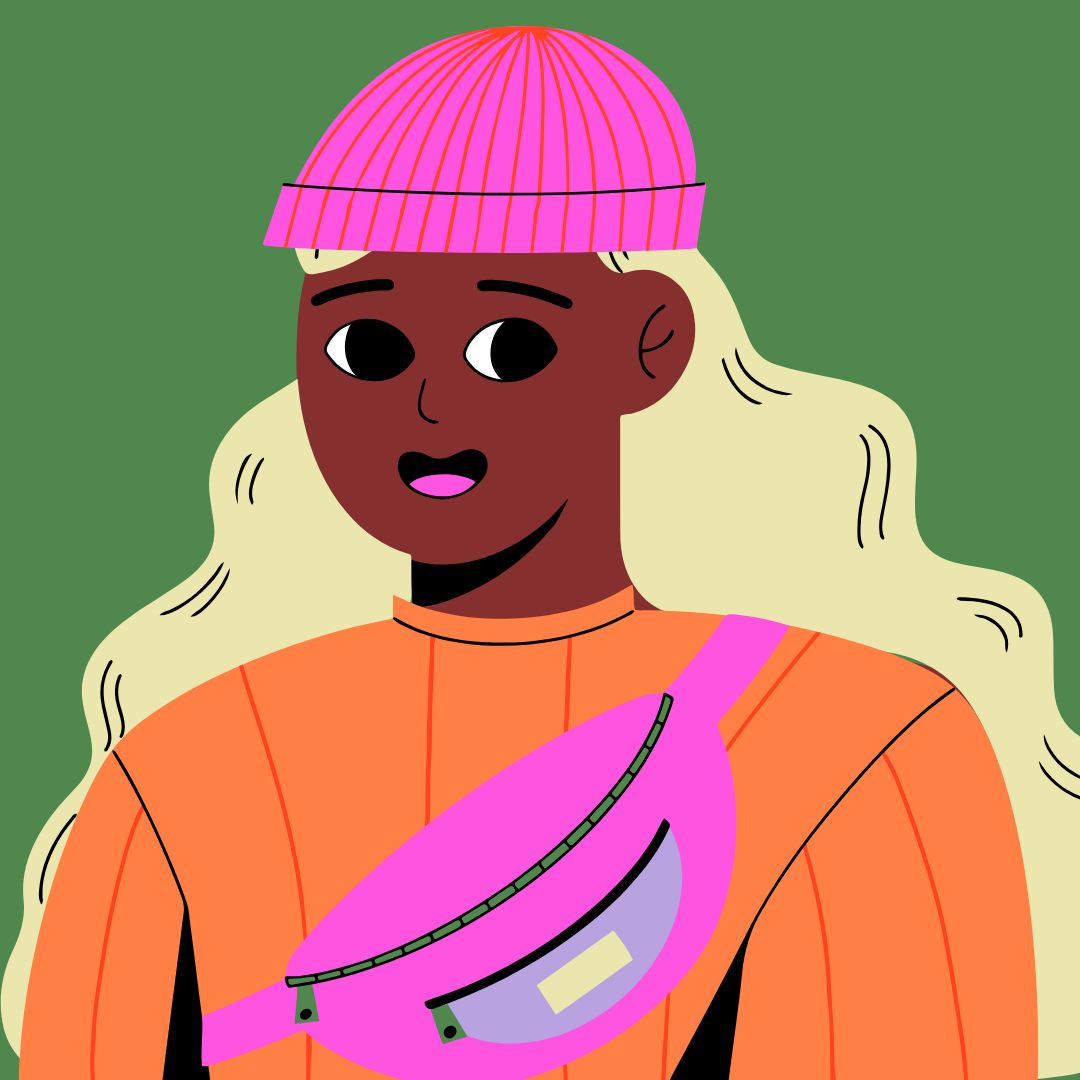
Sourav Pan
Transcript
Domain Bacteria contains prokaryotic organisms with simple but efficient cellular structures.
Bacteria are prokaryotes, characterized by cells without a true nucleus or membrane-bound organelles.
A defining feature of bacteria is their cell wall containing peptidoglycan, a unique polymer not found in other domains.
Bacterial genetic material consists of a single circular chromosome located in the nucleoid region, without being enclosed by a nuclear membrane.
Many bacteria move using flagella, which are simple protein structures that rotate like propellers.
Bacteria display remarkable morphological diversity, including spherical cocci, rod-shaped bacilli, and spiral spirilla forms.
Ecologically, bacteria play vital roles as decomposers, nitrogen fixers, and both beneficial and pathogenic species.
As decomposers, they break down organic matter. Nitrogen fixers convert atmospheric nitrogen into forms plants can use. Some species benefit our health while others cause diseases.
Domain Archaea consists of prokaryotic organisms that often inhabit some of the most extreme environments on Earth.
Archaea are known as extremophiles because they can thrive in conditions that would be lethal to most other life forms.
Thermophiles are heat-loving archaea that can survive in temperatures up to 122 degrees Celsius, often found in hot springs and hydrothermal vents.
Halophiles thrive in extremely salty environments with concentrations greater than two molar sodium chloride, such as salt lakes and the Dead Sea.
Acidophiles can survive in highly acidic environments with pH values below 3, like acidic hot springs and mine drainage.
Methanogens produce methane as a metabolic byproduct and are often found in anaerobic environments like swamps and the digestive tracts of animals.
One key adaptation that allows archaea to survive in these extreme environments is their unique membrane composition.
Unlike bacteria and eukaryotes which have ester linkages in their membrane lipids, archaea have ether linkages between their lipid and glycerol components.
These ether linkages make archaeal cell membranes more resistant to extreme temperatures, high salinity, and acidic conditions.
Despite their prokaryotic cell structure, archaea show surprising similarities to eukaryotes at the molecular level.
One major example is their RNA polymerase machinery. Bacteria have a single RNA polymerase, while both archaea and eukaryotes have multiple RNA polymerases.
The structure and complexity of archaeal RNA polymerases closely resembles those found in eukaryotes, suggesting an evolutionary relationship between these domains.
These unique molecular features of archaea have led scientists to recognize them as a distinct domain of life, separate from both bacteria and eukaryotes.
Cell walls and membranes are fundamental structures that differ significantly across the three domains of life.
Cell walls provide structural support, protect against osmotic pressure, and create barriers against environmental threats.
Bacteria possess cell walls made of peptidoglycan, a mesh of sugars and amino acids.
Gram-positive bacteria have a thick peptidoglycan layer, while Gram-negative bacteria have a thinner layer with an outer membrane. These structures are targets for antibiotics like penicillin.
Archaea have either S-layer protein arrays or pseudopeptidoglycan as their cell wall structure.
Unlike bacterial peptidoglycan, archaeal cell walls have different chemical compositions. This makes them resistant to antibiotics that target bacterial cell walls and allows them to adapt to extreme environments.
In the eukaryotic domain, cell wall composition varies dramatically between different kingdoms.
Plants have cellulose cell walls providing structural rigidity. Fungi use chitin in their cell walls for protection, similar to the material in insect exoskeletons. Animal cells lack a cell wall entirely, relying solely on their plasma membrane.
Cell membranes also differ significantly across the three domains.
Bacterial membranes consist of phospholipid bilayers with straight-chain fatty acids, and contain hopanoids instead of sterols.
Archaeal membranes have unique ether-linked lipids with branched isoprenoid chains, making them more resistant to extreme environments like high temperatures and acidity.
Eukaryotic membranes contain both phospholipids and sterols such as cholesterol, which helps regulate membrane fluidity.
These structural differences have important functional implications. Cell wall composition determines antibiotic targets, membrane structure enables environmental adaptations, and wall type affects cell shape and rigidity.
The endosymbiotic theory explains how complex eukaryotic cells evolved from simpler prokaryotic ancestors.
Proposed by Lynn Margulis in the 1960s, the endosymbiotic theory suggests that mitochondria and chloroplasts originated as free-living bacteria that were engulfed by primitive eukaryotic cells.
Around 1.5 billion years ago, primitive eukaryotic cells existed alongside free-living bacteria.
According to the theory, the primitive cell engulfed an aerobic bacterium, which continued to survive inside the host cell.
Similarly, a photosynthetic bacterium was engulfed and became the chloroplast, enabling the cell to perform photosynthesis.
This relationship provided mutual benefits. The host cell offered protection and nutrients, while the bacteria provided specialized functions like energy production and photosynthesis.
Multiple lines of evidence support the endosymbiotic theory. Let’s examine them one by one.
First, mitochondria and chloroplasts are similar in size to bacteria, ranging from 1 to 10 micrometers. Their structural components are also remarkably similar.
Second, mitochondria and chloroplasts reproduce independently from the host cell through binary fission, just like bacteria do.
Third, mitochondria and chloroplasts contain their own circular DNA and 70S ribosomes, which are distinctly different from the linear DNA and 80S ribosomes in the eukaryotic nucleus.
Fourth, both mitochondria and chloroplasts possess a double membrane structure, with the inner membrane believed to be from the original bacterium and the outer from the host cell. Fifth, they share similar biochemical processes with bacteria, including electron transport chains and protein synthesis mechanisms.
The endosymbiotic theory provides a compelling explanation for the evolution of complex eukaryotic cells through a cooperative relationship between different organisms.
The three domains of life each play crucial and distinctive roles in Earth’s ecosystems.
Bacteria serve as essential decomposers, breaking down organic matter and recycling nutrients. Cyanobacteria are crucial primary producers, generating oxygen through photosynthesis. Many bacteria fix nitrogen in soil and plant roots, making it available to other organisms. Bacterial communities form vast microbiomes in virtually all environments.
Archaea are famous for thriving in extreme environments like hot springs, salt lakes, and deep-sea vents. Methanogenic archaea produce methane in anaerobic habitats such as wetlands and animal digestive tracts. They play crucial roles in global carbon and sulfur cycles. Recent research has revealed their surprising abundance in ocean plankton communities.
Eukaryotes form complex multicellular ecosystems and diverse habitats, from forests to coral reefs. Plants and algae are the dominant photosynthetic organisms, forming the base of most food webs. Fungi serve as major decomposers, breaking down complex organic matter. Animal populations regulate ecosystem dynamics through predation and herbivory.
The three domains interact in complex biogeochemical cycles, like the carbon and nitrogen cycles. Bacteria decompose organic matter, releasing nutrients used by plants and algae. Archaea transform compounds in extreme environments that other organisms cannot access. Together, they maintain the balance of elements essential for life.
Together, the three domains regulate climate and maintain the balance of gases in the atmosphere. They purify water, form soil, and sustain nutrient cycles. No domain exists in isolation—each depends on the others. The interactions between Bacteria, Archaea, and Eukarya are essential for all life on Earth and the functioning of every ecosystem.
Study Materials
No study materials available for this video.
Helpful: 0%
Related Videos
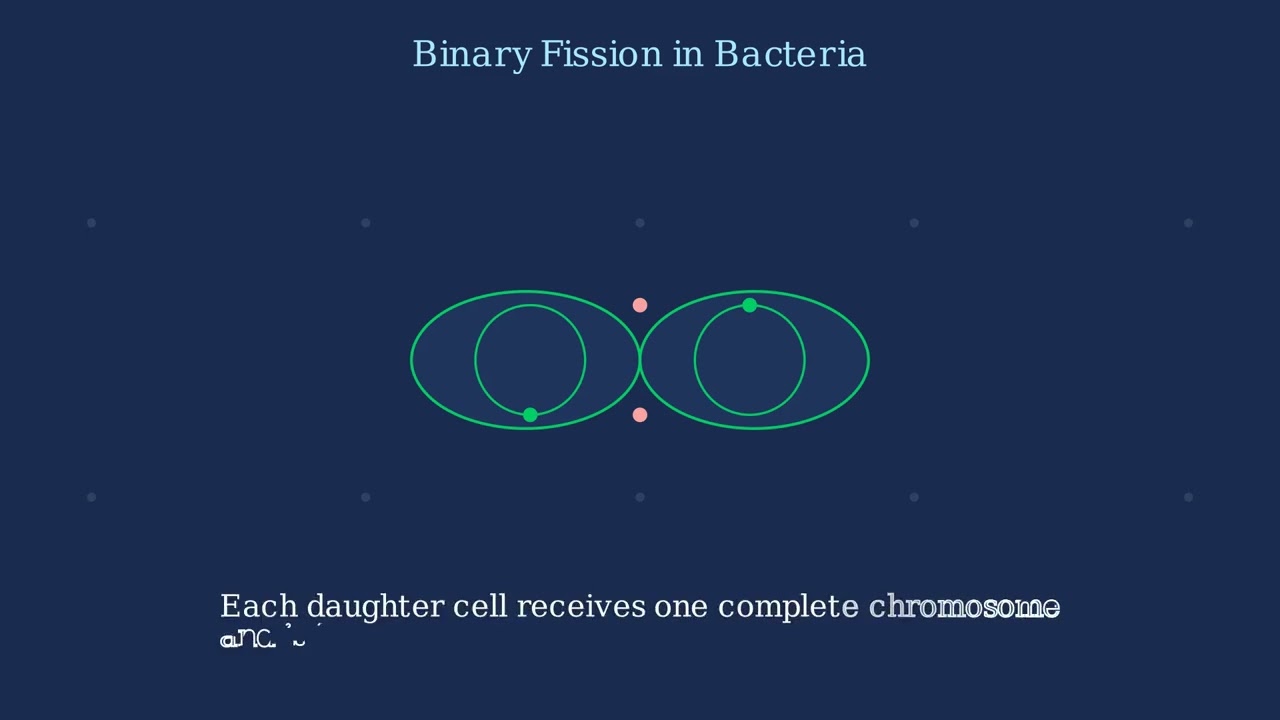
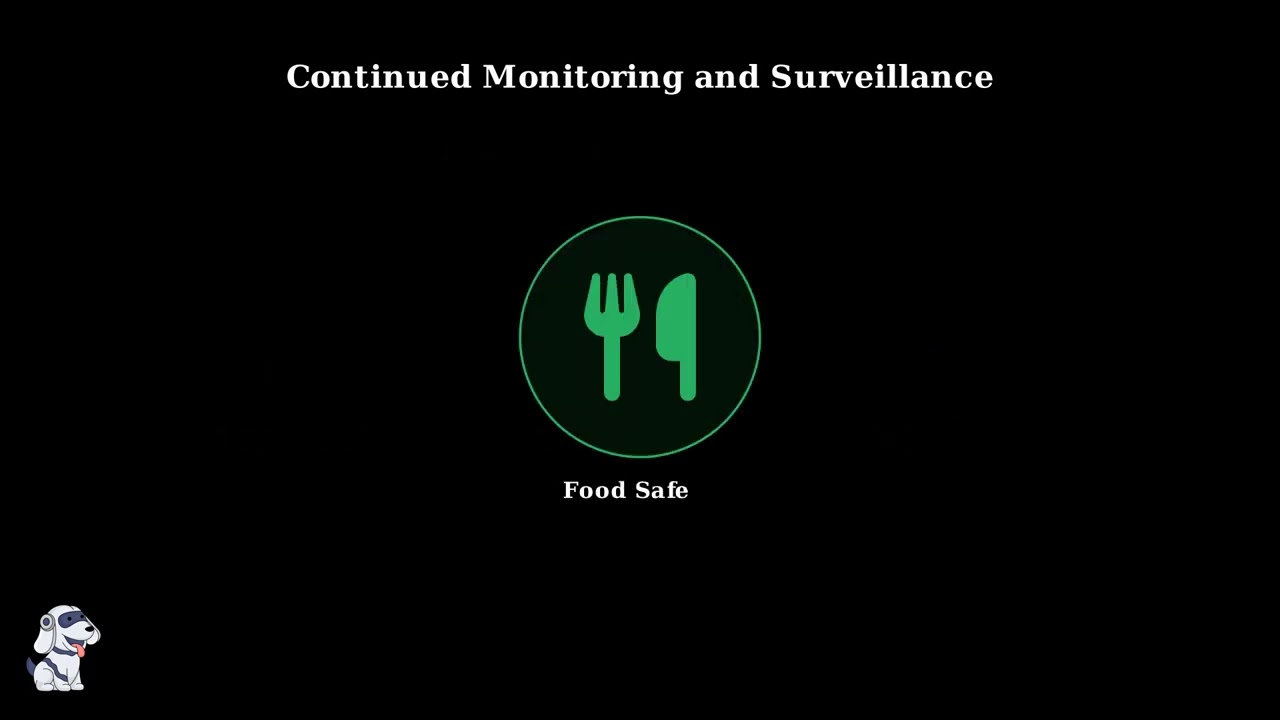
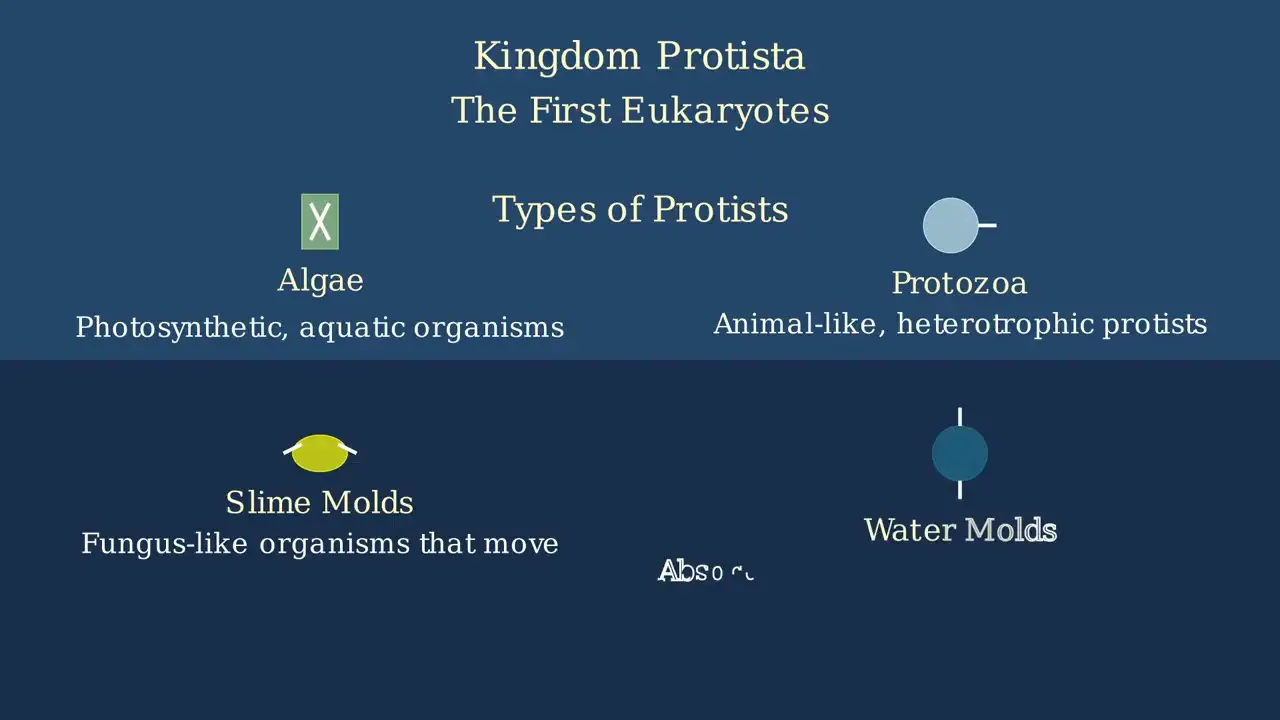
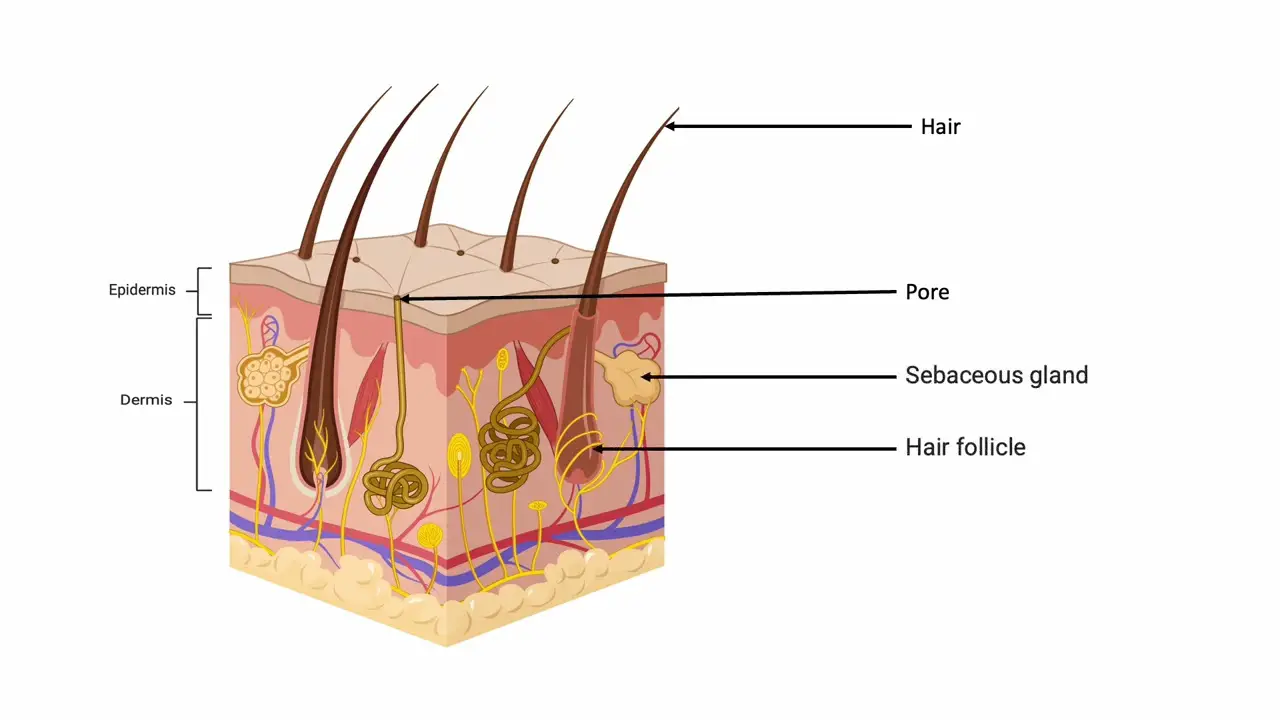
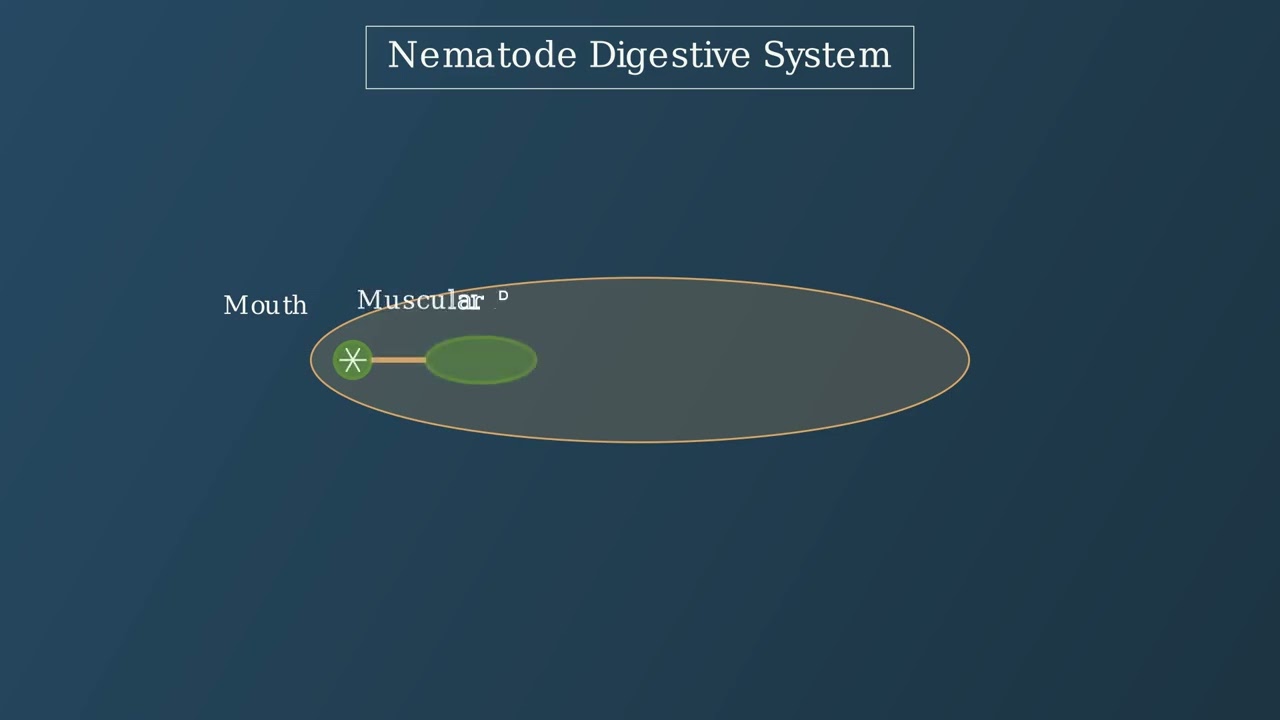
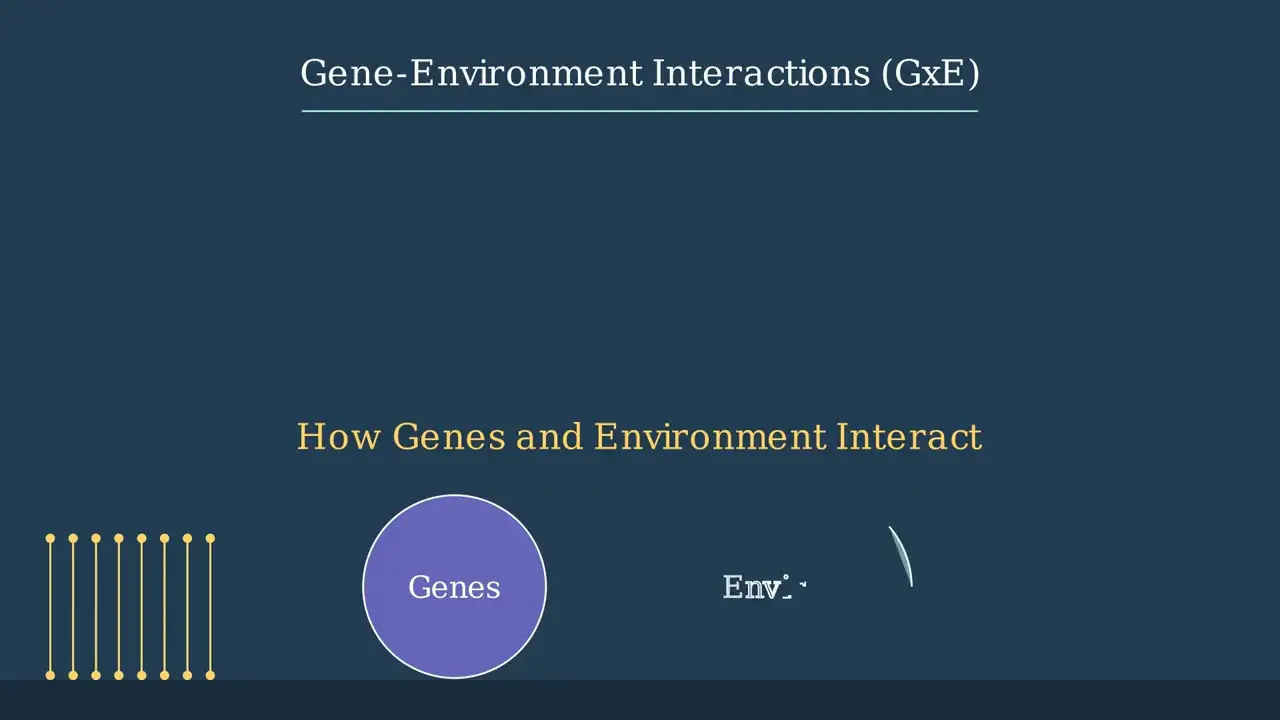
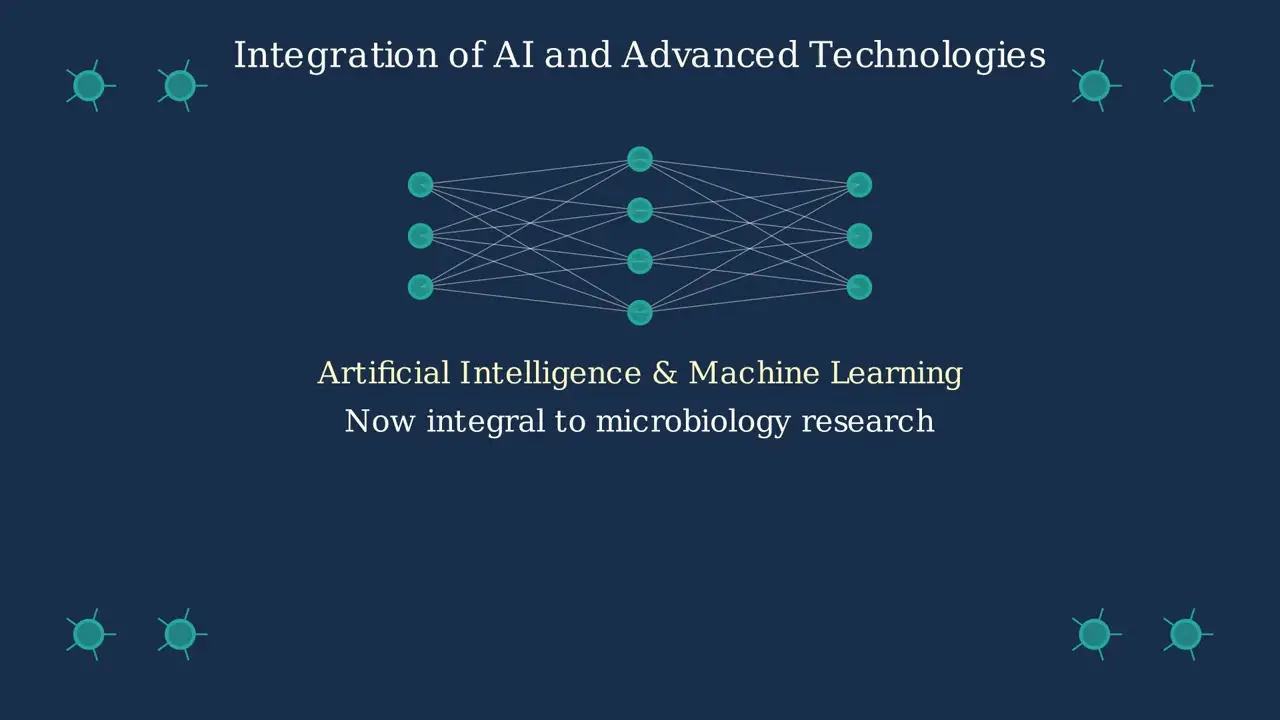
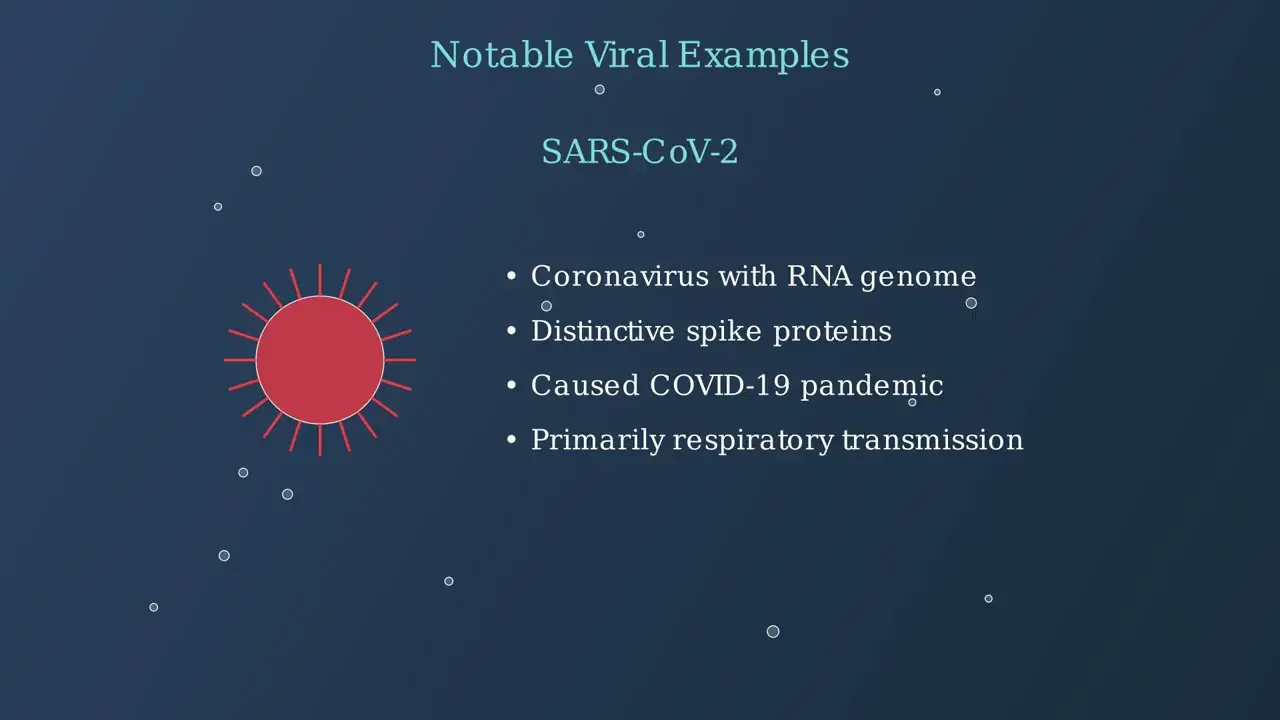
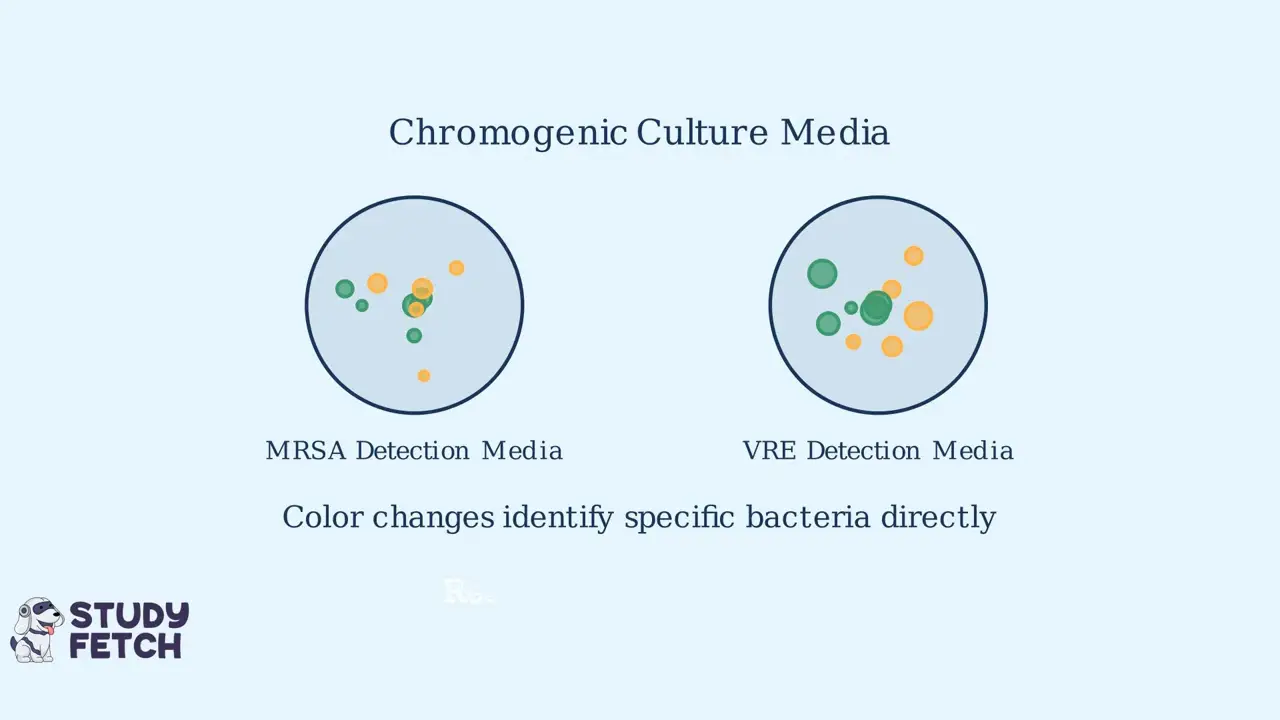
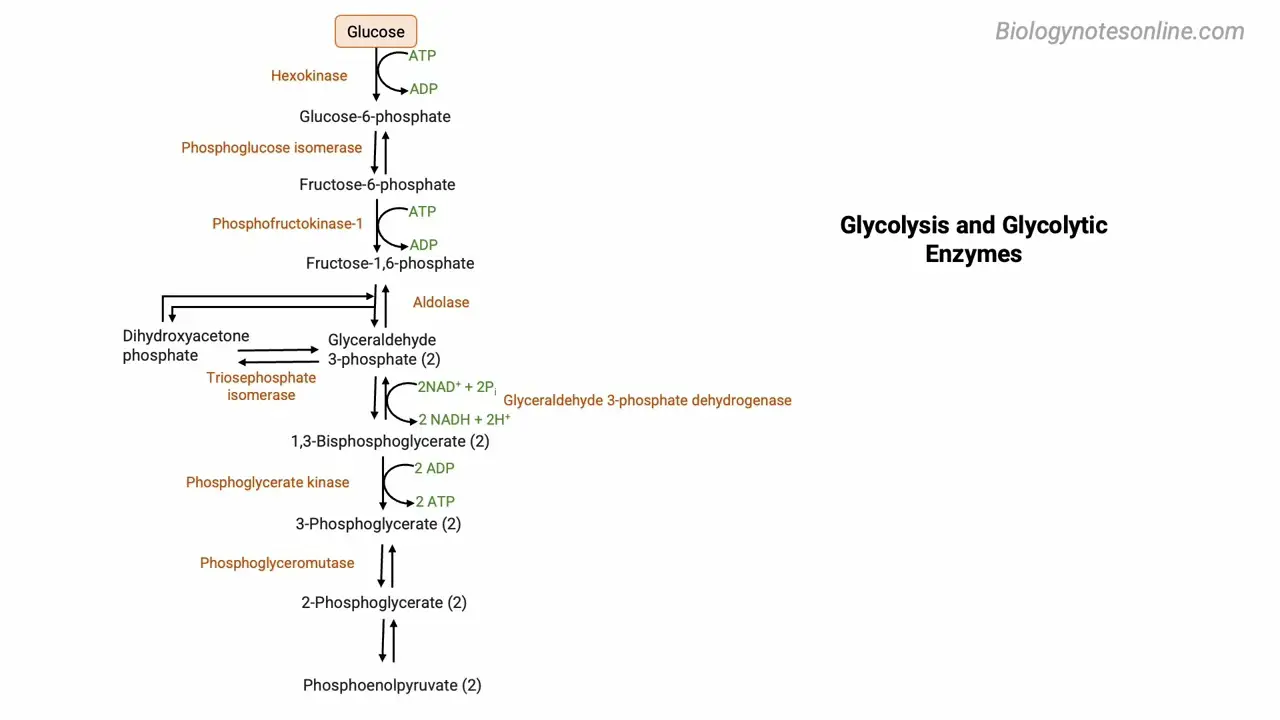