What is Allele?
- An allele is a distinct form of a gene, which plays a key role in genetic inheritance and variation. Genes, which are segments of DNA located on chromosomes, control the traits passed from one generation to the next. Each gene can exist in multiple forms, and these different forms are called alleles. They occupy specific locations, known as loci, on chromosomes.
- Every individual typically inherits two alleles for each gene—one from each parent. These alleles may either be the same or different. When both alleles are identical, the individual is said to be homozygous for that gene. On the other hand, if the alleles differ, the individual is heterozygous. The interaction between these alleles can determine the expression of certain traits.
- Alleles can be classified as dominant or recessive. A dominant allele is the one that is expressed in the organism, even if only one copy is present. In contrast, a recessive allele is only expressed when two copies are present, meaning that its effect is masked by the presence of a dominant allele. This interaction between dominant and recessive alleles helps define an organism’s phenotype, which is the observable expression of a trait.
- The combination of alleles an individual has for a particular gene is known as the genotype. Therefore, an individual’s genotype determines the potential phenotypic traits they may express. This distinction between genotype (the genetic makeup) and phenotype (the observable traits) is fundamental in understanding how genetic information is passed down and expressed in living organisms.
- Besides determining physical characteristics, alleles are also essential in explaining genetic diversity within populations. Allelic variation, often arising through mutations, contributes to the differences seen among individuals. This genetic diversity is the foundation of evolution and adaptation, as certain alleles may offer advantages in changing environments.
Definition of Allele
An allele is a specific variant of a gene that occupies a particular position (locus) on a chromosome, and different alleles can result in variations of a trait. Each individual inherits two alleles for each gene, one from each parent.
Features of Alleles
- Locus Specificity
- Alleles are located at specific positions on chromosomes called loci. Each allele occupies a fixed locus, which is vital for understanding gene organization within the genome and how traits are inherited.
- Two Alleles per Gene
- Individuals inherit two alleles for each gene, one from each parent. This dual inheritance contributes to genetic diversity and provides the basis for variation in traits within populations.
- Dominant and Recessive Interactions
- Alleles can either be dominant or recessive. A dominant allele expresses its associated trait even if only one copy is present, while a recessive allele is only expressed if two copies are inherited. These interactions determine an individual’s phenotype.
- Influence of Genetic and Environmental Factors
- The expression of alleles is not solely determined by genetic information. Environmental factors, as well as interactions with other genes, can influence how a particular allele manifests, leading to variations in phenotypic traits even among individuals with the same genotype.
- Wild-Type and Mutant Alleles
- Wild-type alleles represent the most common or standard form of a gene, typically associated with a “normal” trait expression. Mutant alleles arise from changes in the DNA sequence and can produce variations in traits. These mutations can be beneficial, neutral, or harmful, depending on how they affect an organism.
- Neutral Alleles
- Neutral alleles are those that do not affect an organism’s phenotype. These alleles do not influence observable traits or confer any selective advantage or disadvantage.
- Contribution to Evolution and Adaptation
- Allelic variation provides the genetic flexibility necessary for populations to adapt to changing environments. Through processes like natural selection, certain alleles may become more common in a population, leading to evolutionary change over time.
- Homozygous vs. Heterozygous Genotypes
- When both inherited alleles for a gene are identical, the individual is homozygous for that trait. If the alleles differ, the individual is heterozygous. These genotypic differences play a key role in determining trait expression.
Types of Alleles
There are different types of Alleles such as;
- Wild-Type Alleles
- The wild-type allele is the most common form of a gene found in natural populations. It represents the standard or “normal” version of a gene that typically encodes the standard expression of a trait. This allele is often considered the baseline for comparing other allele variations.
- Mutant Alleles
- Mutant alleles result from changes or mutations in the DNA sequence of a gene. These mutations can alter the gene’s function, producing variations in the associated trait. Mutant alleles can be either dominant or recessive, depending on how they interact with the corresponding allele in the pair. Dominant mutant alleles will express the altered trait even if only one copy is present, while recessive mutant alleles require two copies for the trait to be expressed.
- Dominant Alleles
- A dominant allele expresses its trait even when only one copy is present in the genotype. This means that in a heterozygous state (where one dominant and one recessive allele are present), the dominant allele will determine the trait. This is a key factor in determining which traits become visible in offspring.
- Recessive Alleles
- Recessive alleles only express their traits when two copies are inherited, one from each parent. In the presence of a dominant allele, the trait associated with the recessive allele remains hidden. Recessive alleles often contribute to genetic diversity, as they can be passed down through generations without being expressed.
- Neutral Alleles
- Neutral alleles have no observable impact on an organism’s phenotype. They do not affect any traits or characteristics, as they do not alter the gene’s function in a way that influences physical or biochemical traits. Neutral alleles often arise through mutations that neither benefit nor harm the organism.
- Lethal Alleles
- Lethal alleles are mutations that can lead to the death of an organism, often before birth or early in life. These alleles may affect essential genes that are crucial for survival. Lethal alleles are typically recessive, meaning that two copies are required for the lethal effect to manifest.
- Co-dominant Alleles
- Co-dominant alleles result in both alleles being expressed simultaneously in the heterozygous state. Unlike dominant-recessive interactions, where one allele masks the other, co-dominant alleles allow for both traits to be visible. An example of this is blood type, where both A and B alleles are expressed in individuals with type AB blood.
- Incomplete Dominant Alleles
- Incomplete dominance occurs when neither allele is fully dominant, resulting in a blend of traits in the heterozygous state. The phenotype is an intermediate expression, combining characteristics of both alleles. This type of interaction illustrates a gradient of trait expression rather than a strict dominant-recessive relationship.
What is Complete Dominance?
Complete dominance is a fundamental concept in genetics where one allele completely masks the expression of another allele. This type of inheritance is well exemplified by the flower color in garden peas, as studied by Gregor Mendel, which helped establish foundational principles of genetic inheritance.
Key Concepts
- Alleles and Traits:
- Gene for Flower Color: The flower color in garden peas is determined by a specific gene that exists in two allelic forms: one for purple color (P) and one for white color (p).
- Allele Functions:
- Purple Allele (P): Encodes for a pigment that produces purple flowers.
- White Allele (p): Does not produce the pigment, resulting in white flowers.
- Genotype and Phenotype Relationships:
- Genotypes:
- PP: Homozygous dominant genotype that results in purple flowers.
- Pp: Heterozygous genotype that also results in purple flowers due to the presence of at least one dominant P allele.
- pp: Homozygous recessive genotype that results in white flowers as there is no dominant allele to produce the purple pigment.
- Phenotypes:
- Purple Flowers: Expressed in both PP and Pp genotypes.
- White Flowers: Expressed only in the pp genotype.
- Genotypes:
- Dominant and Recessive Alleles:
- Dominant Allele: In this context, the purple allele (P) is dominant. It can mask the effect of the recessive allele when at least one copy is present.
- Recessive Allele: The white allele (p) is recessive and only manifests its trait when two copies are present (pp).
- Illustrative Example:
- Punnett Square Analysis: To visualize how these alleles combine, a Punnett square can be used to predict the genotypic and phenotypic ratios in the offspring of pea plants.
- For example, a cross between two heterozygous (Pp) pea plants results in a 3:1 ratio of purple to white flowers in the offspring. This ratio reflects the complete dominance of the purple allele.
- Punnett Square Analysis: To visualize how these alleles combine, a Punnett square can be used to predict the genotypic and phenotypic ratios in the offspring of pea plants.
Implications and Extensions
- Understanding Dominance:
- Phenotypic Expression: The dominance relationship means that the phenotype associated with the dominant allele will appear if at least one dominant allele is present.
- Recessive Expression: The recessive phenotype only appears when an individual is homozygous recessive.
- Contrast with Other Inheritance Patterns:
- Codominance and Incomplete Dominance: Unlike complete dominance, codominance involves both alleles contributing equally to the phenotype, while incomplete dominance results in a blending of traits. For example, ABO blood group inheritance exhibits codominance, where both A and B alleles are expressed in the AB blood type.
What is Incomplete Dominance?
Incomplete dominance is a genetic phenomenon where neither allele in a gene pair is fully dominant over the other. This results in a distinct phenotype in heterozygous individuals that is intermediate between the phenotypes of the two homozygous forms. Below is a detailed explanation of incomplete dominance and its implications.
Key Concepts
- Definition and Characteristics:
- Intermediate Phenotype: Incomplete dominance occurs when the heterozygous genotype produces a phenotype that is a blend or intermediate between the phenotypes of the homozygous dominant and homozygous recessive forms.
- Allelic Interaction: Neither allele masks the effect of the other. Both alleles contribute to the phenotype, leading to a mixed or blended appearance.
- Examples of Incomplete Dominance:
- Flower Color in Snapdragons:
- Alleles: The gene for flower color in snapdragons has two alleles: one for red color (R) and one for white color (r).
- Phenotypic Outcomes:
- RR (Homozygous Dominant): Red flowers.
- rr (Homozygous Recessive): White flowers.
- Rr (Heterozygous): Pink flowers. The pink color is a blend of the red and white colors, demonstrating incomplete dominance.
- Coat Color in Cattle:
- Alleles: The coat color in some cattle breeds shows incomplete dominance between the red and white color alleles.
- Phenotypic Outcomes:
- RR (Homozygous Dominant): Red coat.
- WW (Homozygous Recessive): White coat.
- RW (Heterozygous): Roan coat, which is a mix of red and white hairs.
- Flower Color in Snapdragons:
- Genotypic and Phenotypic Ratios:
- Punnett Square Analysis: A Punnett square can be used to predict the phenotypic ratios in offspring from a cross involving incomplete dominance.
- For a cross between two heterozygous individuals (Rr x Rr) in snapdragons:
- Genotype Ratios: 1 RR : 2 Rr : 1 rr.
- Phenotype Ratios: 1 Red : 2 Pink : 1 White.
- For a cross between two heterozygous individuals (Rr x Rr) in snapdragons:
- Punnett Square Analysis: A Punnett square can be used to predict the phenotypic ratios in offspring from a cross involving incomplete dominance.
- Mechanism of Incomplete Dominance:
- Gene Expression: Incomplete dominance occurs due to the presence of different alleles coding for enzymes or proteins that contribute to pigment or other traits. The intermediate phenotype results from the partial activity of the enzymes or proteins produced by the alleles.
- Gene Dosage Effect: The amount of gene product affects the phenotype, and the heterozygous state produces a gene product amount intermediate between the two homozygous states.
- Comparison with Other Inheritance Patterns:
- Codominance: Unlike incomplete dominance, codominance involves both alleles being fully expressed simultaneously without blending. An example is the ABO blood group system, where both A and B alleles are expressed in the AB blood type.
- Complete Dominance: In complete dominance, one allele completely masks the effect of the other, resulting in a phenotype that resembles the dominant allele’s effect, as seen in Mendelian traits like pea flower color.
What are Dominant and Recessive Alleles?
Dominant and recessive alleles are fundamental concepts in Mendelian genetics that describe how different forms of a gene affect an organism’s phenotype. Understanding these concepts is crucial for interpreting patterns of inheritance and genetic expression.
Dominant Alleles
- Definition and Function:
- Dominant Allele: A dominant allele is an allele that expresses its trait in the phenotype, even when only one copy is present. It masks the effect of a recessive allele when both are present.
- Expression: The dominant allele can be present in either one or two copies in an organism’s genotype and will be expressed in the phenotype. For instance, in the case of a trait for widow’s peak hairline, the W allele is dominant.
- Genotypic Representation:
- Homozygous Dominant (WW): An individual with two copies of the dominant allele. This genotype results in the dominant trait being expressed.
- Heterozygous (Ww): An individual with one dominant allele and one recessive allele. The dominant allele will still express the trait, overriding the recessive allele.
- Phenotypic Outcome:
- Example: In the widow’s peak trait, an individual with at least one W allele (either WW or Ww) will exhibit a widow’s peak, as the W allele is dominant over the w allele.
Recessive Alleles
- Definition and Function:
- Recessive Allele: A recessive allele is an allele whose trait is only expressed in the phenotype when two copies are present. It is masked by the presence of a dominant allele.
- Expression: The recessive allele must be homozygous (i.e., two copies of the recessive allele) for its trait to be visible in the phenotype. For example, the w allele for a straight hairline is recessive.
- Genotypic Representation:
- Homozygous Recessive (ww): An individual with two copies of the recessive allele. This genotype results in the recessive trait being expressed because there is no dominant allele to mask it.
- Phenotypic Outcome:
- Example: In the widow’s peak trait, only individuals with the genotype ww will have a straight hairline, as the dominant W allele is not present to overshadow the effect of the recessive w allele.
Interaction Between Dominant and Recessive Alleles
- Phenotypic Expression:
- Dominant Over Recessive: When a dominant allele is present, it determines the phenotype regardless of whether the second allele is dominant or recessive. This means that a heterozygous individual (Ww) will display the dominant trait associated with the W allele.
- Recessive Trait Expression: A recessive trait is only visible when both alleles are recessive. Therefore, for an individual to display a recessive trait, they must have two copies of the recessive allele (ww).
- Mendelian Inheritance Patterns:
- Genotype to Phenotype Mapping: The principles of dominance and recessiveness allow for the prediction of phenotypic ratios in offspring based on the genotypes of the parents. For example, a cross between two heterozygous individuals (Ww x Ww) results in a 3:1 ratio of dominant to recessive phenotypes in their offspring.
What is Codominance?
Codominance is a pattern of inheritance where both alleles in a heterozygous genotype contribute equally and visibly to the organism’s phenotype. Unlike complete dominance, where one allele overshadows the other, codominance results in both traits being expressed simultaneously.
Key Concepts
- Definition and Characteristics:
- Codominant Alleles: Codominance occurs when two different alleles for a gene are both expressed in the phenotype of a heterozygous individual. Neither allele masks the effect of the other.
- Phenotypic Expression: In codominance, the traits associated with both alleles are observable, rather than blending together as in incomplete dominance.
- Example of Codominance:
- ABO Blood Group System:
- Alleles Involved: The ABO blood group gene has three alleles: A, B, and O.
- Codominance: Both A and B alleles are codominant with each other. This means that individuals with one A allele and one B allele will have both A and B antigens on the surface of their red blood cells.
- Recessive Allele: The O allele is recessive and does not affect the expression of A or B antigens.
- ABO Blood Group System:
- Genotypic and Phenotypic Outcomes:
- Genotype and Phenotype Relationships:
- AA or AO Genotype: The phenotype will display A antigens on the red blood cells.
- BB or BO Genotype: The phenotype will display B antigens on the red blood cells.
- AB Genotype: The phenotype will display both A and B antigens, demonstrating codominance.
- OO Genotype: The phenotype will have no A or B antigens, resulting in blood type O.
- Punnett Square Analysis: When performing genetic crosses involving codominance, a Punnett square can help predict the ratios of different blood types among offspring.
- Genotype and Phenotype Relationships:
- Mechanism of Codominance:
- Gene Expression: Codominance arises because both alleles contribute to the final protein product without interfering with each other. In the case of blood type, the A and B alleles code for different antigens that are both incorporated into the cell membrane.
- Antigen Presentation: In individuals with an AB genotype, both antigens (A and B) are present on the red blood cells’ surface, leading to a distinct blood type that shows both characteristics.
- Comparison with Other Inheritance Patterns:
- Complete Dominance: In complete dominance, the presence of one dominant allele masks the effect of the recessive allele, resulting in a phenotype that only reflects the dominant trait.
- Incomplete Dominance: In incomplete dominance, the heterozygous phenotype is a blend of the two homozygous phenotypes, rather than showing both traits distinctly.
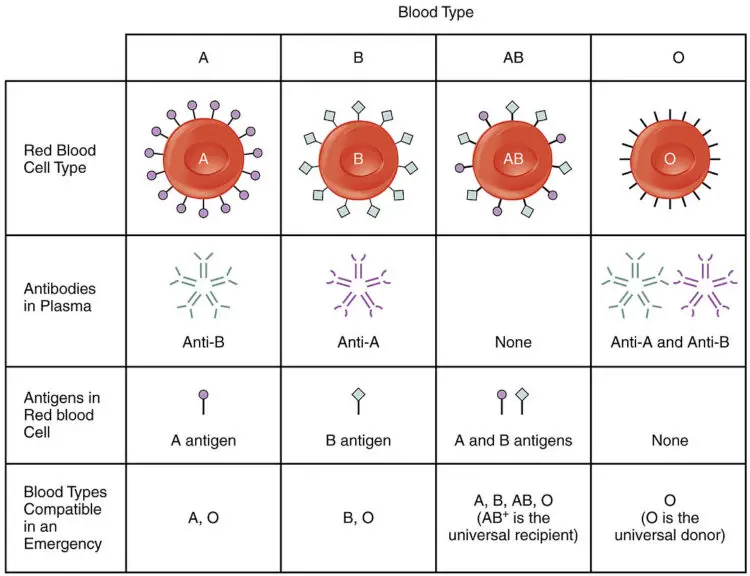
What is Multiple Alleles?
Multiple alleles refer to a genetic scenario where more than two alternative forms of a gene are present within a population. This concept extends the traditional Mendelian inheritance pattern, where typically only two alleles (dominant and recessive) are considered. Instead, multiple alleles contribute to genetic diversity and can affect the phenotype in a more complex manner.
Key Concepts
- Definition and Characteristics:
- Multiple Alleles: A gene with more than two allelic forms. Although an individual can carry a maximum of two alleles for a given gene, the gene pool of a population may include several different alleles.
- Phenotypic Variation: The presence of multiple alleles increases the potential for genetic variation within a population, leading to a broader range of possible phenotypes.
- Example of Multiple Alleles:
- Coat Color in Rabbits:
- Alleles Involved: The gene responsible for coat color in rabbits has four distinct alleles:
- C (full color)
- Cch (chinchilla)
- Chd (Himalayan)
- c (albino)
- Phenotypic Expression: Each allele results in a different coat color or pattern:
- C produces a full-color coat.
- Cch results in a chinchilla coat, which is a diluted color.
- Chd creates a Himalayan coat with coloration on extremities (ears, paws, and tail) and a lighter body color.
- c leads to an albino coat, with no pigmentation.
- Alleles Involved: The gene responsible for coat color in rabbits has four distinct alleles:
- Coat Color in Rabbits:
- Genotypic and Phenotypic Outcomes:
- Genotypic Combinations: An individual rabbit inherits two alleles for the coat color gene, one from each parent. The combination of these alleles determines the rabbit’s coat color.
- CC: Full color.
- CCh: Chinchilla (Cch is dominant over Chd and c but recessive to C).
- ChdChd: Himalayan (Chd is dominant over c but recessive to C and Cch).
- cc: Albino (c is recessive and expressed only when both alleles are c).
- Genotypic Combinations: An individual rabbit inherits two alleles for the coat color gene, one from each parent. The combination of these alleles determines the rabbit’s coat color.
- Mechanism and Impact:
- Allelic Interactions: In cases with multiple alleles, the dominance hierarchy among the alleles determines the phenotype. For example, in the rabbit coat color gene, the full-color allele (C) is dominant over the other alleles, which are expressed in different patterns of dominance.
- Genetic Diversity: Multiple alleles contribute to genetic diversity within a population, influencing the range of phenotypic traits and enabling adaptive variations.
- Comparison with Other Genetic Patterns:
- Simple Dominance/Recessiveness: Unlike simple dominant-recessive inheritance, where only two alleles are considered, multiple alleles introduce additional layers of complexity in trait expression.
- Codominance and Incomplete Dominance: Multiple alleles can exhibit various inheritance patterns, including codominance (where both alleles are expressed equally) or incomplete dominance (where the heterozygous phenotype is a blend of the two homozygous phenotypes).
Genetic Diseases by Alleles
Genetic diseases arise due to mutations in specific alleles of genes, leading to various health issues. These diseases can follow Mendelian inheritance patterns or involve more complex genetic interactions. Here, we examine how specific genetic diseases are associated with mutations in alleles.
1. Sickle Cell Anemia
- Cause: Sickle cell anemia results from a mutation in the HBB gene, which encodes the beta-globin subunit of hemoglobin.
- Mutation Details: The mutation leads to the substitution of adenine with thymine in the DNA sequence, causing a single amino acid change in the beta-globin protein (glutamic acid to valine). This alteration results in the production of abnormal hemoglobin (HbS).
- Phenotypic Effects: The presence of HbS causes red blood cells to assume a rigid, sickle-like shape. These misshapen cells can obstruct blood flow in capillaries, leading to pain, tissue damage, increased risk of infection, and organ damage.
- Inheritance Pattern: Sickle cell anemia is inherited in an autosomal recessive manner. Individuals must inherit two copies of the mutated allele (one from each parent) to express the disease phenotype.
2. Cystic Fibrosis
- Cause: Cystic fibrosis is caused by mutations in the CFTR gene, which codes for the cystic fibrosis transmembrane conductance regulator protein.
- Mutation Details: The most common mutation is a deletion of three nucleotides that results in the loss of a phenylalanine residue at position 508 of the CFTR protein (ΔF508). This mutation impairs the protein’s function as a chloride channel.
- Phenotypic Effects: The defective CFTR protein leads to the production of thick, sticky mucus that accumulates in the lungs, pancreas, and other organs. This mucus obstructs airways and ducts, causing respiratory infections, digestive problems, and reduced life expectancy.
- Inheritance Pattern: Cystic fibrosis follows an autosomal recessive inheritance pattern. Both alleles of the CFTR gene must carry the mutation for the disease to manifest.
3. Huntington’s Disease
- Cause: Huntington’s disease is caused by a mutation in the HTT gene, which encodes the protein huntingtin.
- Mutation Details: The mutation involves an expansion of CAG triplet repeats in the HTT gene. Normal HTT contains 10-35 repeats of the CAG sequence, while mutated versions have 36 or more repeats. This expansion leads to the production of an abnormal huntingtin protein.
- Phenotypic Effects: The mutant huntingtin protein causes progressive neurodegeneration, particularly in the basal ganglia and cerebral cortex. This results in motor dysfunction, cognitive decline, and psychiatric symptoms.
- Inheritance Pattern: Huntington’s disease is inherited in an autosomal dominant manner. A single copy of the mutated allele is sufficient to cause the disease, and affected individuals have a 50% chance of passing the mutation to their offspring.
Allelic Variation
Allelic variation is a fundamental concept in genetics that explains how genetic diversity arises through differences in alleles, the alternative forms of a gene. This variation is crucial for understanding genetic traits and their expressions in different organisms.
Types of Genetic Variation
- Discontinuous Variation:
- Definition: This type of variation results in distinct and separate phenotypic categories. Traits that show discontinuous variation are easily classified into discrete categories, such as blood groups or flower colors.
- Examples:
- Blood Groups: The ABO blood group system is an example where allelic differences result in distinct blood types (A, B, AB, O).
- Flower Color: In some plants, flower color can be either red or white with no intermediate hues.
- Polymorphism and Morphs:
- Polymorphism: Refers to the presence of two or more common phenotypes in a population.
- Morphs: Specific phenotypes within a polymorphism.
- Mutants: Rare phenotypes that deviate from the common types, with the normal phenotype termed as the wild-type.
- Continuous Variation:
- Definition: This variation results in a range of phenotypes that cannot be distinctly categorized. Traits exhibit a continuum of forms, such as height or weight.
- Examples:
- Height and Weight: These traits show a continuous range due to their polygenic nature.
- Eye Color: Shows a spectrum of colors rather than discrete categories.
- Genetic Complexity: Traits with continuous variation are usually influenced by multiple genes and their interactions, making them more complex to analyze compared to discontinuous traits.
Molecular Basis of Allelic Variation
- Genetic Variation and Protein Function:
- Proteins as Phenotypic Factors: Many phenotypic traits are expressed through proteins, which are products of gene expression. These proteins can include enzymes, pigments, and structural components.
- Impact of Genetic Changes:
- Nucleotide Substitution: This involves a change in a single nucleotide and can lead to amino acid substitutions. The effect of such substitutions depends on their location within the protein’s structure.
- Frameshift Mutations: These occur from the addition or deletion of nucleotides in numbers that are not multiples of three, leading to shifts in the reading frame of the mRNA. This often results in significant changes to the protein structure and function.
- Effects on Protein Function:
- Amino Acid Substitution: If the substituted amino acid is critical for the protein’s function, such as in the active site of an enzyme, it can disrupt the enzyme’s activity.
- Stop Codons: Mutations can introduce premature stop codons, leading to truncated proteins that are nonfunctional.
- Frameshift Mutations: These mutations alter the entire downstream amino acid sequence, often resulting in nonfunctional proteins due to drastic changes in the protein’s structure.
Examples of Alleles
The following are some illustrative examples of how different alleles influence various phenotypic characteristics:
- Eye Color
- Blue, Hazel, Brown, and Green Eyes: Eye color is determined by multiple alleles interacting. For example, the presence of different alleles influences the production and distribution of pigments in the iris. Each allele contributes to the specific shade of eye color.
- Hair Color
- Black and Blonde Hair: Hair color is controlled by alleles affecting melanin production. Variants of the MC1R gene are known to influence whether an individual has black, blonde, or other hair colors.
- Skin Color
- Dark and Fair Skin: Skin pigmentation results from the interaction of multiple alleles that control melanin production. These alleles influence the overall skin tone, ranging from dark to fair.
- Blood Type
- AB and O Blood Types: Blood type is determined by alleles of the ABO blood group system. The A and B alleles produce A and B antigens, respectively, while the O allele lacks these antigens. The combination of these alleles determines the blood type: A, B, AB, or O.
- Flower Color in Peas
- Purple and White Flowers: In pea plants, the color of the flowers is governed by alleles of the gene responsible for pigment production. The presence of different alleles leads to either purple or white flowers.
- Height
- Human Height: Height is a polygenic trait influenced by multiple alleles. These alleles interact in complex ways to determine the final stature of an individual.
Importance of Alleles
Alleles play a crucial role in genetics and the expression of traits. Their importance can be understood through several key aspects:
- Genetic Diversity
- Variation: Alleles contribute to genetic variation within a population. This variation is essential for evolution and adaptation as it provides a pool of genetic resources that can be selected for or against in changing environments.
- Unique Combinations: The different combinations of alleles inherited from both parents result in unique genotypes and phenotypes for each individual, contributing to the diversity observed in populations.
- Trait Expression
- Phenotypic Outcomes: Alleles influence the expression of traits, from physical characteristics such as eye color and hair type to physiological traits like blood type and susceptibility to diseases. The interaction between dominant and recessive alleles determines which traits are expressed.
- Homozygosity and Heterozygosity: The presence of two identical alleles (homozygous) or two different alleles (heterozygous) affects trait expression. For instance, a heterozygous individual for a trait may express the dominant phenotype, while a homozygous recessive individual will express the recessive phenotype.
- Genetic Inheritance
- Mendelian Patterns: Alleles follow Mendelian inheritance patterns, which describe how traits are passed from parents to offspring. Understanding these patterns is crucial for predicting genetic outcomes and studying hereditary conditions.
- Punnett Squares: Tools such as Punnett squares use allele combinations to predict the probability of offspring inheriting certain traits. This method is fundamental in genetics for studying inheritance patterns.
- Disease and Health
- Genetic Disorders: Certain alleles are associated with genetic disorders. For example, alleles of the CFTR gene are linked to cystic fibrosis. Identifying and understanding these alleles can aid in diagnosis, treatment, and genetic counseling.
- Personalized Medicine: Knowledge of specific alleles can inform personalized medicine approaches, where treatments and preventive measures are tailored based on an individual’s genetic profile.
- Evolutionary Significance
- Adaptation and Survival: Allelic variation can influence an organism’s ability to adapt to environmental changes. Beneficial alleles that enhance survival and reproduction are more likely to be passed on to future generations, driving evolutionary processes.
- Natural Selection: Alleles that confer advantageous traits can be selected for through natural selection, leading to the gradual evolution of species.
- https://www.biologyonline.com/dictionary/allele#Allelic_Variation
- https://www.geeksforgeeks.org/what-is-an-allele/
- https://collegedunia.com/exams/allele-biology-articleid-1237
- https://www.javatpoint.com/allele-definition
- https://socratic.org/questions/what-is-alil
- https://sphweb.bumc.bu.edu/otlt/MPH-Modules/PH/DNA-Genetics/DNA-Genetics5.html
- https://sciencenotes.org/allele-definition-and-examples/
- https://www.thoughtco.com/allele-a-genetics-definition-373460
- https://courses.lumenlearning.com/wm-biology1/chapter/reading-multiple-alleles/