolling circle replication occurs in the donor.
- This replication results in the formation of a new DNA strand in the recipient, completing the transfer process.
4. Donor Control of Conjugation
- Conjugation is tightly regulated by the donor cell. Most conjugative systems are negatively regulated to ensure that not all cells in a population will transfer DNA at the same time.
- When a donor cell expresses the transfer functions, conjugation can occur, but the system is typically repressed in most cells.
- If a donor cell successfully transfers its genetic material, the recipient may then rapidly propagate the plasmid, allowing the gene transfer process to spread through the population. However, after a period of time, the conjugative system becomes repressed again, maintaining a balance in gene expression.
Key Steps in Bacterial Conjugation
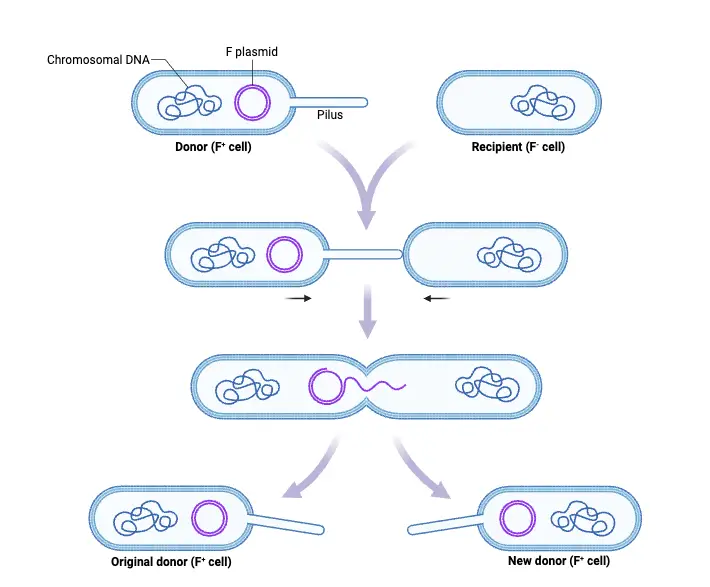
- Formation of Pili on the Donor Cell
The process begins in F+ bacterial cells that carry the F plasmid. The tra locus on the plasmid encodes proteins responsible for pilus formation. These pili extend from the donor cell’s surface, facilitating initial contact with the recipient (F–) cell. - Attachment of Pili to Recipient Cell
Pili proteins adhere to the surface of the F– cell. While the pili establish a physical connection between the two cells, they do not directly mediate plasmid transfer. - Initiation of Membrane Fusion
At the base of the pilus, the traD enzyme plays a crucial role. It enables the fusion of the donor and recipient cell membranes, creating a channel for plasmid transfer. - Nick Formation in the Conjugative Plasmid
The enzyme relaxase introduces a single-strand nick in the F plasmid at a specific site called the origin of transfer (oriT). This marks the starting point for DNA transfer. - Transfer of the T Strand
The nicked DNA strand, known as the T strand, is unwound and transferred from the donor to the recipient in the 5’ to 3’ direction. - Synthesis of Complementary DNA Strands
In both the donor and recipient cells, complementary strands are synthesized, restoring the plasmid to its double-stranded form. As a result, both cells now possess the F plasmid and function as F+ cells. - Integration of the F Plasmid into the Chromosome (Hfr Formation)
Occasionally, the F plasmid integrates into the bacterial chromosome, producing a high-frequency recombination (Hfr) cell. This integration occurs when the circular F element breaks and aligns itself as a linear segment within the chromosome. - Transfer of Chromosomal DNA from Hfr Cells
When conjugation occurs with an Hfr cell, portions of the bacterial chromosome can be transferred to the recipient. However, this process is less frequent, occurring in about one in 10,000 F+ cells. - Replication and Transmission of the Integrated F Element
The integrated F element in Hfr cells replicates along with the bacterial chromosome and is passed to subsequent generations during cell division.
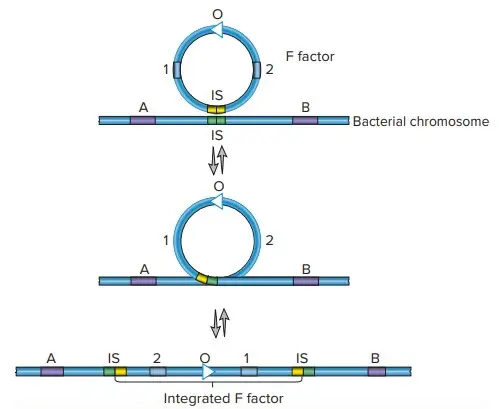
Grouped Requirements for Bacterial Conjugation
Bacterial conjugation involves several key processes, each of which requires specific elements for successful DNA transfer. While variations exist among bacterial subgroups, such as those within Enterobacteria, there are core requirements that are consistent across many species. These requirements can be categorized into two primary groups: those that can transfer DNA autonomously (conjugative) and those that require external assistance (mobilizable).
Key Requirements for Bacterial Conjugation
- oriT (Origin of Transfer)
The essential DNA sequence for conjugation is oriT, often called bom in older literature. This site is where the DNA transfer begins. If the cell contains conjugative functions, any DNA attached to oriT is transferable, even if the element carrying the oriT site is non-conjugative. The DNA at this site is nicked by specific enzymes, and this process is known as plasmid or chromosome mobilization. The exact location of the nick within oriT is referred to as nic. - Nickase or Relaxase (DNA Nicking Enzyme)
The nickase enzyme, also known as relaxase, is crucial for recognizing and cutting the DNA at the oriT site. This enzyme is typically part of a larger complex, known as the relaxosome, which is responsible for DNA processing during transfer. Examples of relaxases include TraI of the F factor, TrwC of R388, MobA of R1162, and NikK of ICEBsI. - Coupling Protein
The coupling protein facilitates the interaction between the relaxase and the transport apparatus that will move the DNA to the recipient cell. The coupling protein is a vital mediator in the transfer process. For example, TraD of the F factor, TraG of RP4, and VirD4 of Ti plasmids serve this function. - Mating Pair Formation (MPF) Proteins
MPF proteins form the intercellular transport apparatus responsible for moving DNA from the donor to the recipient cell. These proteins are part of a complex system called the Type IV Secretion System (T4SS). Some key proteins include TraC of F, TraE of RP4, VirB4 of Ti plasmids, and ConE of ICEBsI. T4SS is responsible for the transfer of both DNA and, in some cases, proteins between bacterial cells. - Integrases (Int)
Integrases are important for conjugational elements that are chromosomally located. These enzymes help the elements excise from the donor chromosome and reintegrate into the recipient chromosome. Integrases recognize specific sequences at both ends of the element for excision and circularization, and often also recognize sequences in the recipient chromosome for reintegration. - Mobilization in Trans vs. Mobilization in Cis
Conjugation is classified into two types based on how the transfer occurs:- Mobilization in trans happens when a conjugative plasmid provides the transfer functions for a non-conjugative element. The transfer process is mediated by proteins from another plasmid.
- Mobilization in cis occurs when a conjugative element integrates into a non-conjugative replicon, enabling both to be transferred simultaneously during conjugation.
Different Configurations of Conjugative Functions
Conjugation functions can vary significantly depending on the configuration of the transfer system, which can involve plasmids, the bacterial chromosome, or specialized elements. Each configuration plays a critical role in the mobility of genetic material, affecting processes like gene transfer and recombination.
Hfr (High Frequency of Recombination)
- Hfr configurations involve a transfer system where a conjugative plasmid integrates into the main bacterial chromosome.
- Cis-mobilization refers to this integration, where the transfer system remains on the chromosome, leading to high recombination rates among transconjugants.
- The phenomenon of transfer gradient occurs, where genes located closer to the origin of transfer (oriT) are transferred more frequently than those located farther away.
- The transfer process can break down DNA during extended conjugation, which causes distal markers (those far from oriT) to be transferred less frequently than proximal markers.
- Hfr configurations have been instrumental in constructing genetic maps of organisms like E. coli and Salmonella enterica.
F-prime Factors (F′)
- F-prime factors are a result of abnormal excision of a conjugative plasmid from the chromosome, typically in an Hfr strain.
- These factors carry DNA segments from the host chromosome, which can be transferred along with the plasmid.
- F′ factors are autonomous, meaning they can carry their own conjugation functions, and also allow for partial diploidy (merodiploidy) of the chromosome regions they carry, such as in the case of the F′-lac factor that includes the lac operon of E. coli.
- F′ factors are useful in genetic studies, particularly in researching gene regulation and dominance.
Transient Interactions and Cointegration
- In some instances, conjugative plasmids may transiently interact with the chromosome or other plasmids, leading to mobilization without stable integration.
- This can happen through homologous recombination in regions with limited DNA homology or through cointegration, where a transposable element facilitates plasmid integration.
- If there is significant homology between the plasmid and the target replicon (more than several kilobases), recombination events create an equilibrium between the plasmid being integrated and remaining in an autonomous state.
- This dynamic results in both mobilization and standard plasmid transfer happening within the same population of cells.
Integrative Conjugative Elements (ICE)
- ICEs are genetic elements found in bacteria that are capable of conjugation but are located on the bacterial chromosome.
- These elements are found in various Gram-positive species like Enterococcus faecalis and Clostridium difficile, as well as in some Gram-negative species like Bacteroides and Haemophilus.
- ICEs excise from the chromosome in the donor cell and can reintegrate into the recipient’s chromosome. This excision is often mediated by an integrase enzyme at specific boundary sites, but it can also occur through the action of a transposon.
- In some cases, the excised ICE interacts with other plasmids to cause cotransfer—where both the ICE and the plasmid are transferred together to the recipient.
Mobile Genomic Islands (MGI)
- MGIs are genetic elements similar to mobilizable plasmids but are located within the chromosome and lack replication functions.
- These islands can excise from the chromosome through an integrase-mediated process, forming circular, non-replicating molecules.
- Though MGIs cannot replicate on their own, they can be transferred through conjugation if the appropriate conjugative functions are available elsewhere in the cell. This transfer depends on the presence of functional oriT sites on the mobile element.
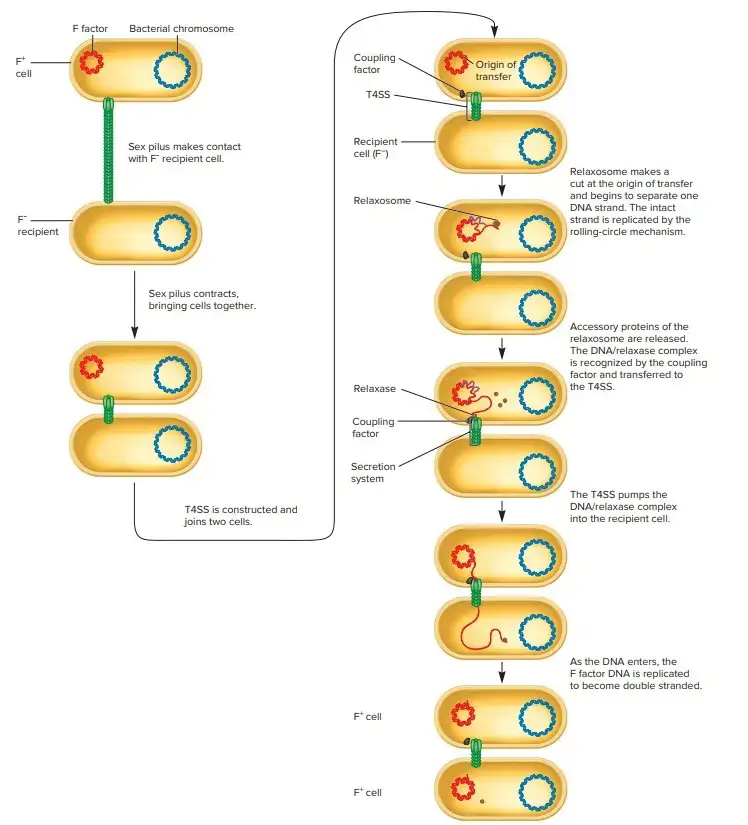
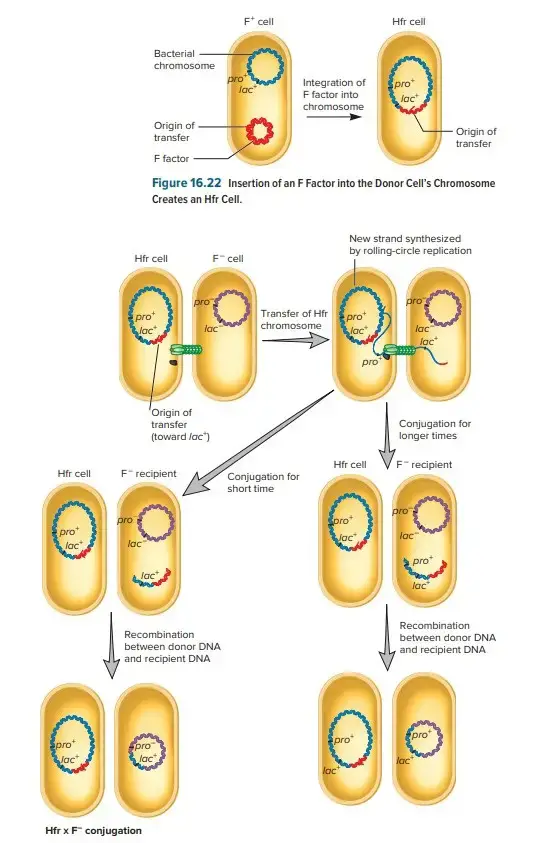
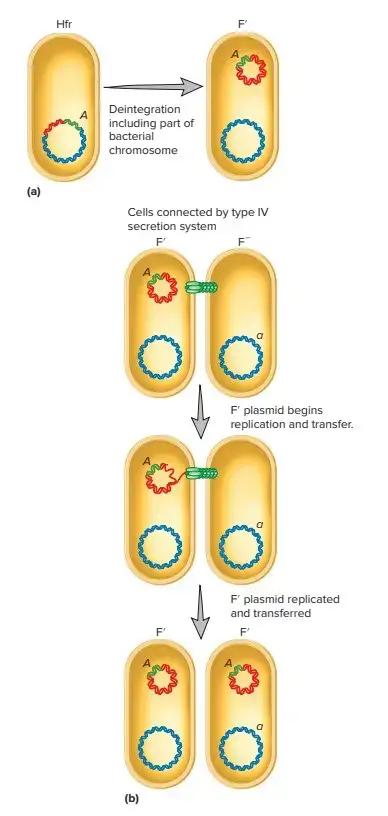
Features of Recipient
The recipient cell plays a crucial role in the conjugation process. Several features and functions determine the cell’s ability to receive and process genetic material from a donor. These features are largely related to the entry and subsequent survival of the transferred DNA.
Enabling Entry
- A key requirement for a recipient is the ability to support the replication of incoming DNA, particularly single-stranded DNA.
- Primase enzyme is essential for this, as it provides the RNA primer necessary for DNA synthesis.
- In some cases, the primase function is part of a protein complex that is transferred along with the DNA.
- Compatibility between the recipient’s expression signals and those of the incoming DNA is critical for successful propagation. This is especially important for plasmids, where the recipient must be able to synthesize the necessary replication proteins.
Barrier Functions
Recipients deploy mechanisms to regulate genetic exchange, including two primary types of barriers:
- Donor-specific exclusion genes
- These genes determine the cell’s ability to receive genetic material from a donor.
- They typically prevent donor cells from acting as recipients, particularly when they have the same genetic makeup.
- Genes responsible for pili production or T4CP (such as TraG of F plasmid) can interfere with the mating pair formation, effectively aborting the transfer from another identical donor.
- Under certain conditions, like late stationary phase, donor cells may transiently act as recipients, a phenomenon called F-phenocopy, until they are allowed to resume vegetative growth.
- For conjugative transposons in E. faecalis, donor cells may not exhibit entry exclusion, allowing them to serve as both donor and recipient.
- Host-dependent restriction–modification systems
- These systems cleave DNA that is not appropriately modified.
- Restriction enzymes recognize modified or unmodified bases in transferred DNA and cut it, preventing successful gene transfer.
- There are different types of restriction systems (types I–IV), and prokaryotic cells often express multiple systems at once.
- Restriction can be significant even though conjugative DNA enters as single-stranded, as the conversion to double-stranded form is rapid, providing an opportunity for restriction to act.
- This restriction is observed in various taxa and affects the success of conjugation, even though earlier studies indicated that conjugative DNA was not restricted in the same way as phage or transformed DNA.
Conjugative Element Countermeasures
Several countermeasures ensure that the recipient cell can successfully receive genetic material despite the restrictive barriers:
- Anti-restriction functions (Ard)
- Many conjugative plasmids contain genes that protect against restriction systems, specifically targeting type I restriction enzymes.
- These genes can be transferred early during conjugation and help ensure the success of DNA transfer.
- Ard proteins have been shown to inhibit multiple families of type I restriction enzymes, improving the chances of successful DNA transfer by overcoming the host’s defense mechanisms.
- Inhibition of SOS induction
- During conjugation, transferred single-stranded DNA can trigger the SOS DNA damage response, which may lead to genome instability and mutagenesis.
- The psi genes, such as psiB, inhibit SOS induction by suppressing the activity of RecA, which would normally trigger a cascade of DNA repair mechanisms.
- This inhibition helps prevent genome rearrangements, prophage induction, and potential cell death during the conjugation process.
Examples of Bacterial Conjugation
Below are examples of bacterial conjugation that illustrate its diverse impact on various organisms.
- Agrobacterium tumefaciens and Plant Tumor Formation
- Agrobacterium tumefaciens is a bacterium that causes crown gall disease in plants.
- The bacterium transfers part of its Ti (tumor-inducing) plasmid into plant cells through conjugation.
- This transferred DNA, known as T-DNA, becomes integrated into the plant’s genome.
- The result is uncontrolled cell division, which leads to tumor formation.
- Shigella spp. and Antibiotic Resistance
- Shigella species are responsible for dysentery in humans.
- They often harbor R plasmids, which encode antimicrobial resistance genes.
- Conjugation enables the transfer of these plasmids between Shigella bacteria and potentially other species.
- This exchange of genetic material contributes to the spread of antibiotic resistance, complicating treatment strategies.
- Escherichia coli and Antibiotic Resistance Transmission
- Escherichia coli (E. coli) can acquire resistance to antibiotics through conjugation, especially in clinical settings.
- Resistance genes carried on plasmids, such as those found in E. coli strains, can be transferred to other bacteria, leading to the rapid spread of resistance traits in bacterial populations.
- Pseudomonas aeruginosa and Genetic Adaptation
- Pseudomonas aeruginosa is known for its adaptability in various environments, particularly in hospitals.
- It can acquire genes for resistance to multiple drugs via conjugation, aiding its survival in the presence of antibiotics.
- The ability of this bacterium to transfer genetic material is a key factor in its persistence in healthcare environments.
Importance of Bacterial Conjugation
The following points highlight the key importance of conjugation in bacterial evolution and environmental interactions.
- Rapid Evolution and Adaptation
- Conjugation enables bacteria to rapidly exchange genetic information, which accelerates evolution.
- This allows bacteria to adapt quickly to new environmental challenges, such as exposure to antibiotics or changes in available nutrients.
- Bacteria can develop traits that confer survival advantages, even in hostile environments like soil, water, or within host organisms.
- Spread of Antibiotic Resistance
- A major concern with conjugation is its role in spreading antibiotic resistance.
- Bacteria exchange plasmids containing resistance genes, which can grant new strains the ability to withstand multiple antibiotics.
- This rapid spread of resistance complicates treatment strategies, particularly in hospital settings, where resistant bacteria can proliferate and cause outbreaks.
- Ecological Impact on Microbial Communities
- Conjugation occurs not only in pathogenic bacteria but also in environmental bacteria, enhancing genetic diversity.
- This genetic exchange helps bacteria acquire beneficial traits, such as improved metabolic pathways or the ability to resist environmental stressors.
- As a result, bacterial communities, both pathogenic and non-pathogenic, become more resilient and capable of thriving in diverse ecological niches.
FAQ
What is meant by conjugation bacteria?
Conjugation bacteria refers to a process of genetic exchange between bacteria where genetic material, typically in the form of plasmids, is transferred from one bacterium (donor) to another (recipient) through direct cell-to-cell contact.
What is the process of bacterial conjugation?
Bacterial conjugation involves several steps:
a) The donor bacterium, which contains a conjugative plasmid, forms a physical connection called a conjugation bridge or pilus with the recipient bacterium.
b) The plasmid DNA is transferred from the donor to the recipient through the conjugation bridge.
c) The transferred plasmid DNA integrates into the recipient bacterium’s genome or remains as an extrachromosomal element.
d) The recipient bacterium now becomes a donor and can transfer the plasmid to other bacteria in subsequent conjugation events.
What is bacterial conjugation and its mechanism?
Bacterial conjugation is a mechanism of horizontal gene transfer in bacteria, allowing the transfer of genetic material, such as plasmids, between bacterial cells. The process involves the physical contact and formation of a conjugation bridge between the donor and recipient bacteria, followed by the transfer of genetic material through this bridge.
What are the two types of bacterial conjugation?
The two types of bacterial conjugation are:
a) Fertility or F-factor conjugation: In this type, the donor bacterium contains an F-plasmid (also called an F-factor) that carries genes for the formation of a conjugation bridge and other conjugative functions.
b) HFR (High Frequency of Recombination) conjugation: HFR conjugation occurs when the donor bacterium has the F-plasmid integrated into its chromosome, resulting in a high frequency of transfer of chromosomal genes to the recipient bacterium during conjugation.
Why is it called conjugation?
The term “conjugation” is derived from the Latin word “conjugare,” which means “to join together.” It describes the joining or connection between the donor and recipient bacteria through a conjugation bridge during the process of genetic material transfer.
What is an example of bacterial conjugation?
An example of bacterial conjugation is the transfer of antibiotic resistance genes between bacteria. For instance, E. coli strains carrying antibiotic resistance plasmids can transfer these plasmids to other E. coli strains or different bacterial species through conjugation, leading to the spread of antibiotic resistance.
Why is conjugation important for bacteria?
Conjugation is important for bacteria as it enables the transfer of beneficial genetic traits, such as antibiotic resistance genes, virulence factors, and metabolic capabilities. It plays a significant role in bacterial adaptation, evolution, and the spread of genetic diversity among bacterial populations.
What bacteria use conjugation?
Conjugation is observed in various bacterial species. Examples of bacteria that use conjugation include Escherichia coli, Agrobacterium tumefaciens, Streptomyces species, Vibrio cholerae, and Bacillus subtilis, among others.
What is the principle of bacterial conjugation?
The principle of bacterial conjugation involves the transfer of genetic material from a donor bacterium to a recipient bacterium through a physical connection known as a conjugation bridge. This transfer can result in the acquisition of new genetic traits by the recipient bacterium.
What is the F and F conjugation?
F conjugation, also known as fertility or F-factor conjugation, is a type of bacterial conjugation where the donor bacterium contains a self-transmissible F-plasmid (F-factor). The F-plasmid carries genes required for the formation of the conjugation bridge and other conjugative functions.
What is HFR and F conjugation?
HFR (High Frequency of Recombination) conjugation is a type of conjugation in which the donor bacterium carries an F-plasmid integrated into its chromosome. During HFR conjugation, the transfer of genetic material includes not only the F-plasmid but also chromosomal genes adjacent to the integration site.
What is HFR and F prime conjugation?
HFR (High Frequency of Recombination) and F prime (F’) conjugation are used interchangeably. It refers to the process in which the donor bacterium carries an F-plasmid integrated into the chromosome and also carries additional chromosomal genes. The transferred genetic material includes the F-plasmid and the adjacent chromosomal genes.
Who is the father of conjugation in bacteria?
Joshua Lederberg, along with Edward Tatum, is often referred to as the father of conjugation in bacteria. They conducted groundbreaking experiments in the 1940s that demonstrated the transfer of genetic material between bacteria and laid the foundation for understanding bacterial conjugation.
Who discovered bacterial conjugation?
Bacterial conjugation was discovered by Joshua Lederberg and Edward Tatum in the late 1940s. Their experiments with Escherichia coli demonstrated the transfer of genetic material between bacterial cells, leading to the discovery of conjugation as a mechanism of horizontal gene transfer.
What is HFR conjugation in bacteria?
HFR (High Frequency of Recombination) conjugation is a type of bacterial conjugation where the donor bacterium carries an F-plasmid integrated into its chromosome. During conjugation, the transfer of genetic material includes the F-plasmid and adjacent chromosomal genes, resulting in a high frequency of recombination in the recipient bacterium.
- Frost, L.S., Leplae, R., Summers, A.O., and Toussaint, A. (2005). Mobile genetic elements: the agents of open source evolution. Nature Reviews Microbiology, 3(9), 722-732.
- Raleigh, E. A., & Low, K. B. (2013). Conjugation. Brenner’s Encyclopedia of Genetics, 144–151. doi:10.1016/b978-0-12-374984-0.00321-1
- Frost, L. S. (2009). Conjugation, Bacterial. Encyclopedia of Microbiology, 517–531. doi:10.1016/b978-012373944-5.00007-9
- Lawley, T.D., Klimke, W.A., Gubbins, M.J., and Frost, L.S. (2003). F factor conjugation is a true type IV secretion system. FEMS Microbiology Letters, 224(1), 1-15.
- Wilkins, B.M. (2002). Plasmid promiscuity: meeting the challenge of DNA immigration control. Journal of Bacteriology, 184(9), 2453-2461.
- Christie, P.J., and Cascales, E. (2005). Structural and dynamic properties of bacterial type IV secretion systems (Review). Molecular Membrane Biology, 22(1-2), 51-61.
- Waters, V.L. (2001). Conjugation between bacterial and mammalian cells. Nature Genetics, 29(4), 375-376.
- Guglielmini, J., Quintais, L., Garcillán-Barcia, M.P., de la Cruz, F., and Rocha, E.P. (2011). The repertoire of ICE in prokaryotes underscores the unity, diversity, and ubiquity of conjugation. PLoS Genetics, 7(8), e1002222.
- Lawley, T.D., and Kaper, J.B. (2005). Bacterial sexual reproduction and the evolution of virulence. Current Biology, 15(7), 568-574.
- Grohmann, E., Christie, P.J., Waksman, G., and Backert, S. (2018). Type IV secretion in Gram-negative and Gram-positive bacteria. Molecular Microbiology, 107(4), 455-471.
- https://www.biointeractive.org/classroom-resources/bacterial-conjugation
- https://biologydictionary.net/bacterial-conjugation/
- https://biologyreader.com/bacterial-conjugation.html