What is Vitamin B1 (Thiamine)?
- Vitamin B1, commonly known as Thiamine, is a water-soluble vitamin that plays a pivotal role in the metabolism of glucose, proteins, and lipids in the human body. It is an essential micronutrient, meaning that it cannot be synthesized by the body and must be obtained through dietary sources or supplements.
- Structurally, Thiamine is composed of a thiazole ring and an aminopyrimidine ring, connected by a methylene bridge. The thiazole ring is substituted with methyl and hydroxyethyl side chains. In its active form, Thiamine is known as thiamine pyrophosphate (TPP) or thiamine diphosphate (ThDP), which acts as a coenzyme in various metabolic reactions. Besides ThDP, other natural derivatives of Thiamine include thiamine monophosphate (ThMP), thiamine triphosphate (ThTP), adenosine thiamine triphosphate (AThTP), and adenosine thiamine diphosphate (AThDP).
- Dietary sources rich in Thiamine include whole grains, legumes, certain meats, and fish. However, the processing of grains can lead to a significant loss of Thiamine content. Therefore, many countries have adopted the practice of enriching cereals and flours with this essential vitamin.
- The body absorbs Thiamine through the small intestine, where it is hydrolyzed into its free form by the enzyme phosphatase. Once absorbed, it is stored in various organs, including the heart, kidneys, liver, and brain. Given its short half-life of 14 to 18 days, a regular intake of Thiamine is crucial to prevent deficiency.
- Deficiency of Thiamine can lead to several health complications. Early symptoms of deficiency encompass neurological, cardiac, and gastrointestinal manifestations. A severe form of Thiamine deficiency results in a condition called beriberi, which can be categorized as wet or dry. Wet beriberi is characterized by cardiac symptoms, while dry beriberi primarily affects the nervous system. Prolonged deficiency can escalate to Wernicke-Korsakoff syndrome, a severe neurological disorder commonly associated with chronic alcohol consumption.
- In conclusion, Thiamine is an indispensable vitamin that plays a vital role in various metabolic processes. It is crucial to ensure adequate intake of this vitamin to maintain optimal health and prevent potential deficiencies and associated disorders. Therefore, understanding its functions, sources, and the consequences of its deficiency is essential for overall well-being.
Definition of Vitamin B1 (Thiamine)
Vitamin B1, also known as thiamine, is a water-soluble vitamin essential for the metabolism of glucose and amino acids in the body. It plays a critical role in energy production and is vital for the proper function of the nervous system. Thiamine is naturally found in foods such as whole grains, legumes, and some meats and fish, and is also available as a dietary supplement. Deficiency in thiamine can lead to disorders like beriberi and Wernicke encephalopathy.
Mechanism of Action
- Absorption and Circulation: Once ingested, thiamin is absorbed from the gastrointestinal tract into the bloodstream. From here, it circulates throughout the body, reaching various tissues and organs. Excess thiamin that the body doesn’t utilize is excreted through the urine. It’s noteworthy that while the liver, heart, kidney, and brain can store small amounts of thiamin, these reserves are limited and last only for a short duration.
- Activation: In the blood, an enzyme called thiamin diphosphokinase facilitates the conversion of thiamin into its active form, thiamin pyrophosphate (TPP).
- Role in Metabolism: TPP is a crucial coenzyme in several metabolic pathways:
- Carbohydrate Metabolism: TPP acts as a cofactor in glycolysis and the oxidative decarboxylation of carbohydrates. Therefore, it aids in the breakdown of sugars and the production of energy.
- Krebs Cycle: TPP is essential for mitochondrial enzyme complexes like α-ketoglutarate dehydrogenase and pyruvate dehydrogenase. These enzymes are integral to the Krebs cycle (or tricarboxylic acid cycle), a central metabolic pathway responsible for energy production. A deficiency in thiamin can impair these enzymes, leading to issues like the accumulation of lactic acid. This buildup can cause damage to specific brain structures, evident in MRI scans.
- Lipid and Amino Acid Metabolism: TPP is involved in the metabolism of lipids and branched-chain amino acids, ensuring the proper breakdown and utilization of fats and proteins.
- Pentose Phosphate Pathway: TPP is required by the erythrocyte transketolase enzyme during the pentose phosphate pathway. This pathway is vital for nucleotide synthesis and provides reduced nicotinamide adenine dinucleotide phosphate (NADPH) for various synthetic processes.
Chemistry of Vitamin B1 (Thiamine)
- Structural Components: At the core of thiamine’s structure are two distinct rings: a pyrimidine ring and a thiazole ring. These two rings are interconnected by a methylene bridge, as depicted in Fig.7.17. Notably, thiamine stands out as the sole natural compound that incorporates a thiazole ring in its structure.
- Formation of Thiamine Pyrophosphate (TPP): The alcohol (OH) group present in thiamine undergoes esterification with phosphate, specifically binding with two moles of phosphate. This process results in the formation of thiamine pyrophosphate (TPP), also referred to as cocarboxylase. TPP is not just a derivative of thiamine but serves as its active coenzyme form, crucial for various enzymatic reactions in the body.
- Role of ATP: The pyrophosphate group in TPP is derived from adenosine triphosphate (ATP). ATP donates the pyrophosphate moiety, facilitating the conversion of thiamine to TPP.
- Enzymatic Catalysis: The transformation of thiamine to TPP is not a spontaneous event. It requires the action of a specific enzyme known as thiamine pyrophosphate transferase. This enzyme catalyzes the reaction, ensuring the efficient and accurate formation of TPP from thiamine.
Biochemical functions
- Carbohydrate Metabolism and Energy Release: TPP is intricately linked with reactions that release energy from carbohydrates. This connection emphasizes the importance of TPP in energy production and utilization within the body.
- Role in Pyruvate Conversion: The enzyme pyruvate dehydrogenase is responsible for the oxidative decarboxylation of pyruvate, converting it irreversibly to acetyl CoA. This reaction is not only dependent on TPP but also on other coenzymes. The specifics of this process are further elaborated in the section on carbohydrate metabolism in Chapter 13.
- Involvement in the Citric Acid Cycle: The enzyme α-Ketoglutarate dehydrogenase, a component of the citric acid cycle, shares similarities with pyruvate dehydrogenase. This enzyme, too, necessitates the presence of TPP for its proper functioning.
- Hexose Monophosphate Shunt (HMP Shunt): TPP is essential for the enzyme transketolase, which operates within the hexose monophosphate shunt (HMP shunt). This pathway plays a role in pentose sugar metabolism and the generation of NADPH.
- Decarboxylation of Branched Chain Amino Acids: The enzyme branched chain α-keto acid dehydrogenase (decarboxylase) oversees the oxidative decarboxylation of branched chain amino acids such as valine, leucine, and isoleucine. This conversion results in the formation of respective keto acids. Significantly, this enzyme also mandates the presence of TPP.
- Neural Functions: Beyond metabolic reactions, TPP holds significance in the realm of neural activity. It is postulated that TPP is essential for the synthesis of acetylcholine, a neurotransmitter, and for the ion translocation processes within neural tissues.
Recommended dietary allowance (RDA)
- General Requirement: The daily need for thiamine is closely tied to carbohydrate intake. For adults, a dietary provision of 1-1.5 mg/day is advised, which translates to approximately 0.5 mg for every 1,000 Calories of energy consumed. For children, the RDA ranges between 0.7 to 1.2 mg/day. Specific conditions such as pregnancy, lactation, old age, and alcoholism may lead to a slight increase in thiamine requirements, with a recommendation of up to 2 mg/day.
- US National Academy of Medicine Guidelines: In 1998, the US National Academy of Medicine revised the Estimated Average Requirements (EARs) and RDAs for thiamine. For women and men aged 14 and above, the EARs are set at 0.9 mg/day and 1.1 mg/day, respectively. The corresponding RDAs are 1.1 mg/day for women and 1.2 mg/day for men. It’s noteworthy that RDAs are set higher than EARs to cater to individuals with above-average requirements. During pregnancy and lactation, the RDA is pegged at 1.4 mg/day. For infants up to 12 months, the Adequate Intake (AI) ranges from 0.2 to 0.3 mg/day. As children age from 1 to 13 years, the RDA progressively increases from 0.5 to 0.9 mg/day.
- European Food Safety Authority (EFSA) Guidelines: The EFSA employs different terminologies, referring to the set of dietary recommendations as Dietary Reference Values. They use Population Reference Intakes (PRIs) in place of RDAs and Average Requirements instead of EARs. For all individuals, including women (whether pregnant or lactating), men, and children, the PRI is set at 0.1 mg of thiamine per megajoule (MJ) of dietary energy. Given the conversion rate of 1 MJ being equivalent to 239 kcal, an adult consuming 2390 kilocalories should ideally intake 1.0 mg of thiamine. This recommendation is marginally lower than the US RDA.
- Upper Intake Level: Neither the National Academy of Medicine nor the EFSA has established an upper intake threshold for thiamine, given the absence of data indicating adverse effects from high doses.
- Safety Considerations: When taken orally, thiamine is generally well-tolerated and poses no toxicity risks. However, there have been infrequent reports of side effects when thiamine is administered intravenously, which include allergic reactions, nausea, lethargy, and coordination issues.
- Labeling Standards: In the US, for food and dietary supplement labeling purposes, the thiamine content in a serving is expressed as a percentage of the Daily Value. Since May 27, 2016, this Daily Value has been standardized at 1.2 mg, aligning with the RDA.
Dietary Sources of Thiamine
- Plant-Based Sources: Cereals, pulses, oil seeds, and nuts are rich in thiamine. Among these, cereals are particularly noteworthy. The outer layer of cereals, known as the bran, is where thiamine is predominantly concentrated. However, it’s essential to note that the process of polishing rice can lead to a significant reduction in its thiamine content, with about 80% of the vitamin being removed.
- Animal-Based Sources: Besides plant-based foods, thiamine is also found in various animal products. Pork, liver, heart, and kidney are notable sources. Additionally, milk is another animal-derived beverage that contains thiamine.
- Parboiled Rice: An interesting aspect of rice processing is the method of parboiling, which involves boiling paddy with its husk. When rice undergoes this process and is subsequently milled, thiamine is retained, ensuring that the nutrient is not lost during polishing.
- Water Solubility and Cooking: Thiamine’s water-soluble nature implies that it can be extracted into the water during the cooking process. Therefore, it’s crucial to be cautious while cooking foods rich in thiamine. If these foods are boiled or cooked in water, the vitamin leaches into the water. To maximize the retention of thiamine, it’s advisable not to discard the water used for cooking.
Deficiency Symptoms of Vitamin B1 (Thiamine)
- Beri-Beri: A significant deficiency of Vitamin B1 can result in a condition termed ‘beri-beri.’ The name ‘beri-beri’ is derived from the Sinhalese language, translating to “I cannot” repeated twice. This condition is predominantly observed in populations that primarily consume polished rice as their staple food. The polishing process removes the outer layer of the rice grain, which is rich in thiamine, leading to a reduced intake of the vitamin.
- Early Indications: The initial symptoms of thiamine deficiency can be subtle but progressively worsen if not addressed. These include:
- Loss of Appetite (Anorexia): Individuals may experience a decreased desire to eat.
- Weakness: A general feeling of fatigue and lack of energy.
- Digestive Issues: Constipation and nausea are common digestive problems.
- Mental Health Effects: Symptoms such as mental depression and irritability can manifest.
- Peripheral Neuropathy: This refers to disorders that occur when nerves outside the brain and spinal cord are damaged. It can lead to numbness and a tingling sensation in the extremities.
- Neurological Symptoms: As the deficiency progresses, individuals may report feelings akin to ‘pins and needles sensations,’ especially in the legs. This sensation is indicative of nerve damage or neuropathy, which can be a direct consequence of prolonged thiamine deficiency.
Synthesis of B1
Its biosynthesis is a complex process that occurs in specific organisms, including bacteria, some protozoans, plants, and fungi. This expository article aims to provide a detailed and sequential explanation of the synthesis of thiamine, emphasizing its functions and the technical vocabulary associated with its production.
- Biosynthesis of Thiamine:
- Thiazole and Pyrimidine Moieties: Thiamine biosynthesis begins with the separate formation of thiazole and pyrimidine moieties. These moieties are then combined to produce Thiamine Monophosphate (ThMP) through the action of thiamine-phosphate synthase.
- Formation of Pyrimidine Ring: The pyrimidine ring system is synthesized in a reaction catalyzed by phosphomethylpyrimidine synthase, an enzyme belonging to the radical SAM superfamily of iron–sulfur proteins. This enzyme utilizes S-adenosyl methionine as a cofactor. The starting material for this process is 5-aminoimidazole ribotide, which undergoes a rearrangement reaction to form the product.
- Formation of Thiazole Ring: The thiazole ring is synthesized in a reaction catalyzed by thiazole synthase. The precursors for this process include 1-deoxy-D-xylulose 5-phosphate, 2-iminoacetate, and a sulfur carrier protein named ThiS. An additional protein, ThiG, is essential for bringing all the components of the ring together at the enzyme’s active site.
- Final Step: The final step involves the decarboxylation of the thiazole intermediate, which reacts with the pyrophosphate derivative of phosphomethylpyrimidine to form ThMP.
- Regulation of Biosynthetic Pathways: The biosynthetic pathways of thiamine are regulated by riboswitches. In the presence of adequate thiamine, the thiamine binds to the mRNAs of the enzymes required for its synthesis, inhibiting their translation. In its absence, there is no inhibition, leading to the production of the necessary enzymes. The TPP riboswitch, responsible for this regulation, is unique as it is found in both eukaryotic and prokaryotic organisms.
- Laboratory Synthesis: The first total synthesis of thiamine was achieved in 1936. The process involved multiple steps, starting with the treatment of ethyl 3-ethoxypropanoate with ethyl formate, leading to the formation of a substituted pyrimidine. This was followed by various reactions to produce thiamine.
- Industrial Synthesis: Merck & Co. adapted the laboratory-scale synthesis in 1937, allowing for the manufacture of thiamine. However, alternative routes using the Grewe diamine intermediate were explored, leading to competitive manufacturing processes. Efficient routes to the diamine have been a subject of interest.
- Synthetic Analogues: Several synthetic analogues of thiamine, such as Benfotiamine, fursultiamine, and sulbutiamine, were developed primarily in Japan during the 1950s and 1960s. These analogues were designed to enhance thiamine absorption.
Thiamine transport
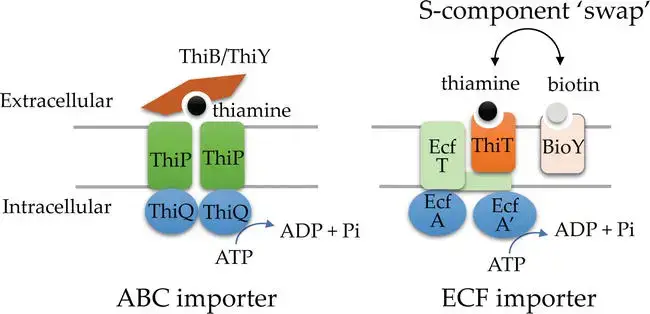
Thiamine, recognized as a vital micronutrient, undergoes active transportation into cells, moving against its concentration gradient. This transportation mechanism negates the necessity for the de novo synthesis of thiamine within the cells. Predictions suggest the presence of thiamine transporters in archaea, based on their similarity to bacterial transport systems or through the identification of potential transporter genes. These genes either exhibit genomic synteny with thiamine biosynthesis genes or are located downstream of ThDP-binding riboswitch motifs, commonly referred to as THI-box motifs .
The transporters responsible for the movement of thiamine and its precursors in bacteria, which are conserved in archaea, can be categorized into several types:
- ABC-type transporters such as ThiBPQ and ThiYXZ.
- A distinct class of ABC-type transporters known as energy coupling factor (ECF) importers.
- NiaP transporters, which belong to the major facilitator superfamily (MSF, IPR036259). These utilize an ion gradient for their function.
- PnuT transporters, which facilitate the diffusion of thiamine.
Both ECF and ABC transporters are primary active transporters. They utilize ATP hydrolysis to facilitate thiamine uptake, as evidenced by their conserved ATPases. The distinguishing factor between ECF and ABC transporters lies in their solute-binding protein type. ECF employs a transmembrane substrate-capture protein, termed the S component (ThiT), whereas ABC utilizes an extracytoplasmic solute binding protein, examples being ThiB or ThiY. ECF systems exhibit modularity, allowing ThiT and other S-components (like the biotin-specific BioY) to bind interchangeably to the system’s transmembrane (T) component. In contrast, ABC systems lack this modularity. Their solute-binding proteins (ThiB/Y) attach to the transporter’s extracytoplasmic domain.
Biochemical changes in B1 deficiency
A deficiency in thiamine can lead to a series of biochemical changes that disrupt these processes. The following exposition provides a detailed and sequential explanation of these alterations:
- Impaired Carbohydrate Metabolism: One of the primary roles of thiamine is to aid in the metabolism of carbohydrates. In its absence, carbohydrate metabolism becomes compromised. Consequently, there is an accumulation of pyruvate in the tissues, which is detrimental. Elevated levels of pyruvate are observed in the plasma, and it is also excreted in the urine.
- Pyruvate and the Blood-Brain Barrier: Under normal circumstances, pyruvate does not traverse the blood-brain barrier. However, in the context of thiamine deficiency, there is an alteration in this barrier. This change allows pyruvate to directly enter the brain. It is postulated that the accumulation of pyruvate in the brain disrupts its metabolism, potentially leading to conditions like polyneuritis.
- Impairment in Nerve Impulse Transmission: Thiamine is essential for the production of Thiamine Pyrophosphate (TPP), which is crucial for nerve impulse transmission. A deficiency in thiamine leads to a lack of TPP, thereby hampering the transmission of nerve impulses.
- Reduced Transketolase Activity: Transketolase is an enzyme that plays a role in the pentose phosphate pathway. In the absence of adequate thiamine, the activity of transketolase in erythrocytes (red blood cells) is diminished. Measuring the activity of RBC transketolase serves as a reliable diagnostic test to determine thiamine deficiency.
Wernicke-Korsakoff syndrome
Wernicke-Korsakoff syndrome, often referred to as cerebral beri-beri, is a neurological disorder predominantly observed in individuals with chronic alcoholism. Delving into the intricacies of this condition provides a clearer understanding of its origins and manifestations.
- Association with Alcoholism: Chronic alcohol consumption elevates the body’s demands for thiamine. Alcohol not only increases the requirement for this essential nutrient but also adversely affects its absorption and utilization. Therefore, individuals who consume alcohol excessively are at a heightened risk of developing thiamine deficiency.
- Thiamine Deficiency: Thiamine, commonly known as Vitamin B1, plays a pivotal role in various metabolic processes within the body. An insufficient intake of thiamine, coupled with impaired intestinal absorption, often precipitated by chronic alcoholism, can lead to a severe deficiency. This deficiency is the primary factor responsible for the onset of Wernicke-Korsakoff syndrome.
- Clinical Manifestations: The syndrome is characterized by a constellation of neurological symptoms. One of the most prominent manifestations is memory loss. Affected individuals often display apathy, indicating a lack of interest or enthusiasm in activities they once enjoyed. Additionally, a distinctive feature of this condition is the rhythmical to-and-fro motion of the eyeballs, a phenomenon that further underscores the neurological impact of the syndrome.
Thiamine deficiency due to thiaminase and pyrithiamine
However, certain factors can lead to its deficiency, with thiaminase and pyrithiamine being two significant contributors.
- Thiaminase and Seafoods: Thiaminase is an enzyme found in specific seafoods. When consumed, this enzyme can degrade thiamine by cleaving its structure, specifically splitting the pyrimidine and thiazole rings. Therefore, the dietary inclusion of seafoods rich in thiaminase can result in thiamine destruction, leading to its deficiency. A notable observation is the incidence of beri-beri in certain regions of Japan. This condition, which is a manifestation of thiamine deficiency, is attributed to the consumption of fish that are rich in thiaminase.
- Pyrithiamine and Plants: Pyrithiamine is a structural analogue of thiamine and acts as its antimetabolite. This compound is naturally found in specific plants, particularly ferns. When consumed, pyrithiamine interferes with the metabolic functions of thiamine, leading to its deficiency. A clear example of this is observed in animals like horses and cattle. Often termed “fern poisoning,” these animals develop thiamine deficiency when they overconsume fern plants containing pyrithiamine.
References
- Maupin-Furlow, J. A. (2018). Vitamin B1 (Thiamine) Metabolism and Regulation in Archaea. InTech. doi: 10.5772/intechopen.77170
- Martel JL, Kerndt CC, Doshi H, et al. Vitamin B1 (Thiamine) [Updated 2022 Oct 12]. In: StatPearls [Internet]. Treasure Island (FL): StatPearls Publishing; 2023 Jan-. Available from: https://www.ncbi.nlm.nih.gov/books/NBK482360/
- https://www.netmeds.com/health-library/post/vitamin-b1-functions-food-sources-deficiencies-and-toxicity
- https://www.onlinebiologynotes.com/vitamin-b-complex-structure-coenzyme-forms-and-biological-roles/