What is Urea Cycle?
- The urea cycle, also known as the ornithine cycle, is a vital metabolic pathway responsible for the conversion of ammonia into urea. This cycle plays a pivotal role in the body’s mechanism to eliminate excess nitrogen.
- Nitrogen is indispensable for the synthesis of proteins, nucleic acids, and other essential molecules. However, the degradation of proteins can lead to the accumulation of ammonia, a substance that is toxic to cells and tissues. Therefore, it becomes imperative for the body to have a system in place to manage this excess ammonia.
- The urea cycle offers a solution to this challenge. Through a series of intricate biochemical reactions, ammonia is transformed into urea, a compound that is far less harmful. These reactions occur primarily in the liver and are facilitated by both mitochondrial and cytosolic enzymes.
- Besides its role in humans, the urea cycle is also significant in amphibians and mammals. These organisms, termed ureotelic, utilize the cycle to dispose of the highly toxic ammonia by converting it into urea.
- The discovery of the urea cycle can be credited to Hans Krebs and Kurt Henseleit in 1932. Within the body, ammonia is generated from various processes, including amino acid catabolism, deaminations, and during prolonged periods of starvation.
- Then, it becomes essential for all animals to excrete this ammonia in some form. Aquatic organisms, for instance, directly excrete ammonia and are thus called ammonotelic.
- However, mammals and amphibians cannot directly excrete ammonia due to its toxic nature. Therefore, they transform it into urea, a simpler and less toxic compound.
- Once formed in the liver, urea is transported to the kidneys via the bloodstream. It is then excreted as a component of urine. This elimination process is of paramount importance. If nitrogenous waste is not efficiently removed, its buildup can have detrimental effects on the body.
- In contrast to ammonia, urea is inert, water-soluble, and can be effortlessly excreted in urine, emphasizing the importance of the urea cycle in maintaining the body’s internal equilibrium.
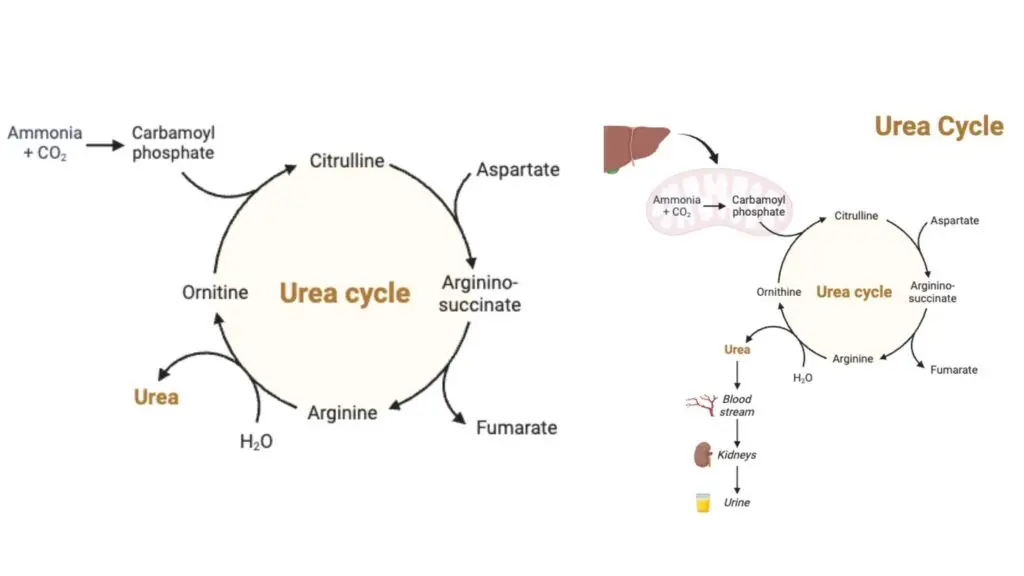
Definition of Urea Cycle
The urea cycle is a series of biochemical reactions in the liver that convert ammonia, a toxic byproduct of protein metabolism, into urea, a less harmful compound that is excreted in the urine.
Urea Cycle Reactions/Urea Cycle Steps
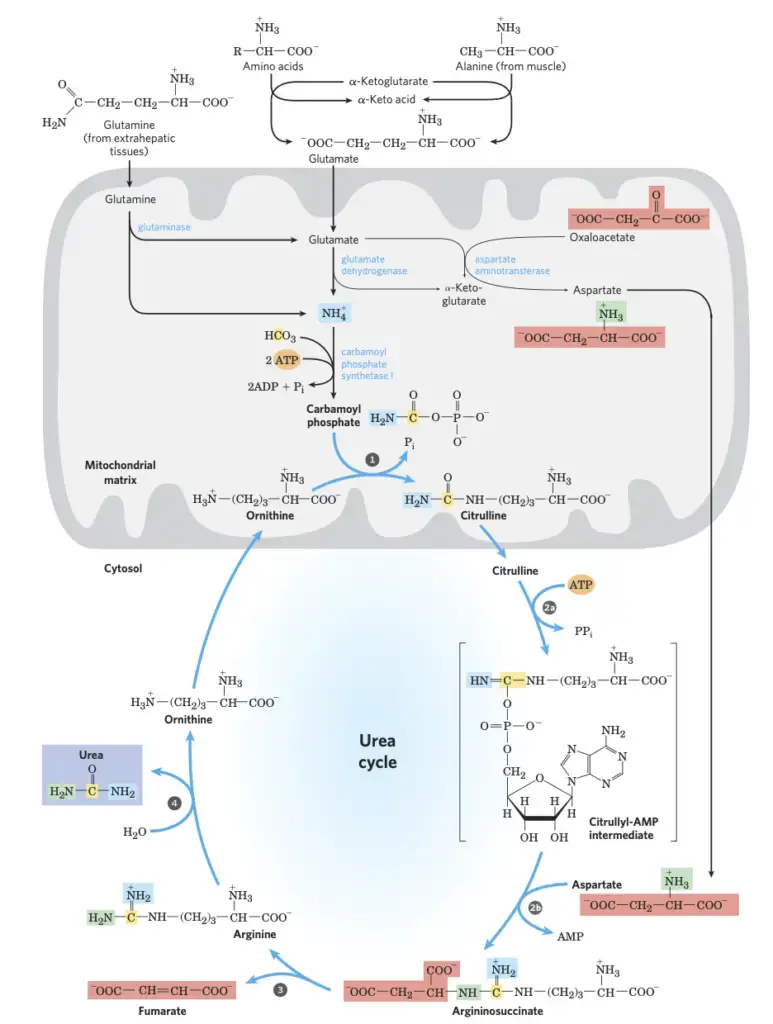
The urea cycle is a critical metabolic pathway that operates primarily in the liver, facilitating the detoxification of ammonia into urea. This cycle encompasses both the mitochondrial matrix and the cytosol of the cell. Here is a detailed and sequential elucidation of the steps involved in the urea cycle:
- Formation of Carbamoyl Phosphate: Initiated within the mitochondrial matrix, ammonia, which primarily arises from various metabolic pathways, combines with carbon dioxide (represented as HCO₃⁻) to produce carbamoyl phosphate. This reaction is ATP-dependent and is catalyzed by the enzyme carbamoyl phosphate synthetase I. It’s essential to note that this mitochondrial enzyme is distinct from its cytosolic counterpart, which plays a role in pyrimidine biosynthesis.
- Formation of Citrulline: In this step, the carbamoyl group from carbamoyl phosphate is transferred to ornithine, producing citrulline and releasing inorganic phosphate (Pi). This reaction is facilitated by the enzyme ornithine transcarbamoylase. Ornithine, although not one of the standard amino acids in proteins, is pivotal in nitrogen metabolism. Once formed, citrulline is then transported from the mitochondria to the cytosol.
- Synthesis of Argininosuccinate: The next phase involves the introduction of the second amino group. Aspartate, generated in the mitochondria and then transported to the cytosol, combines with citrulline to form argininosuccinate. This condensation reaction, occurring in the cytosol and catalyzed by argininosuccinate synthetase, requires ATP and progresses through a citrullyl-AMP intermediate.
- Formation of Arginine and Fumarate: Argininosuccinate is subsequently cleaved by the enzyme argininosuccinase to yield arginine and fumarate. The fumarate produced can be converted to malate and then transported into the mitochondria, where it integrates with the citric acid cycle intermediates.
- Production of Urea and Ornithine: The final step of the urea cycle involves the cleavage of arginine to produce urea and ornithine. This reaction is catalyzed by the cytosolic enzyme arginase. The ornithine produced is then transported back into the mitochondria, marking the beginning of another cycle iteration.
It’s worth highlighting that the enzymes involved in the urea cycle appear to be strategically clustered. The product of one enzymatic reaction is directly channeled to the subsequent enzyme in the pathway. For instance, the citrulline exiting the mitochondrion is directly passed to the active site of argininosuccinate synthetase, ensuring efficiency. This channeling continues for argininosuccinate, arginine, and ornithine, with only urea being released into the general cytosolic pool of metabolites.
Overall Reaction of the urea cycle
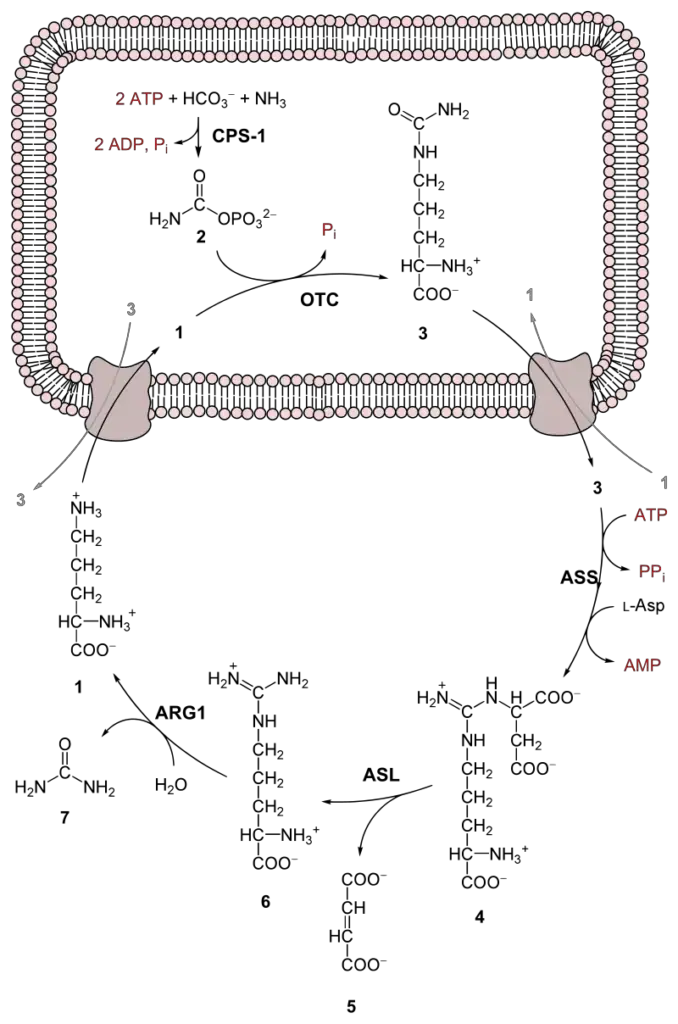
The urea cycle is a fundamental metabolic pathway responsible for the detoxification of ammonia and the synthesis of urea. The overall stoichiometry of the urea cycle can be summarized by the following equation:
Aspartate + NH3 + HCO3− + 3ATP + H2O → urea+fumarate + 2ADP + AMP + 2Pi + PPi
This equation highlights the conversion of aspartate, ammonia, bicarbonate, and three molecules of ATP into urea, fumarate, two molecules of ADP, AMP, inorganic phosphate (Pi), and pyrophosphate (PPi). Notably, the synthesis of urea is an irreversible process due to the consumption of four high-energy phosphate bonds, resulting in a large, negative change in Gibbs free energy (∆G).
Furthermore, the nitrogen atoms in the urea molecule have distinct origins. One nitrogen is derived from free ammonia (NH3), while the other nitrogen is sourced from aspartate. The amino acid glutamate plays a pivotal role in this process. Glutamate serves as the immediate precursor for both the ammonia and aspartate nitrogen. Specifically, ammonia is produced through the oxidative deamination of glutamate by the enzyme glutamate dehydrogenase. On the other hand, aspartate nitrogen is formed through the transamination of oxaloacetate by aspartate transaminase (AST).
In essence, both nitrogen atoms of the urea molecule can be traced back to glutamate. Glutamate, in turn, assimilates nitrogen from other amino acids, emphasizing its central role in nitrogen metabolism.
Step by Step Reactions of the urea cycle
- Formation of Carbamoyl Phosphate
- Reactants: Ammonia (NH₃), bicarbonate (HCO₃⁻), and two molecules of ATP.
- Products: Carbamoyl phosphate, two molecules of ADP, and inorganic phosphate (Pi).
- Catalyzed by: The enzyme carbamoyl phosphate synthetase 1 (CPS1).
- Location: This reaction takes place in the mitochondria.
- Function: This is the initial and rate-limiting step of the urea cycle, where ammonia is captured in the form of carbamoyl phosphate.
- Formation of Citrulline
- Reactants: Carbamoyl phosphate and ornithine.
- Products: Citrulline and inorganic phosphate (Pi).
- Catalyzed by: The enzyme ornithine transcarbamoylase (OTC), with the assistance of co-factors zinc and biotin.
- Location: This reaction also occurs in the mitochondria.
- Function: Ornithine and carbamoyl phosphate combine to form citrulline, which is then transported to the cytosol for the next steps.
- Formation of Argininosuccinate
- Reactants: Citrulline, aspartate, and ATP.
- Products: Argininosuccinate, AMP, and pyrophosphate (PPi).
- Catalyzed by: The enzyme argininosuccinate synthetase (ASS).
- Location: This reaction takes place in the cytosol.
- Function: Citrulline and aspartate combine, using energy from ATP, to form argininosuccinate.
- Cleavage of Argininosuccinate
- Reactants: Argininosuccinate.
- Products: Arginine and fumarate.
- Catalyzed by: The enzyme argininosuccinate lyase (ASL).
- Location: This reaction occurs in the cytosol.
- Function: Argininosuccinate is split into arginine and fumarate. Fumarate can then enter the citric acid cycle.
- Formation of Urea
- Reactants: Arginine and water (H₂O).
- Products: Ornithine and urea.
- Catalyzed by: The enzyme arginase (ARG1) with the co-factor manganese.
- Location: This reaction takes place in the cytosol.
- Function: Arginine is hydrolyzed to produce urea, the primary nitrogenous waste product in humans. Ornithine, the other product, is transported back to the mitochondria to participate in the cycle again.
Step | Reactants | Products | Enzyme/Co-factors | Location |
---|---|---|---|---|
1 | NH3 + HCO₃⁻ + 2ATP | carbamoyl phosphate + 2ADP + Pi | CPS1 | mitochondria |
2 | carbamoyl phosphate + ornithine | citrulline + Pi | OTC (with zinc and biotin) | mitochondria |
3 | citrulline + aspartate + ATP | argininosuccinate + AMP + PPi | ASS | cytosol |
4 | argininosuccinate | arginine + fumarate | ASL | cytosol |
5 | arginine + H2O | ornithine + urea | ARG1 (with manganese) | cytosol |
The Citric Acid and Urea Cycles Can Be Linked
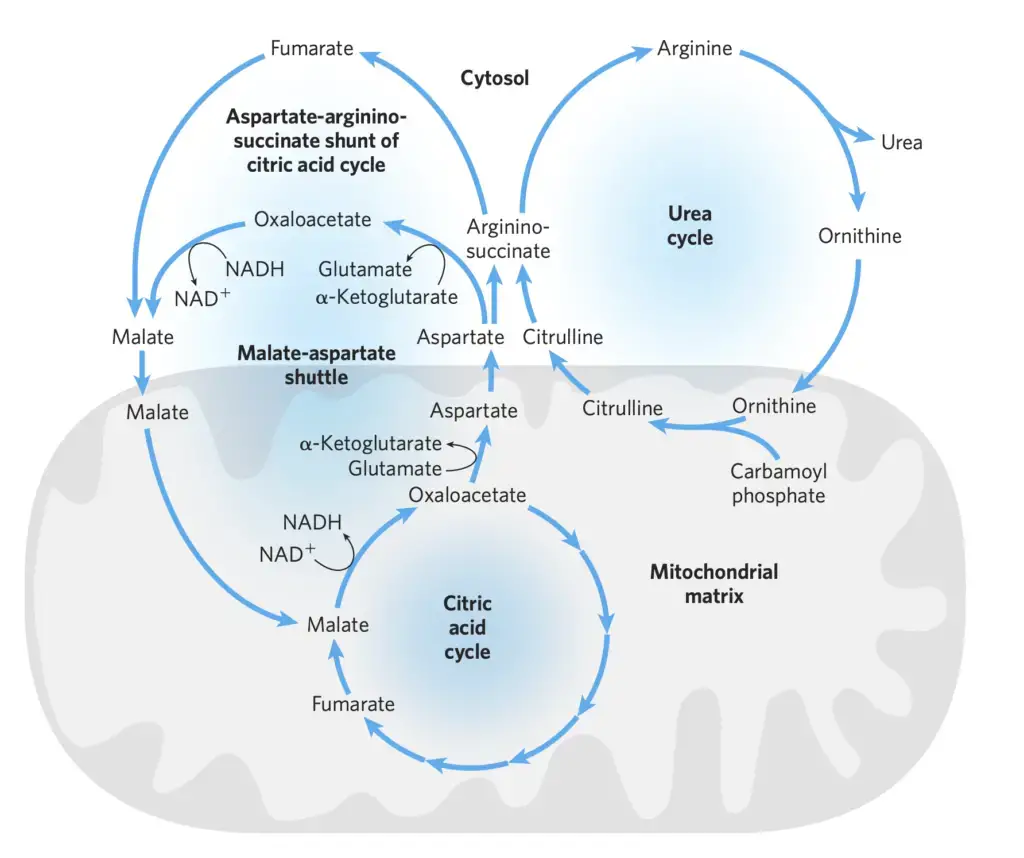
The intricate relationship between the urea cycle and the citric acid cycle, often referred to as the “Krebs bicycle,” showcases the sophisticated interplay of metabolic pathways within the cell. This relationship is particularly evident in the liver, where both cycles operate in tandem, ensuring the efficient processing of amino groups and carbon skeletons of amino acids.
- Interconnection of the Cycles: The fumarate produced during the argininosuccinase reaction in the urea cycle is also an intermediate in the citric acid cycle. This establishes a direct link between the two cycles. Furthermore, the nitrogen-acquiring reactions in the urea cycle involve the consumption of ATP, emphasizing the energy-dependent nature of these processes.
- The Aspartate-Argininosuccinate Shunt: This shunt provides a metabolic bridge between the pathways that process the amino groups and carbon skeletons of amino acids. Aspartate, formed in the mitochondria by the transamination of oxaloacetate and glutamate, is transported to the cytosol. Here, it serves as a nitrogen donor in the urea cycle reaction facilitated by argininosuccinate synthetase. This intricate interplay effectively links the fates of amino groups and carbon skeletons.
- Transport and Conversion: Several enzymes of the citric acid cycle, such as fumarase and malate dehydrogenase, exist in both the cytosol and the mitochondria as isozymes. Fumarate, produced in the cytosol, can be converted to malate, which can either be utilized in the cytosol or transported into the mitochondria to participate in the citric acid cycle. This interplay is further intertwined with the malate-aspartate shuttle, a mechanism that imports reducing equivalents into the mitochondrion.
- The Malate-Aspartate Shuttle: Reducing equivalents, in the form of NADH produced by various metabolic processes, cannot directly cross the mitochondrial inner membrane. Instead, these equivalents are transported into the mitochondrion via the malate-aspartate shuttle. In this process, aspartate is converted to oxaloacetate in the cytosol, which is then reduced to malate using NADH. The malate is subsequently transported into the mitochondrial matrix, where it can be converted back to oxaloacetate, generating NADH in the process.
- Balance of Amino Acids: Maintaining a balance between the concentrations of glutamate and aspartate in the cytosol is crucial. The enzyme aspartate aminotransferase (AAT), also known as glutamate-oxaloacetate transaminase (GOT), plays a pivotal role in transferring amino groups between these amino acids. This enzyme is highly active in hepatocytes and is a key indicator in diagnosing various medical conditions when its levels are detected in the blood due to tissue damage.
In conclusion, the urea and citric acid cycles are not isolated metabolic pathways but are intricately linked, showcasing the cell’s ability to efficiently manage and utilize its resources. The interconnected nature of these cycles ensures that the cell can effectively process and detoxify amino groups, while also managing energy and carbon skeletons.
Genetic Defects in the Urea Cycle
Genetic defects in the urea cycle can have profound implications for an individual’s ability to metabolize proteins. The urea cycle plays a pivotal role in converting toxic ammonia, produced during the breakdown of amino acids, into urea, which is then excreted from the body. A malfunction in this cycle can lead to severe health consequences.
- Implications of Urea Cycle Defects: Individuals with genetic defects in any enzyme of the urea formation process face challenges when consuming protein-rich diets. When they ingest amino acids beyond the minimum daily requirements for protein synthesis, these amino acids are deaminated in the liver. This process produces free ammonia. However, due to the defect in the urea cycle, this ammonia cannot be converted to urea and released into the bloodstream. As a result, ammonia, which is highly toxic, accumulates in the body. Depending on the specific enzyme that is absent, this can lead to hyperammonemia or an accumulation of one or more urea cycle intermediates. Since most steps in the urea cycle are irreversible, the missing enzyme activity can often be pinpointed by identifying which cycle intermediate is present in abnormally high concentrations in the blood or urine.
- Dietary Considerations: While the breakdown of amino acids can be detrimental to those with urea cycle deficiencies, completely avoiding proteins is not a viable solution. This is because humans cannot synthesize half of the 20 common amino acids. These essential amino acids must be obtained through the diet.
- Available Treatments: Various treatments have been developed to assist individuals with urea cycle defects. One approach involves the dietary administration of aromatic acids like benzoate or phenylbutyrate. These acids help reduce blood ammonia levels. For instance, benzoate transforms into benzoyl-CoA, which then combines with glycine to produce hippurate. This process consumes glycine, prompting its regeneration and the uptake of ammonia. Similarly, phenylbutyrate is converted to phenylacetate, which then combines with glutamine to form phenylacetylglutamine. This process also results in the uptake of ammonia. Both hippurate and phenylacetylglutamine are non-toxic and are excreted through urine.Specific treatments are tailored to particular enzyme deficiencies. For example, a deficiency in N-acetylglutamate synthase can be addressed by administering carbamoyl glutamate, an analog of N-acetylglutamate. This analog effectively activates carbamoyl phosphate synthetase I. In cases of deficiencies in enzymes like ornithine transcarbamoylase, argininosuccinate synthetase, and argininosuccinase, supplementing the diet with arginine proves beneficial. However, these treatments often necessitate strict dietary control and essential amino acid supplements. In rare instances of arginase deficiency, arginine must be excluded from the diet, as it is the substrate for the malfunctioning enzyme.
In conclusion, genetic defects in the urea cycle present significant challenges in protein metabolism. However, with careful dietary management and specific treatments, these challenges can be addressed, ensuring the well-being of affected individuals.
Important enzymes Involve in Urea Cycle
The urea cycle is a crucial metabolic pathway responsible for the detoxification of ammonia and the production of urea. This cycle involves a series of enzymatic reactions that convert toxic ammonia into urea, which is then excreted from the body. Several enzymes play pivotal roles in ensuring the smooth functioning of this cycle. Here is a detailed and sequential explanation of the essential enzymes involved in the urea cycle:
- Carbamoyl Phosphate Synthetase I: This enzyme is located in the mitochondria and plays a foundational role in the urea cycle. It catalyzes the conversion of ammonium and bicarbonate into carbamoyl phosphate. This reaction is the rate-limiting step, meaning it determines the overall speed of the urea cycle. The formation of carbamoyl phosphate requires the expenditure of two ATP molecules, emphasizing the energy-intensive nature of this step.
- Ornithine Transcarbamoylase: Situated in the mitochondria, ornithine transcarbamoylase facilitates the combination of ornithine and carbamoyl phosphate. The result of this reaction is the formation of citrulline. Therefore, this enzyme plays a pivotal role in advancing the cycle by producing citrulline, a key intermediate.
- Argininosuccinate Synthetase: Transitioning to the cytosol, argininosuccinate synthetase takes center stage. This enzyme catalyzes the condensation of citrulline with aspartate, leading to the formation of arginosuccinate. This reaction is energy-dependent, requiring one ATP molecule for completion.
- Argininosuccinate Lyase: Also located in the cytosol, argininosuccinate lyase is responsible for cleaving arginosuccinate into two distinct molecules: arginine and fumarate. This step is crucial as it produces arginine, a direct precursor to urea in the cycle.
- Arginase: The final enzyme in the urea cycle, arginase, operates in the cytosol. It cleaves arginine, resulting in the production of one molecule of urea and ornithine. The urea produced is then ready for excretion from the body, while the ornithine is transported back into the mitochondria, ready to participate in the cycle once again.
Enzyme | Function & Location |
---|---|
Carbamoyl Phosphate Synthetase I | Located in the mitochondria. Converts ammonium and bicarbonate into carbamoyl phosphate. This reaction requires two ATP and is the rate-limiting step in the urea cycle. |
Ornithine Transcarbamoylase | Located in the mitochondria. Combines ornithine and carbamoyl phosphate to form citrulline. |
Argininosuccinate Synthetase | Located in the cytosol. Condenses citrulline with aspartate to form arginosuccinate. This reaction requires one ATP. |
Argininosuccinate Lyase | Located in the cytosol. Splits arginosuccinate into arginine and fumarate. |
Arginase | Located in the cytosol. Cleaves arginine into one molecule of urea and ornithine. The ornithine is then transported back into the mitochondria for entry back into the cycle. |
Urea Cycle Products
- Urea: At the heart of the urea cycle is the compound urea. Derived from ammonia and carbon dioxide, urea serves as a primary nitrogenous waste product. It is soluble in water, allowing it to be transported to the kidneys and subsequently excreted in the urine.
- Fumarate: As the urea cycle progresses, fumarate emerges as an intermediate byproduct. This compound doesn’t just end its journey in the urea cycle. It finds its way into the citric acid cycle, also known as the Krebs cycle, where it participates in energy production processes vital for cellular function.
- ADP and AMP: The urea cycle is energy-intensive. As it unfolds, adenosine triphosphate (ATP) is hydrolyzed, yielding adenosine diphosphate (ADP) and adenosine monophosphate (AMP). These molecules, especially ATP, are central to cellular energy transactions.
- Pi and PPi: The breakdown of ATP during the urea cycle also results in the release of inorganic phosphate (Pi) and pyrophosphate (PPi). These compounds are integral to various biochemical reactions, serving as phosphate donors or acceptors.
- Water: The urea cycle’s reactions are not dry affairs. Hydrolysis reactions, particularly those involving ATP breakdown and argininosuccinate cleavage, release water molecules, underscoring the interconnectedness of metabolic pathways.
Product | Description |
---|---|
Urea | Primary nitrogenous waste product derived from ammonia and carbon dioxide. Transported to the kidneys and excreted in the urine. |
Fumarate | An intermediate byproduct that can participate in the citric acid cycle for energy production. |
ADP and AMP | Byproducts of ATP hydrolysis, central to cellular energy transactions. |
Pi (Inorganic Phosphate) and PPi (Pyrophosphate) | Compounds released from ATP hydrolysis, serving as phosphate donors or acceptors in various biochemical reactions. |
Water | Product of hydrolysis reactions in the urea cycle, especially from ATP breakdown and argininosuccinate cleavage. |
Regulation of Urea Cycle
Regulation of the urea cycle is crucial for ensuring the efficient removal of excess nitrogen from the body. This cycle is modulated through various mechanisms to maintain the body’s nitrogen balance.
- Role of N-Acetylglutamic Acid (NAG): N-Acetylglutamic acid plays a pivotal role as an allosteric activator of carbamoyl phosphate synthetase I (CPS I), the enzyme responsible for the initial step of the urea cycle. Elevated levels of NAG enhance the activity of CPS I, thereby promoting the efficient removal of surplus ammonia through the urea cycle.
- Influence of Substrate Concentrations: The availability of substrates also dictates the regulation of the urea cycle. High concentrations of ammonia and ornithine stimulate the progression of the urea cycle, ensuring the timely removal of excess nitrogen. Conversely, elevated levels of citrulline, arginine, or urea act as a feedback mechanism, preventing excessive urea production.
- Interconnection with the Citric Acid Cycle: The urea cycle does not operate in isolation; it is intricately linked with the citric acid cycle, a central metabolic pathway. The citric acid cycle contributes fumarate, an intermediate molecule, to the urea cycle. This fumarate is generated when argininosuccinate undergoes cleavage. Once produced, fumarate can re-enter the citric acid cycle, emphasizing the interconnectedness of these metabolic pathways. This integration ensures that the body’s energy production is harmonized with the removal of waste products.
Therefore, the regulation of the urea cycle is a testament to the body’s intricate metabolic network, ensuring that nitrogen balance is maintained while seamlessly integrating with other metabolic pathways.
Regulation Mechanism | Description |
---|---|
Role of N-Acetylglutamic Acid (NAG) | N-Acetylglutamic acid acts as an allosteric activator of carbamoyl phosphate synthetase I (CPS I), the enzyme initiating the urea cycle. Elevated NAG levels enhance CPS I activity, promoting efficient removal of surplus ammonia. |
Influence of Substrate Concentrations | The availability of substrates like ammonia and ornithine stimulate the urea cycle, ensuring timely removal of excess nitrogen. Conversely, high levels of citrulline, arginine, or urea act as feedback, preventing excessive urea production. |
Interconnection with the Citric Acid Cycle | The urea cycle is intricately linked with the citric acid cycle. The citric acid cycle contributes fumarate to the urea cycle, which can re-enter the citric acid cycle, emphasizing the interconnectedness of these metabolic pathways. |
Disorders of the Urea Cycle
Genetic defects in the enzymes involved in this cycle can lead to a range of disorders, which manifest with varying symptoms and severity. These disorders are primarily characterized by an inability to effectively process ammonia, leading to its accumulation in the body.
- Ornithine Transcarbamylase (OTC) Deficiency:
- Description: This is the only X-linked recessive enzyme deficiency in the urea cycle. Due to the enzyme’s deficiency, there’s an accumulation of carbamoyl phosphate in the mitochondria. This excess carbamoyl phosphate is redirected to the pyrimidine synthesis pathway in the cytoplasm, leading to the conversion into orotic acid. This acid accumulates in the blood and urine, often manifesting as orange crystals in infants’ diapers.
- Argininosuccinate Synthetase Deficiency (Citrullinemia Type I):
- Description: An autosomal recessive disorder, it results in the buildup of citrulline and subsequently, ammonia. In infants, symptoms include seizures, lethargy, anorexia, and respiratory distress. In adults, milder symptoms such as vomiting, ataxia, and lethargy are observed.
- Carbamoyl Phosphate Synthetase I (CPS I) Deficiency:
- Description: Another autosomal recessive metabolic disorder, it typically manifests in infants as lethargy, reduced appetite, and altered body temperature and respiratory rate. Some individuals only show symptoms later in life.
- Other Urea Cycle Disorders:
- Description: Urea cycle disorders, although rare, can be severe. Typically manifesting shortly after birth, affected infants may experience vomiting, lethargy, and even coma, potentially leading to brain damage. In adults, symptoms can mimic a stroke, and liver malfunction can lead to cirrhosis and muscle mass loss. Mutations in the enzymes and transporters of the urea cycle result in these disorders. If untreated, individuals can experience hyperammonemia or the accumulation of cycle intermediates.
- Individual Disorders:
- N-Acetylglutamate Synthase (NAGS) Deficiency
- Ornithine Transcarbamoylase (OTC) Deficiency
- Citrullinemia Type I (Deficiency of argininosuccinic acid synthase)
- Argininosuccinic Aciduria (Deficiency of argininosuccinic acid lyase)
- Argininemia (Deficiency of arginase)
- Hyperornithinemia, hyperammonemia, homocitrullinuria (HHH) syndrome (Deficiency of the mitochondrial ornithine transporter)
All urea cycle defects, except for OTC deficiency, are inherited in an autosomal recessive manner. OTC deficiency, being X-linked recessive, can manifest in some females. While most urea cycle disorders result in hyperammonemia, argininemia and certain forms of argininosuccinic aciduria do not present with elevated ammonia levels.
Disorder | Description | Inheritance Pattern |
---|---|---|
Ornithine Transcarbamylase (OTC) Deficiency | The only X-linked recessive enzyme deficiency in the urea cycle leading to accumulation of carbamoyl phosphate and orotic acid in blood and urine. | X-linked recessive |
Argininosuccinate Synthetase Deficiency (Citrullinemia Type I) | An autosomal recessive disorder resulting in the buildup of citrulline and ammonia. Symptoms vary from seizures in infants to vomiting in adults. | Autosomal recessive |
Carbamoyl Phosphate Synthetase I (CPS I) Deficiency | Manifests as lethargy, reduced appetite, and altered body temperature in infants. Some individuals show symptoms later in life. | Autosomal recessive |
N-Acetylglutamate Synthase (NAGS) Deficiency | Deficiency in the NAGS enzyme. | Autosomal recessive |
Citrullinemia Type I (Deficiency of argininosuccinic acid synthase) | Deficiency leading to buildup of citrulline. | Autosomal recessive |
Argininosuccinic Aciduria (Deficiency of argininosuccinic acid lyase) | Deficiency leading to buildup of argininosuccinate. | Autosomal recessive |
Argininemia (Deficiency of arginase) | Deficiency leading to buildup of arginine. | Autosomal recessive |
Hyperornithinemia, hyperammonemia, homocitrullinuria (HHH) syndrome | Deficiency of the mitochondrial ornithine transporter. | Autosomal recessive |
Importance of Urea Cycle
- Detoxification of Ammonia: The primary function of the urea cycle is to convert highly toxic ammonia into urea, a relatively non-toxic compound. Ammonia is a byproduct of amino acid metabolism, and its accumulation in the body can be harmful. The urea cycle ensures that ammonia is safely excreted from the body in the form of urea through urine.
- Nitrogen Excretion: The urea cycle is the primary mechanism in humans for excreting excess nitrogen. This is crucial because the body constantly metabolizes proteins and amino acids, leading to the production of nitrogenous waste.
- Metabolic Interplay: The urea cycle is closely linked with other metabolic pathways, especially the citric acid cycle (or Krebs cycle). For instance, fumarate, a byproduct of the urea cycle, feeds into the citric acid cycle, illustrating the intricate metabolic interplay between different pathways.
- Amino Acid Metabolism: The urea cycle plays a role in the metabolism of several amino acids. For instance, arginine, ornithine, and citrulline, which are part of the urea cycle, are also involved in various other biochemical processes in the body.
- Regulation of Nitrogen Balance: The urea cycle helps maintain a balance between the synthesis and degradation of amino acids, ensuring that the body has an adequate supply of amino acids for protein synthesis while preventing the accumulation of excess nitrogen.
- Energy Production: Some steps in the urea cycle are linked to the production of ATP, the primary energy currency of the cell. This highlights the cycle’s role not just in detoxification but also in energy metabolism.
- Clinical Significance: Defects or deficiencies in enzymes of the urea cycle can lead to various disorders, emphasizing the cycle’s importance in health. These disorders, although rare, can lead to severe symptoms like hyperammonemia, neurological impairments, and even life-threatening conditions if not diagnosed and treated promptly.
References
- Lehninger Principles of Biochemistry by Nelson D. Cox Michael M.
- Lippincott Illustrated Reviews: Biochemistry (Lippincott Illustrated Reviews Series)
- by Denise Ferrier (Author)
- Ginguay, Antonin (2020). Reference Module in Life Sciences || Amino Acid Metabolism. , (), –. doi:10.1016/B978-0-12-819460-7.00059-1
- Engelking, Larry R. (2015). Textbook of Veterinary Physiological Chemistry || Urea Cycle (Krebs-Henseleit Ornithine Cycle). , (), 58–64. doi:10.1016/b978-0-12-391909-0.50010-4
- Barmore W, Azad F, Stone WL. Physiology, Urea Cycle. [Updated 2023 May 8]. In: StatPearls [Internet]. Treasure Island (FL): StatPearls Publishing; 2023 Jan-. Available from: https://www.ncbi.nlm.nih.gov/books/NBK513323/
- https://www.newenglandconsortium.org/urea-cycle-disorders-1
- https://www.news-medical.net/health/The-Urea-Cycle-Step-by-Step.aspx
- https://medlineplus.gov/ency/article/000372.htm
- https://byjus.com/biology/steps-of-the-urea-cycle/
- https://reactome.org/content/detail/R-HSA-70635