What is Transpiration?
- Transpiration is a fundamental physiological process in plants involving the movement of water from the plant’s interior to its exterior, primarily through the evaporation of water vapor from the aerial parts such as leaves, stems, and flowers. This process occurs passively, meaning it does not require energy expenditure from the plant.
- During transpiration, water absorbed by the plant roots moves upward through the vascular system and eventually evaporates into the atmosphere. This evaporation predominantly takes place through tiny openings on the leaf surfaces known as stomata. Transpiration serves multiple functions: it cools the plant, adjusts osmotic pressure within cells, and facilitates the mass flow of essential mineral nutrients from the soil to the plant.
- When water loss from transpiration exceeds water uptake by the roots, plants respond by closing their stomata to reduce water loss. This action, while conserving water, also slows down the uptake of nutrients and decreases carbon dioxide absorption. Consequently, the plant’s metabolic processes, including photosynthesis and growth, may be hindered.
- Transpiration, therefore, is crucial for maintaining the plant’s internal water balance and nutrient distribution, playing a key role in its overall physiological health.
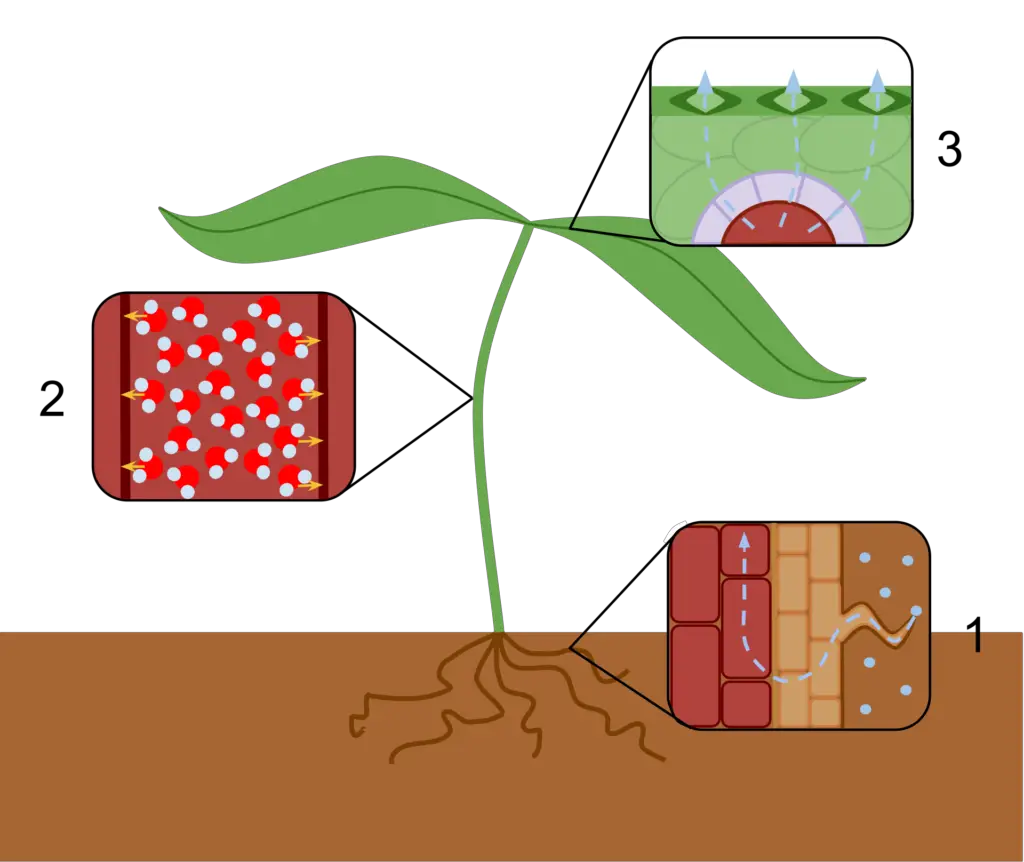
Types of Transpiration
Transpiration in plants occurs through several distinct pathways, each playing a specific role in water movement and evaporation. These pathways are classified into four primary types: stomatal, cuticular, lenticular, and bark transpiration.
- Stomatal Transpiration: This is the predominant form of transpiration, accounting for approximately 50-97% of the total transpiration in most plants. Stomatal transpiration takes place through stomata, which are small pores primarily located on the surfaces of leaves. Some stomata are also present on young stems, flowers, and fruits. The stomata facilitate the diffusion of water vapor from the internal plant tissues to the atmosphere. The internal air of the plant becomes saturated with water vapor, and as the external air is typically less saturated, water vapor diffuses outward. This process continues as long as the stomata remain open.
- Cuticular Transpiration: This type of transpiration occurs through the cuticle or epidermal cells covering the plant surfaces. Although cuticular transpiration generally contributes only 3-10% of the total transpiration in most plants, it can be more significant in herbaceous plants with thin cuticles, sometimes accounting for up to 50% of total transpiration. Cuticular transpiration happens continuously, both day and night, as the cuticle allows water vapor to escape directly from the plant’s surface.
- Lenticular or Lenticellate Transpiration: This transpiration occurs through lenticels, which are specialized structures found in the woody branches of trees. Lenticular transpiration contributes a minimal amount, about 0.1% of the total transpiration. It is a continuous process because lenticels lack closure mechanisms, allowing for ongoing water vapor exchange between the atmospheric air and the plant’s cortical tissue through intercellular spaces.
- Bark Transpiration: Bark transpiration involves the evaporation of water through the corky covering of stems. Although the rate of bark transpiration is relatively low, it is often higher than lenticular transpiration due to the larger surface area of the bark. Like cuticular and lenticular transpiration, bark transpiration occurs throughout both day and night, contributing to the plant’s overall water regulation.
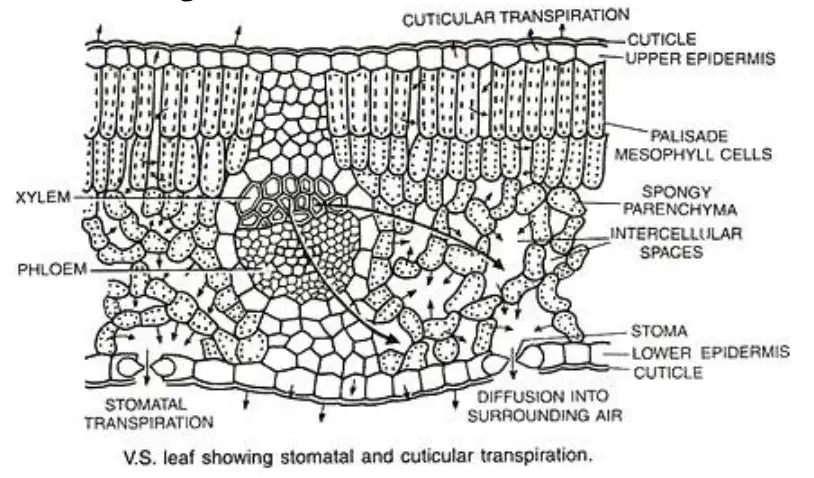
Factors affecting Transpiration
Transpiration is influenced by a variety of factors that can be classified into two primary categories: external (environmental) and internal (structural) factors.
External or Environmental Factors
- Atmospheric Humidity
- High Humidity: When the atmospheric humidity is high, the rate of transpiration decreases. This occurs because the air is more saturated with moisture, reducing the diffusion gradient for water vapor from the plant’s internal tissues to the atmosphere.
- Low Humidity: In contrast, low atmospheric humidity enhances transpiration rates as the air is less saturated with moisture, facilitating a more significant diffusion of water vapor.
- Temperature
- Increased Temperature: Higher temperatures generally increase transpiration rates. This is due to the combined effects of lower relative humidity and the enlargement of stomatal apertures. Temperatures approaching 0°C often result in closed stomata, whereas temperatures up to 30°C encourage stomatal opening and increased transpiration.
- Wind
- Gentle Wind: Mild wind enhances transpiration by removing the moisture-laden air near the plant’s surface, thereby accelerating the diffusion of water vapor from the leaves.
- Strong Wind: Excessive wind can hinder transpiration by impeding the outward movement of water vapor and potentially causing stomatal closure.
- Light
- Exposure to Light: Light increases the rate of transpiration as it promotes stomatal opening and raises leaf temperature. In darkness, stomata close, which significantly reduces transpiration.
- Available Soil Water
- Sufficient Water: Adequate soil water availability supports normal transpiration rates by ensuring efficient water absorption by the roots.
- Water Deficiency: Insufficient soil water leads to reduced transpiration, as the plant struggles to maintain water balance, impacting overall water uptake and distribution.
- CO2 Concentration
- Increased CO2: Higher atmospheric or internal CO2 concentrations lead to stomatal closure, thereby reducing transpiration rates.
Factor | Description |
---|---|
Atmospheric Humidity | – High Humidity: Decreases transpiration due to reduced water vapor diffusion. – Low Humidity: Increases transpiration by facilitating vapor diffusion. |
Temperature | – Higher temperatures increase transpiration by lowering relative humidity and enlarging stomatal apertures. – Near freezing temperatures may close stomata. |
Wind | – Gentle Wind: Increases transpiration by removing moisture-laden air. – Strong Wind: May decrease transpiration by hindering water vapor diffusion and closing stomata. |
Light | – Light increases transpiration by promoting stomatal opening and increasing leaf temperature. – Darkness reduces transpiration due to stomatal closure. |
Available Soil Water | – Sufficient Water: Supports normal transpiration. – Deficiency: Reduces transpiration due to impaired water uptake. |
CO2 Concentration | – Increased CO2: Causes stomatal closure, reducing transpiration. |
Internal or Structural Factors
- Internal Water Conditions
- Water Availability: Internal water conditions are crucial for transpiration. A shortage of water within the plant reduces the transpiration rate. Prolonged high transpiration rates can also create an internal water deficit if water absorption does not match the rate of loss.
- Leaf Area
- Leaf Size: Larger leaf areas generally increase the magnitude of transpiration. However, smaller plants may transpire at a higher rate per unit area compared to larger plants.
- Leaf Structure
- Cuticle Thickness: The thickness of the cuticle can influence transpiration rates. A thicker cuticle generally reduces transpiration.
- Epidermal Hairs: The density and thickness of epidermal hairs on the leaf surface can trap a layer of air, reducing transpiration by creating a barrier to water vapor loss.
- Stomata
- Stomatal Characteristics: The number, distribution, size, and periodic opening of stomata affect transpiration rates. Xerophytes, for example, often have stomata that are open at night and closed during the day to conserve water, resulting in lower daytime transpiration.
- Leaf Orientation, Size, and Shape
- Leaf Position: The angle of leaf orientation relative to sunlight affects transpiration. Leaves perpendicular to light absorb more heat, increasing transpiration, while parallel leaves experience less heat absorption.
- Leaf Modifications: Reduced leaf size and specialized structures such as spines or thorns lower transpiration rates by minimizing surface area and exposure.
- Root-Shoot Ratio
- Ratio Impact: An increased root-shoot ratio typically enhances transpiration efficiency. Plants like sorghum often transpire more per unit leaf surface compared to plants like corn.
- Disease
- Effect of Disease: Diseased plants may exhibit higher transpiration rates compared to healthy plants, often due to compromised water regulation mechanisms.
Factor | Description |
---|---|
Internal Water Conditions | – Adequate Water: Essential for maintaining transpiration rates. – Water Deficit: Reduces transpiration due to internal water shortage. |
Leaf Area | – Larger Leaves: Generally increase transpiration magnitude. – Smaller Plants: May transpire more per unit area compared to larger plants. |
Leaf Structure | – Cuticle Thickness: Thicker cuticles reduce transpiration. – Epidermal Hairs: Increase thickness of the stationary air layer, reducing transpiration. |
Stomata | – Number, Size, Distribution: Affect transpiration rates. – Xerophytes: Stomata often closed during the day to conserve water. |
Leaf Orientation, Size, Shape | – Leaf Position: Perpendicular leaves absorb more heat and increase transpiration. – Reduced Leaf Size: Decreases transpiration rates. |
Root-Shoot Ratio | – Higher Ratio: Typically increases transpiration efficiency. – Sorghum vs. Corn: Sorghum often has higher transpiration per unit leaf surface. |
Disease | – Diseased Plants: May have higher transpiration rates due to compromised water regulation mechanisms. |
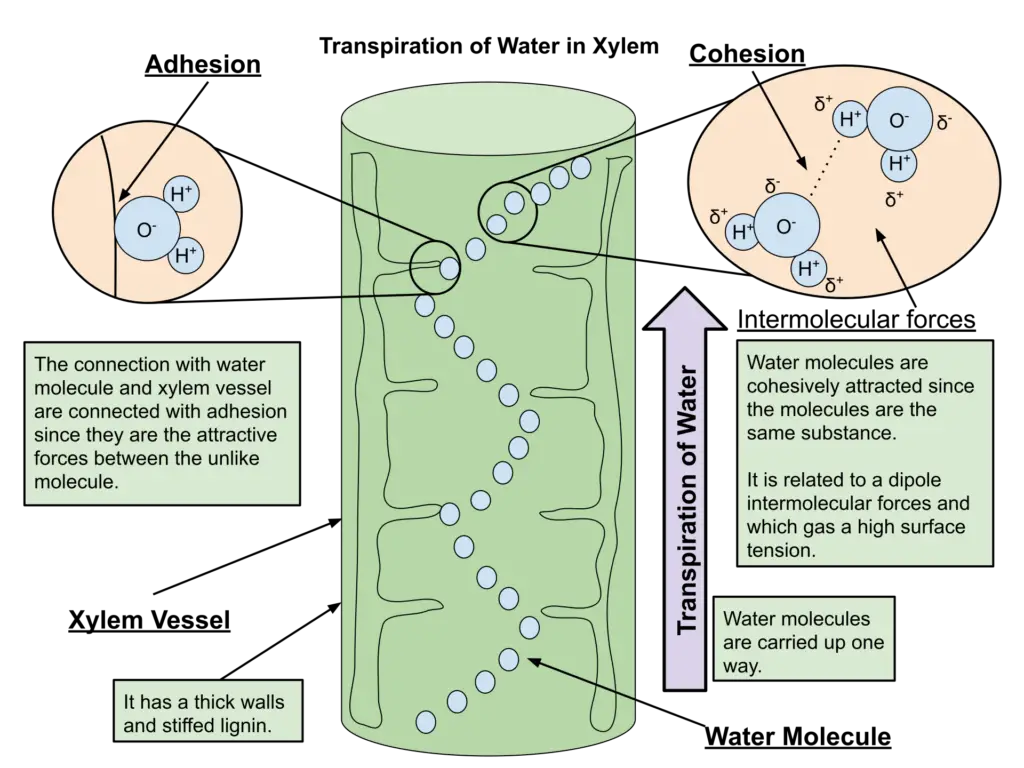
Significance of Transpiration
Transpiration is a crucial physiological process in plants with several important functions. Its significance extends to various aspects of plant health and functionality, which can be categorized into the following key areas:
- Water and Mineral Absorption
- Water Uptake: Transpiration facilitates the absorption of water from the soil by creating a negative pressure within the plant’s vascular system. This pressure draws water up from the roots to the leaves, where it evaporates.
- Mineral Transport: The water flow driven by transpiration also aids in the movement of mineral salts dissolved in the soil solution, ensuring that essential nutrients reach different parts of the plant.
- Sap Ascent and Nutrient Translocation
- Suction Force: The process of transpiration generates a suction force that assists in the ascent of sap through the xylem vessels. This force is critical for the efficient translocation of water and dissolved minerals from the roots to the leaves and other plant tissues.
- Cell Turgidity and Osmosis
- Maintaining Turgidity: Transpiration helps in maintaining the optimum turgidity of plant cells. Adequate turgor pressure is essential for cell structure and growth, as it supports the plant’s overall rigidity and shape.
- Osmotic Balance: The removal of water through transpiration also plays a role in proper osmosis within the cell sap, which is vital for nutrient balance and cellular function.
- Excess Water Removal
- Water Regulation: Transpiration assists in the removal of excess water from the plant body. This process helps prevent waterlogging and maintains internal water balance.
- Cooling Effect
- Temperature Regulation: The evaporation of water from plant surfaces has a cooling effect, reducing leaf temperature. This cooling effect is important for preventing heat stress and maintaining optimal metabolic processes within the plant.
- Stomatal Function and Photosynthesis
- Stomatal Dynamics: Transpiration influences the opening and closing of stomata, which are crucial for gas exchange. This regulation impacts photosynthesis and respiration by controlling the availability of carbon dioxide and oxygen.
Adaptation to prevent water loss by excess of transpiration
Plants in arid or water-scarce environments have developed various adaptations to minimize water loss through excessive transpiration. These adaptations can be categorized into structural, morphological, and physiological modifications.
- Thick and Reflective Cuticle
- Function: In some xerophytic plants, the leaves are covered with a thick and reflective cuticle. This cuticle acts as a barrier to water loss by reducing the permeability of the leaf surface. Additionally, the reflective nature of the cuticle helps in reflecting sunlight, thereby reducing leaf temperature and further minimizing water loss.
- Sunken Stomata and Multiple Epidermal Layers
- Structure: Plants like Nerium have adapted by developing sunken stomata and multiple epidermal layers.
- Function: Sunken stomata are recessed into the leaf surface, which reduces exposure to the external environment and decreases water vapor loss. Multiple layers of epidermal cells provide additional insulation and protection against excessive transpiration.
- Modification of Leaves into Phylloclades
- Adaptation: In extreme xerophytes such as cacti, leaves are absent and stems are modified into structures called phylloclades.
- Function: Phylloclades perform photosynthesis while significantly reducing the surface area available for transpiration. This adaptation helps in conserving water by minimizing the loss through evaporation.
- Reduction of Leaves to Scaly Branches
- Adaptation: Plants like Asparagus have evolved to reduce their leaves to scaly branches known as cladodes.
- Function: These cladodes are adapted to reduce water loss while still performing essential functions such as photosynthesis. The reduction in leaf surface area minimizes the amount of water lost through transpiration.
- Well-Developed Deep Root System
- Structure: Xerophytic plants often have extensive, deep root systems.
- Function: These deep roots allow the plant to access water from deeper soil layers that are less likely to be depleted. This adaptation ensures that the plant can obtain the necessary water with minimal loss through transpiration.
What is Guttation?
Guttation is a physiological process in plants where water is exuded in liquid form from the tips or edges of leaves. This phenomenon is distinct from transpiration, which primarily involves the loss of water vapor through stomata. Guttation occurs through specialized structures known as hydathodes.
Hydathodes: Structure and Function
- Location: Hydathodes are situated at the leaf margins, typically where the veins terminate.
- Structure: Each hydathode comprises a permanent water pore, a small underlying cavity, and a loose tissue called epithem.
- Epithem: This tissue is closely associated with the vascular elements of the veins and plays a crucial role in water regulation.
Mechanism of Guttation
- Root Pressure: Guttation is facilitated by root pressure, a condition where water is pushed upward from the roots into the xylem vessels of the veins.
- Water Movement: Under increased root pressure, water is transferred from the xylem into the epithem.
- Exudation: Once the cavity within the hydathode is filled with water, it exudes as liquid drops through the water pore.
Conditions Favoring Guttation
- Environmental Factors: Guttation is most commonly observed in plants growing in warm, moist soils.
- Timing: This process typically occurs early in the morning when root pressure is high and transpiration rates are relatively low.
Composition and Impact
- Composition of Drops: The liquid drops consist not only of water but also of various dissolved inorganic and organic substances.
- Residue Formation: Upon drying, these droplets leave behind a residue of salts and organic compounds on the leaf margins.
Examples of Plants Exhibiting Guttation
- Common Examples: Guttation can be observed in plants such as garden nasturtium, tomato, strawberry, and Colocasia.
- Observation: In these plants, liquid droplets ooze from the uninjured margins of leaves where the main veins terminate.
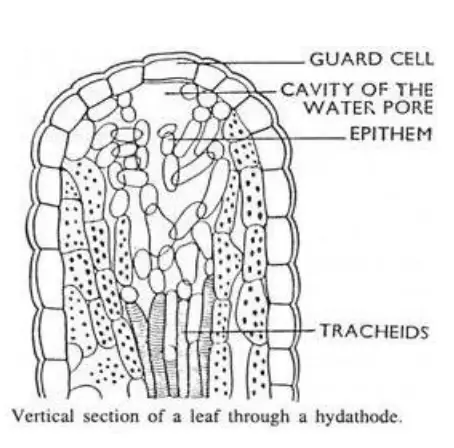
What is Plant anti-transpirants?
Anti-transpirants are substances applied to plants to reduce the rate of transpiration, thereby mitigating water loss and preventing wilting. This approach can be crucial in optimizing crop yields, particularly in environments where high transpiration rates could lead to reduced plant health and productivity. Anti-transpirants are classified into two main categories based on their mechanisms of action: metabolic inhibitors and film-forming agents.
1. Metabolic Inhibitors:
- Phenylmercuric Acetate (PMA):
- PMA is a chemical compound that impedes the normal metabolic processes in plant cells, leading to a reduction in stomatal opening. This decreased stomatal aperture limits water loss through transpiration.
- Abscisic Acid (ABA):
- ABA is a plant hormone known for its role in regulating stomatal closure. By promoting the closing of stomata, ABA effectively reduces water vapor loss and helps in conserving water within the plant.
- Aspirin:
- Aspirin, primarily known as a medicinal compound, also functions as an anti-transpirant by influencing stomatal behavior. Its application can reduce the rate of transpiration by affecting the plant’s internal signaling pathways.
2. Film-Forming Anti-Transpirants:
- Colorless Plastics:
- These materials form a thin, transparent layer on the plant’s surface. They restrict the loss of water vapor while still allowing the exchange of gases such as oxygen (O₂) and carbon dioxide (CO₂), which are essential for photosynthesis.
- Silicon Emulsions:
- Silicon-based anti-transpirants create a protective coating on plant surfaces. This coating prevents excessive water loss without significantly obstructing gas exchange, thus balancing water conservation with photosynthetic needs.
Application and Benefits:
- Anti-transpirants can be particularly advantageous in crop production by helping to maintain optimal water levels in plants, even under conditions of high transpiration. This, in turn, can lead to improved plant health and higher yields.
- Examples of film-forming anti-transpirants include silicon oil and various waxes, which provide an effective barrier against water loss while permitting necessary gas exchanges.
Stomatal Apparatus
The stomatal apparatus, a critical component of plant physiology, comprises structures essential for gas exchange and transpiration. Understanding the stomatal apparatus involves examining its morphology, function, and regulatory mechanisms.
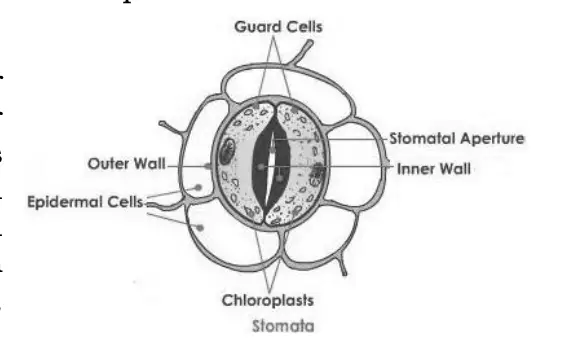
1. Structure of Stomata
- Stomatal Pores: Stomata are microscopic pores located predominantly on the epidermis of leaves and other aerial plant parts. These pores measure approximately 10-14 µm in length and 3-12 µm in width. Their density varies significantly, ranging from 1,000 to 60,000 stomata per square centimeter of leaf surface.
- Guard Cells: Each stomatal pore is flanked by a pair of specialized cells known as guard cells. These cells are crucial for regulating the size of the stomatal aperture. They contain chloroplasts, which are involved in photosynthesis, and small vacuoles. The guard cells are linked to adjacent epidermal cells via plasmodesmata, facilitating communication.
- Cell Structure: The guard cells exhibit a unique structure. Their walls are thicker on the inner side and thinner on the outer side. This structural asymmetry plays a vital role in their function. The guard cells’ shape is generally kidney-like, and their curved surfaces aid in the opening and closing of the stomatal pore.
2. Function of Stomata
- Gas Exchange: Stomata are primarily responsible for the exchange of gases between the plant and its environment. During daylight, when the stomata are open, carbon dioxide (CO₂) enters the leaf for photosynthesis, while oxygen (O₂) exits.
- Transpiration: Another critical function of stomata is transpiration, the process of water vapor loss from the plant. This loss of water creates a suction pull, aiding in the uptake of water from the roots. Additionally, transpiration contributes to cooling the plant.
- Stomatal Dynamics: The opening and closing of stomata are controlled by changes in turgor pressure within the guard cells. When the turgor pressure is high, the guard cells swell, causing the stomatal pore to open. Conversely, when turgor pressure decreases, the guard cells shrink, leading to pore closure. This dynamic movement is crucial for regulating gas exchange and water loss.
3. Stomatal Index and Frequency
- Stomatal Frequency: The number of stomata per unit area of leaf surface is referred to as stomatal frequency. This frequency varies among plant species and environmental conditions. Xerophytes, which are adapted to dry environments, typically have a higher number of stomata compared to mesophytes, which thrive in more moderate conditions.
- Variation in Leaf Types: In isobilateral leaves, which are characteristic of monocots, the number of stomata is roughly equal on both the upper (adaxial) and lower (abaxial) surfaces. In contrast, dorsiventral leaves, common in dicots, generally have a higher density of stomata on the lower surface compared to the upper surface.
4. Stomatal Dynamics
- Mechanism of Action: The regulation of stomatal movement involves complex interactions between guard cell turgor pressure and the structural properties of the cell walls. The guard cells’ ability to change shape is facilitated by microfibrils within their cell walls, which are oriented to support the expansion and contraction required for stomatal function.
- Opening and Closing: During the opening of stomata, increased turgor pressure causes the guard cells to swell, creating a pore. During closure, the process reverses as turgor pressure decreases, leading to the narrowing of the pore.
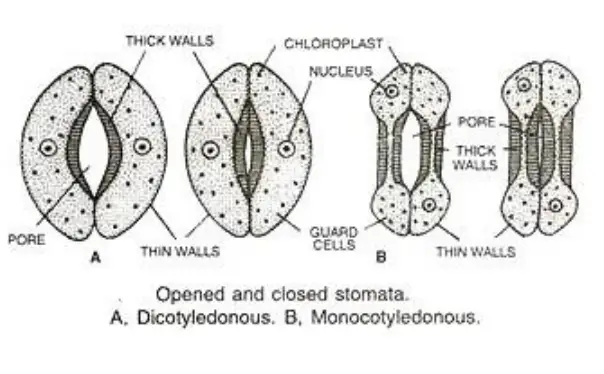
Types of Stomata
Stomata are microscopic pores found on the surfaces of leaves and stems, facilitating gas exchange between the plant and its environment. They play a crucial role in processes such as photosynthesis and transpiration. Stomatal types are classified based on the arrangement of the epidermal cells adjacent to the guard cells. This classification varies between dicots and monocots.
Stomatal Types in Dicots
- Anomocytic Type (Ranunculaceous Type)
- Description: In this type, the guard cells are encircled by epidermal cells that are morphologically indistinguishable from the surrounding cells.
- Characteristics:
- No distinct differentiation between the guard cells and surrounding epidermal cells.
- The arrangement is more or less uniform and lacks specialized subsidiary cells.
- Anisocytic Type (Cruciferous Type)
- Description: Here, the guard cells are surrounded by three subsidiary cells of varying sizes.
- Characteristics:
- The subsidiary cells are uneven in size, creating an asymmetrical arrangement.
- This type is typically found in the Brassicaceae family (e.g., mustard).
- Paracytic Type (Rubiaceous Type)
- Description: Each guard cell in this type is accompanied by one or more subsidiary cells that are aligned parallel to the long axis of the epidermal cell.
- Characteristics:
- Subsidiary cells run parallel to the guard cells, creating a linear arrangement.
- Common in the Rubiaceae family (e.g., coffee plants).
- Diacytic Type (Caryophyllaceous Type)
- Description: The stoma in this type is surrounded by two subsidiary cells positioned perpendicular to the guard cells.
- Characteristics:
- The two subsidiary cells are oriented at right angles to the guard cells.
- Found in the Caryophyllaceae family (e.g., carnations).
- Actinocytic Type
- Description: In this type, the stomata are encircled by a ring of radiating cells.
- Characteristics:
- The surrounding cells radiate outward from the guard cells, forming a circular pattern.
- This arrangement is less common but can be seen in some plant species.
Stomatal Types in Monocots
In monocots, stomatal classification is based on the number of subsidiary cells surrounding the guard cells. These types include:
- Types with Single Subsidiary Cell
- Description: Each guard cell is surrounded by a single subsidiary cell.
- Types with Multiple Subsidiary Cells
- Description: Guard cells are encircled by multiple subsidiary cells, varying in number.
Stomatal mechanism
The mechanism of stomatal function involves complex biochemical and physiological processes that regulate the opening and closing of stomata. This regulation is crucial for balancing gas exchange and water conservation in plants. The stomatal mechanism can be understood through three primary theories, each offering insights into how guard cells control the stomatal aperture.
1. Hypothesis of Guard Cell Photosynthesis
- Mechanism Overview: This hypothesis posits that the chloroplasts within guard cells are responsible for their movement. During daylight, chloroplasts in the guard cells conduct photosynthesis, producing sugars.
- Osmotic Changes: The accumulation of sugars increases the osmotic concentration within the guard cells, causing them to draw water from surrounding cells. This influx of water leads to cell expansion, resulting in the opening of the stomatal pore.
- Limitations: Although this theory highlights the role of photosynthesis in stomatal function, it has been found that the contribution of guard cell chloroplasts to photosynthesis is relatively minimal. Therefore, other mechanisms are also crucial for understanding stomatal dynamics.
2. Classical Starch Hydrolysis Theory
- Daytime Mechanism:
- Photosynthesis and CO2 Levels: In the presence of light, guard cells utilize CO2 for photosynthesis. This process lowers CO2 concentration within the guard cells and raises the pH, making the environment more alkaline.
- Starch Conversion: The alkaline pH activates the enzyme phosphorylase, which converts starch into glucose 1-phosphate. This compound is then transformed into glucose and phosphoric acid.
- Osmotic Effects: The increase in glucose raises the osmotic concentration of guard cells. Consequently, water is absorbed from adjacent cells, causing guard cells to swell and the stomatal pore to open.
- Nighttime Mechanism:
- Reduced Photosynthesis: In the absence of light, CO2 levels rise within the guard cells, and the pH drops, making the environment more acidic.
- Starch Formation: The decrease in pH leads to the conversion of glucose back into starch, mediated by phosphorylase.
- Osmotic Effects: The reduction in glucose lowers the osmotic concentration, leading to water loss from the guard cells. This loss of turgidity causes the stomatal pore to close.
3. Malate or K+ Ion Pump Hypothesis (Modern Theory)
- Daytime Mechanism:
- Photosynthesis and CO2 Utilization: During the day, guard cells perform photosynthesis, consuming CO2 and raising the pH, making the environment more alkaline.
- Starch to Malate Conversion: Starch is converted into malic acid, which dissociates into malate (an anion) and hydrogen ions (H+).
- Ion Exchange: H+ ions are actively transported out of the guard cells, while potassium ions (K+) are taken up from surrounding cells via the H+/K+ ATPase pump. This process requires ATP energy.
- Osmotic Effects: The increase in malate and K+ ions raises the osmotic concentration, causing water to flow into the guard cells (endosmosis). The guard cells become turgid, opening the stomatal pore.
- Nighttime Mechanism:
- Cease in Photosynthesis: Photosynthesis stops in the dark, causing CO2 levels to rise and pH to fall, making the environment more acidic.
- ABA Hormone Release: The plant hormone abscisic acid (ABA) is released, which inhibits the active outflux of H+ ions and promotes the re-entry of K+ ions into surrounding cells.
- Starch Formation: Malate combines with H+ to form malic acid, which is then converted into starch.
- Osmotic Effects: The decrease in osmotic concentration leads to water exiting the guard cells (exosmosis), causing them to become flaccid and the stomatal pore to close.
Changes occurs During opening and closing of stomata
The opening and closing of stomata are regulated through a series of biochemical and physiological changes within the guard cells. These changes are crucial for managing gas exchange and water loss in plants. The mechanisms underlying these processes differ between day and night, reflecting the plant’s adaptive responses to environmental conditions.
Opening of Stomata
- Daytime Activation:
- Photosynthesis: During daylight, guard cells engage in photosynthesis, which consumes CO2 and lowers its concentration within the cells.
- pH Increase: The reduction in CO2 results in an alkaline environment within the guard cells, raising the pH to less than 7.
- Conversion of Starch:
- Malic Acid Formation: The increased pH triggers the conversion of starch into malic acid. This malic acid dissociates into malate (an anion) and hydrogen ions (H+).
- Ion Dynamics: The accumulation of H+ ions initially makes the environment acidic. Subsequently, H+ ions are actively transported out of the guard cells, and potassium ions (K+) are taken up from the surrounding cells through the H+/K+ ATPase pump, which requires ATP.
- Osmotic Changes:
- Increased Osmotic Pressure: The influx of K+ and the presence of organic acids elevate the osmotic concentration within the guard cells. This lowers the water potential inside the guard cells.
- Endosmosis: Water from adjacent subsidiary cells diffuses into the guard cells due to the osmotic gradient. As a result, the guard cells become turgid, causing the stomatal pore to open.
Closing of Stomata
- Nighttime Deactivation:
- Cease in Photosynthesis: In the absence of light, photosynthesis halts, leading to a rise in CO2 concentration within the guard cells.
- pH Decrease: The increase in CO2 lowers the pH of the guard cells to greater than 5, making the environment more acidic.
- Role of Abscisic Acid (ABA):
- ABA Release: The plant releases the growth-inhibiting hormone abscisic acid (ABA), which impedes the active transport of H+ ions out of the guard cells.
- Effect on K+ Ions: ABA induces the H+/K+ ATPase pump to expel K+ ions from the guard cells back into the surrounding cells, which requires energy expenditure.
- Osmotic Changes:
- Starch Formation: Malate combines with H+ ions to form malic acid, which is then converted back into starch. This reduces the osmotic concentration within the guard cells.
- Exosmosis: The decrease in osmotic pressure causes water to move out of the guard cells (exosmosis) into the subsidiary cells. Consequently, the guard cells become flaccid, leading to the closure of the stomatal pore.
Factors Affecting Stomatal Movements
Stomatal movements, which regulate gas exchange and water loss in plants, are influenced by a variety of environmental and internal factors. These factors can be broadly categorized into external (environmental) and internal (endogenous) influences. Understanding these factors is crucial for comprehending how plants adapt to varying conditions.
External Factors
- Light Intensity
- Mechanism: Stomata generally open in response to light and close in darkness. The intensity of light required for this response can be minimal; for instance, in tobacco plants, light as low as 250 foot-candles is sufficient. Both red and blue wavelengths are effective, with blue light being slightly more potent.
- Exceptions: In Crassulacean Acid Metabolism (CAM) plants, such as Agave, Opuntia, and Pineapple, stomata remain closed during the day and open only at night. This adaptation helps conserve water in arid environments.
- Temperature
- Mechanism: High temperatures (around 38°C to 40°C) can cause stomata to open even in the absence of light, while extremely low temperatures (around 0°C) typically result in stomatal closure, even under continuous light conditions. Generally, temperatures above 30°C can inhibit stomatal opening in many plant species.
- Atmospheric Humidity
- Mechanism: In humid conditions, stomata tend to remain open for extended periods, facilitating gas exchange. Conversely, in dry environments, stomata often close to minimize water loss.
- Water Availability
- Mechanism: Water stress, resulting from insufficient water availability relative to transpiration rates, leads to stomatal closure. This response is mediated by abscisic acid (ABA) and an increase in the differential pressure of the epidermal cells.
- Mechanical Shock
- Mechanism: Physical damage or shock to the plant can result in stomatal closure as a protective response.
- CO2 Concentration
- Mechanism: Stomata typically open in response to low CO2 concentrations and close when CO2 levels are high. The sensitivity to CO2 is localized to the inner side of the guard cells. In environments with high internal CO2 levels or in dark, CO2-free conditions, stomata remain closed. However, they will open in light to utilize internal CO2.
Internal Factors
- Oxygen
- Mechanism: Adequate oxygen is required for stomatal opening, as it is involved in the metabolic processes that drive this movement.
- pH
- Mechanism: An increase in the pH of the guard cells’ environment typically facilitates stomatal opening, while a decrease in pH induces closure.
- Growth Hormones
- Mechanism: Cytokinins play a crucial role in stomatal opening. In contrast, abscisic acid (ABA) is involved in stomatal closure, helping the plant respond to stress conditions.
- Minerals
- Mechanism: The availability of potassium ions (K+) from adjacent epidermal cells is essential for stomatal opening. Additionally, other minerals, such as phosphorus (P), nitrogen (N), magnesium (Mg), and calcium (Ca), also play significant roles in regulating stomatal movements.
What is Root pressure?
Root pressure is a physiological phenomenon observed in vascular plants, characterized by the osmotic pressure within the root system that propels sap upward through the plant’s stem to the leaves. This process plays a role in the overall water transport system of plants, although it is not the sole mechanism responsible for moving water to the tops of the tallest trees.
Mechanism of Root Pressure
- Formation of Root Pressure
- Osmotic Gradient: Root pressure arises from the active transport of mineral nutrients and ions into the root xylem. This accumulation of solutes lowers the water potential within the xylem cells of the roots.
- Water Movement: As a result of this lowered water potential, water from the surrounding soil diffuses into the root xylem through osmosis. The influx of water creates a positive pressure within the xylem vessels.
- Impact on Sap Movement
- Pressure Build-Up: The accumulation of water generates transverse osmotic pressure, which exerts force on the walls of the xylem vessels, pushing the sap upward through the plant stem.
- Guttation: In some cases, particularly at night or under conditions of low transpiration, the root pressure can be high enough to cause guttation. This process involves the exudation of droplets of xylem sap from the edges or tips of leaves.
- Experimental Observation
- Measurement: To study root pressure, researchers often excise the shoot of a plant near the soil level. The xylem sap will then exude from the cut stem due to the root pressure. Attaching a pressure gauge to the cut stem allows for direct measurement of root pressure.
Limitations of Root Pressure
- Height Limitation
- Maximum Reach: Root pressure is effective in pushing water only a limited distance up the plant stem. The maximum root pressure observed in some plants can raise water to approximately 6.87 meters.
- Tall Trees: This mechanism alone cannot account for the water transport in the tallest trees, which can exceed 100 meters in height. Therefore, root pressure is not sufficient to explain the water movement to the top of these trees.
- Role in Water Transport
- Transpirational Pull: During periods of high transpiration, the xylem sap is typically under tension rather than pressure due to the transpirational pull, which predominantly drives the movement of water upward through the plant.
Ascent of sap
The ascent of sap refers to the process through which water absorbed by the roots of a plant travels upward through the stem to reach the aerial parts, including the leaves and flowers. This movement is essential for the plant’s physiological processes and can be divided into two primary areas of study: the path and mechanism of sap ascent.
Path of Ascent of Sap
- Xylem as the Conduit:
- The ascent of sap occurs predominantly through the xylem vessels. Xylem is a type of vascular tissue responsible for transporting water and nutrients from the roots to the rest of the plant.
- The xylem vessels are specialized structures that facilitate the upward movement of water due to their hollow, tubular nature.
- Ringing Experiment:
- A common method to study the path of sap ascent is the ringing experiment. In this procedure, a ring of bark, including all tissues outside the vascular cambium, is removed from a stem.
- The stem is then placed in water, and observations are made regarding the condition of the leaves above the ringed area. The leaves remain fresh and green, indicating that water continues to reach them through the xylem, despite the removal of the bark. This demonstrates that xylem tissues are crucial for water transport.
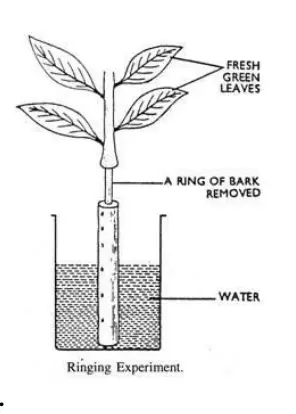
Mechanism of Ascent of Sap
The ascent of sap, which involves the upward movement of water from the roots to the leaves and other aerial parts of the plant, is a critical physiological process. Understanding the mechanisms that facilitate this movement provides insight into how plants maintain their internal water balance. The mechanism of sap ascent can be explained through several theories, each highlighting different aspects of this complex process.
1. Vital Theories
Vital theories propose that the upward movement of sap is influenced by the activities of living cells within the plant. Two prominent theories in this category are:
- Relay Pump Theory:
- This theory, proposed by Godlewski (1884), suggests that the ascent of sap results from the pumping activity of living cells within the xylem, specifically the xylem parenchyma cells.
- According to this theory, the cells in the medullary rays, which are also living, alter their osmotic pressure in a way that facilitates the movement of sap upward through the xylem vessels.
- Pulsation Theory:
- Introduced by Bose (1923), this theory posits that the upward movement of water is driven by the pulsatory activity of living cells in the innermost cortical layer, just outside the endodermis.
- The pulsations of these cells generate a pressure that helps push the sap upward through the plant’s vascular system.
2. Root Pressure Theory
- Concept:
- Proposed by Priestly (1916), the root pressure theory explains sap ascent through the generation of pressure in the roots.
- Water absorbed by root hairs accumulates in the cortical cells of the roots, causing them to become turgid. The elastic walls of these cells then exert pressure on the fluid contents, forcing them into the xylem vessels.
- As the cortical cells lose water and become flaccid, they absorb more water and become turgid again, creating an intermittent pumping action. This action generates a considerable pressure that aids in the ascent of sap.
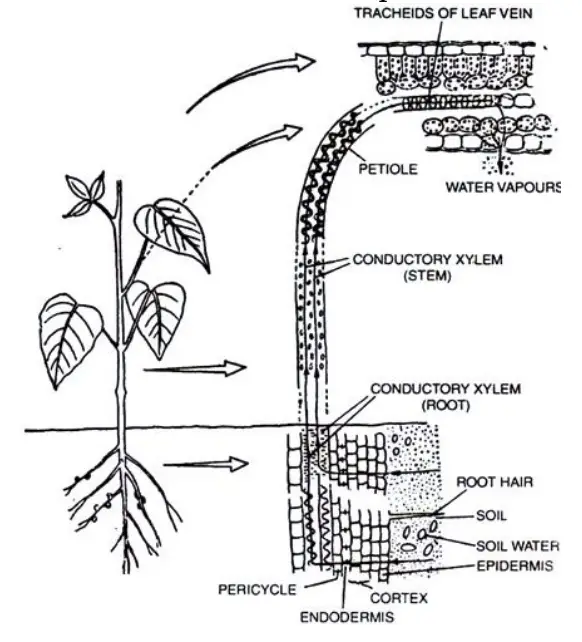
3. Physical Force Theories
Physical force theories emphasize the role of physical principles rather than the activities of living cells. The most widely accepted theory in this category is the Cohesion-Tension Theory (also known as the Cohesion-Tension and Transpiration Pull Theory), proposed by Dixon and Joly (1894) and refined by Dixon in 1914.
- Continuous Water Column:
- The theory asserts that a continuous column of water exists from the roots through the stem and into the leaves. This column is maintained within the tracheary elements of the xylem, such as vessels and tracheids.
- The water column remains uninterrupted due to the extensive network of tracheary elements, which allows for the passage of water even if some elements are blocked. The forces of transpiration provide the necessary energy and pull to prevent the water column from falling under gravity.
- Cohesion and Adhesion Forces:
- Cohesion: Water molecules are attracted to each other through hydrogen bonds, resulting in a strong mutual attraction known as cohesion. This force allows the water column to withstand significant tension or pull.
- Adhesion: Water molecules are also attracted to the walls of the xylem vessels, a force known as adhesion. This attraction helps maintain the water column’s continuity and facilitates capillary action within the xylem vessels.
- Development of Tension or Transpiration Pull:
- The transpiration process involves the loss of water vapor from the intercellular spaces of mesophyll cells in the leaves. This loss creates a negative osmotic potential, leading to a reduction in water potential.
- The resultant water deficiency in the mesophyll cells causes them to draw water from the xylem vessels. This collective water deficiency across all leaves generates a pulling force or tension that is transmitted through the water column in the xylem, effectively lifting water from the roots to the leaves.
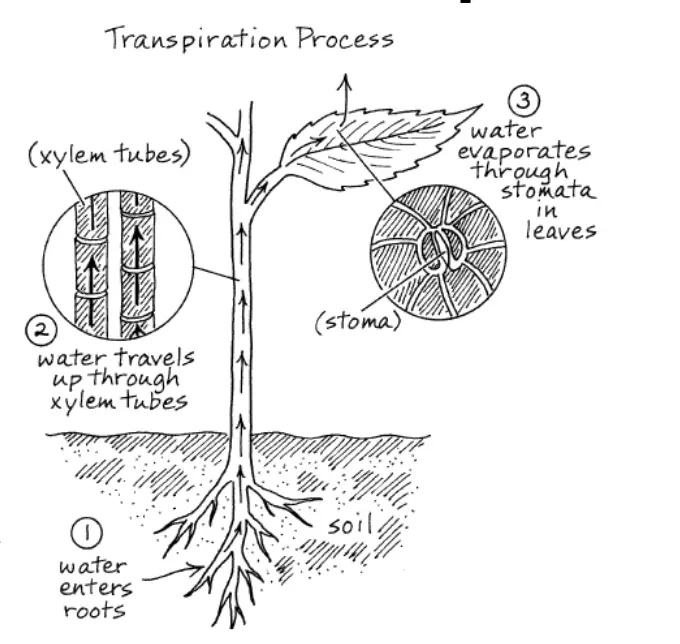
Food translocation in plants
Food translocation in plants refers to the movement of organic materials, primarily in the form of sucrose, from the sites of production to various parts of the plant where they are either utilized or stored. This process is fundamental to plant growth and survival and is primarily facilitated by the phloem tissue. Below is a detailed exploration of the key aspects of food translocation in plants:
- Phloem: The Conduit for Food Transport:
- The phloem is the primary tissue responsible for the conduction of food in plants. Unlike xylem, which conducts water unidirectionally from roots to leaves, phloem can transport food materials in multiple directions depending on the plant’s needs. This bidirectional movement ensures that all parts of the plant receive the necessary nutrients.
- Source and Sink Relationship:
- The translocation of food occurs between two key points: the source and the sink. The source refers to the parts of the plant that produce or store food, such as leaves (where photosynthesis occurs) or storage organs like tubers. The sink refers to the parts of the plant that require or store these nutrients, such as roots, shoots, fruits, and developing buds.
- For example, during the germination of a potato tuber, the tuber acts as the source, supplying nutrients to the developing buds, which act as the sink. Conversely, in early spring, the roots may act as a source, providing nutrients to emerging buds.
- Seasonal and Developmental Variations:
- The direction of food translocation can vary based on the plant’s developmental stage or seasonal conditions. The source and sink roles may interchange depending on the plant’s physiological needs, ensuring that nutrients are directed where they are most needed at any given time.
- Sucrose as the Primary Transport Molecule:
- In plants, sucrose is the primary form of carbohydrate transported through the phloem. Sucrose is a non-reducing sugar, making it chemically inert and stable during transport. This stability is crucial for efficient translocation.
- Pressure Flow Hypothesis:
- The most widely accepted theory explaining food translocation in plants is the Pressure Flow or Mass Flow Hypothesis, proposed by Munch. According to this hypothesis, the movement of sucrose between the source and sink follows a gradient of turgor pressure (T.P.).
- Phloem Loading at the Source: At the source, sucrose is actively loaded into the phloem sieve tubes. This loading increases the osmotic pressure within the sieve cells, causing water to move from the nearby xylem into the phloem, thereby increasing turgor pressure.
- Phloem Unloading at the Sink: At the sink, sucrose is actively unloaded from the phloem, leading to a decrease in osmotic pressure. This decrease allows water to exit the phloem and re-enter the xylem, lowering turgor pressure in the sieve cells at the sink.
- This difference in turgor pressure between the source and sink creates a pressure gradient that drives the flow of sucrose from areas of high pressure (source) to areas of low pressure (sink).
- Role of Metabolic Energy:
- Phloem conduction is not a passive process; it requires metabolic energy. The active loading and unloading of sucrose at the source and sink, respectively, are facilitated by carrier molecules that utilize energy to transport sucrose against concentration gradients.
- Experimental Evidence:
- The Pressure Flow Hypothesis was experimentally demonstrated by Munch through the Bimodal experiment, which provided empirical support for the theory. Modern research continues to validate the active nature of phloem transport, emphasizing its reliance on metabolic processes.
Factors affecting translocation
Translocation in plants, the movement of organic nutrients from sources (where they are produced or stored) to sinks (where they are utilized or stored), is a complex process influenced by various environmental and physiological factors. Understanding these factors is crucial for comprehending how plants manage the distribution of essential nutrients. Below are the key factors that affect translocation:
- Temperature:
- Temperature plays a significant role in the rate of translocation. The optimal temperature for translocation typically ranges between 20-30°C. As temperature increases within this range, the rate of translocation also increases. However, if the temperature exceeds the upper limit, the rate begins to decline. Conversely, at lower temperatures, the process slows down significantly, affecting the efficiency of nutrient distribution.
- Light Intensity:
- Light intensity directly influences the movement of assimilates, particularly those from the leaves. Higher light intensity generally enhances the translocation of food, particularly directing it more towards the roots than the shoots. This occurs because increased light boosts photosynthetic activity, leading to a greater production of assimilates. On the other hand, lower light intensity can inhibit the growth of both roots and shoots, thereby reducing the rate of translocation.
- Hormones:
- Plant hormones, particularly cytokinins, have a notable impact on translocation. Cytokinins stimulate the movement of water-soluble nitrogen compounds, facilitating their transport through the phloem. This hormonal regulation ensures that nutrients are efficiently allocated to areas of the plant where they are most needed, such as growing tissues.
- Oxygen:
- Oxygen is essential for the process of phloem loading, where nutrients are actively transferred from mesophyll cells into the phloem. This process requires energy in the form of ATP, which is generated through cellular respiration—a process dependent on oxygen. Without sufficient oxygen, the efficiency of phloem loading decreases, thereby slowing down the entire translocation process.
- Minerals:
- Certain minerals play critical roles in facilitating translocation. For instance, boron is essential for the translocation of sugars, particularly sucrose, within the plant. Phosphorus also contributes to the movement of solutes, aiding in the overall translocation process. The presence of these minerals in adequate amounts is necessary to maintain effective nutrient transport.
- Water Availability:
- Water content within plant cells significantly affects the translocation of photosynthates. Adequate water is necessary to maintain turgor pressure within the phloem, which drives the movement of nutrients from sources to sinks. A shortage of water can disrupt this process, leading to reduced translocation efficiency.
- Metabolic Inhibitors:
- Metabolic inhibitors, such as iodoacetate, hydrogen cyanide (HCN), and carbon monoxide, can adversely affect translocation. These inhibitors interfere with the process of respiration by hindering ATP production, which is crucial for both phloem loading and unloading. As a result, the overall process of translocation is significantly impaired when these inhibitors are present.
What is Root hair?
Root hairs are specialized extensions of the root’s outer epidermal cells, crucial for nutrient and water absorption. Their structure is intricately designed to maximize their functional efficiency.
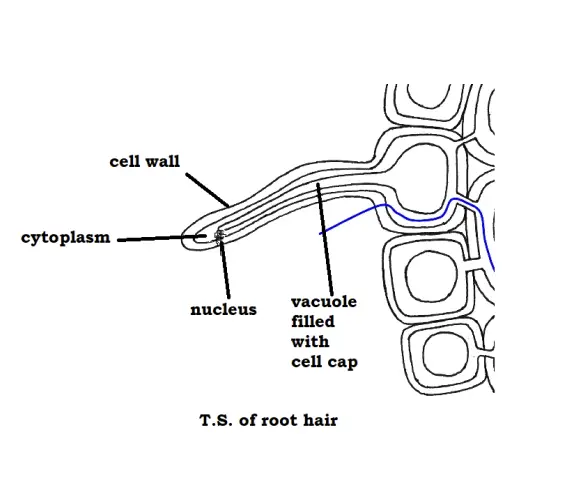
1. Basic Structure
- Prolongation of Epidermal Cells: Root hairs are elongated extensions of the epidermal cells of the root’s proliferative layer. These structures emerge from the root’s surface and significantly increase its absorptive area.
- Cell Wall Composition: The cell wall of a root hair is composed of two distinct layers:
- Outer Layer: This layer consists of hydrophilic pectic compounds, which are effective in absorbing water. The hydrophilic nature of these compounds facilitates the uptake of moisture from the soil.
- Inner Layer: Beneath the outer layer lies a cellulose-based layer. Cellulose is a polysaccharide that enhances the cell wall’s structural integrity while contributing to water absorption.
- Plasma Membrane: Inside the cell wall, the plasma membrane encloses the cell’s cytoplasm. This membrane is selectively permeable, regulating the movement of ions and molecules in and out of the root hair.
- Cytoplasm and Vacuole: The cytoplasm houses a large vacuole filled with cell sap. The osmotic pressure within the vacuole is typically higher than the surrounding soil solution, driving water uptake into the root hair.
- Nucleus: The nucleus is located within the cytoplasm, directing cellular functions and regulating metabolic processes.
2. Functional Aspects
- Selective Permeability: The plasma membrane of the root hair cells is selectively permeable. This selectivity allows the root hair to control the intake of nutrients and water while excluding harmful substances.
- Water Absorption: The outer cell wall’s hydrophilic nature and the osmotic pressure created by the vacuole together enhance the root hair’s ability to absorb water from the soil efficiently.
3. Symplast and Apoplast Pathways
- Symplast Pathway:
- Definition: The symplast refers to the network of living cell protoplasts connected through plasmodesmata.
- Function: This pathway allows for the direct transfer of water, ions, and small molecules between cells, facilitating communication and nutrient distribution throughout the plant.
- Apoplast Pathway:
- Definition: The apoplast consists of the non-living components of the plant, including cell walls, intercellular spaces, and xylem vessels.
- Function: This pathway facilitates the movement of water and dissolved substances through the cell walls and intercellular spaces. It operates independently of the cytoplasmic streaming found in the symplast.
Difference between transpiration and guttation
Aspect | Transpiration | Guttation |
---|---|---|
Water Form | Water is lost in the form of water vapor, which is a gaseous state of water. This vapor diffuses from the internal air spaces of the plant into the atmosphere. | Water is lost in the form of liquid droplets, which are exuded from the plant’s surface. These droplets appear on the edges or tips of leaves. |
Entry Points | Occurs through various structures including stomata (small pores on leaf surfaces), cuticle (waxy layer covering the leaf), and lenticels (small openings in stems). | Occurs specifically through water stomata or hydathodes, which are specialized structures found mostly on leaf margins or tips. |
Volume of Water Lost | A significant volume of water is lost through transpiration. This process involves the continuous movement of water from the soil through the plant and into the atmosphere. | Comparatively, a smaller volume of water is lost through guttation. It is typically a localized process that does not involve the entire plant. |
Composition of Water | The water vapor lost through transpiration is generally pure, with minimal dissolved substances. It primarily consists of water molecules. | Gutted water contains dissolved substances such as salts and sugars. This is due to the accumulation of these solutes in the plant’s xylem sap. |
Effect on Leaves | Transpiration has a cooling effect on leaves, as the evaporation of water vapor absorbs heat energy from the leaf surface, reducing its temperature. | Guttation does not have a cooling effect on leaves. Since it involves the exudation of liquid water, it does not influence leaf temperature significantly. |
Occurrence | Transpiration occurs in nearly all terrestrial plants, functioning as a universal mechanism for water regulation and cooling. | Guttation occurs specifically in certain herbaceous plants that are growing in moist environments or under conditions of high soil water availability. |
Timing | Transpiration typically occurs during daylight hours when light and higher temperatures promote the opening of stomata and enhance water vapor loss. | Guttation generally takes place during the cooler, more humid conditions of early morning or at night when transpiration rates are low. |
Environmental Conditions | Transpiration is favored by dry conditions, where lower humidity and higher temperatures increase the rate of water vapor loss from the plant. | Guttation is favored by humid conditions where high soil moisture leads to excess water in the plant, causing the release of droplets through hydathodes. |
Water Loss Mechanism | The process involves the diffusion of water vapor from the internal leaf spaces to the external atmosphere, driven by the concentration gradient and environmental factors. | Guttation involves the exudation of liquid water directly from plant tissues through hydathodes, which is a result of root pressure overcoming atmospheric pressure. |
Regulation | The rate of transpiration is primarily regulated by the opening and closing of stomata, which are controlled by environmental factors such as light, temperature, and humidity. | Guttation is regulated by the availability of water in the plant and external humidity levels, with root pressure playing a significant role in driving the process. |
Impact on Plant | Excessive transpiration can lead to wilting if the rate of water loss exceeds the plant’s ability to absorb water from the soil. | Guttation does not result in wilting as it usually occurs under conditions of adequate soil moisture and is a minor process compared to transpiration. |
Advantages of transpiration
Transpiration, the process through which plants lose water vapor from their aerial parts, plays a crucial role in the overall physiology and functioning of plants. Below are the key advantages of transpiration:
- Water Absorption Enhancement:
- Transpiration creates a negative pressure within the leaf, which contributes to the upward movement of water from the roots through the xylem vessels. This tension, often referred to as the “transpiration pull,” facilitates the continuous absorption of water from the soil by the roots.
- Ascent of Sap:
- The tension created by transpiration is vital for the ascent of sap. As water evaporates from the leaf surfaces, it pulls more water upward from the roots through the xylem. This process ensures that water, along with essential nutrients and minerals absorbed by the roots, is effectively transported to various parts of the plant.
- Nutrient Distribution:
- Transpiration is integral to the movement of water and dissolved minerals from the roots to the other parts of the plant. This upward movement ensures that all parts of the plant receive the necessary nutrients required for growth and development.
- Cooling Effect:
- The evaporation of water from the leaves during transpiration has a cooling effect, which helps in regulating the temperature of the plant. This cooling mechanism is particularly important in preventing heat injury to the leaves during periods of high temperature and intense sunlight. The cooling also extends to the surrounding air, contributing to the microclimate around the plant.
- Protection from Heat Injury:
- By cooling the leaves, transpiration plays a defensive role against potential heat damage. This is especially crucial in conditions where the plant is exposed to high temperatures, as it helps maintain the structural and functional integrity of the leaves.
FAQ
In the context of the water cycle, transpiration is the process through which water vapor is released from plants into the atmosphere. This release of water vapor contributes to the overall movement of water in the hydrological cycle. As plants transpire, they add moisture to the air, which can eventually condense to form clouds and, subsequently, lead to precipitation. Transpiration, combined with evaporation from other surfaces, plays a key role in regulating weather patterns and climate.
Transpiration is a crucial biological process in which water is absorbed by plant roots from the soil, transported through the plant's vascular system, and released into the atmosphere as water vapor. This process occurs primarily through small pores called stomata on the surface of leaves, but it can also occur through other plant parts such as stems. Transpiration helps in maintaining the plant's water balance and contributes to nutrient uptake and cooling.
Transpiration in plants refers to the movement of water from the soil through the plant to the atmosphere. This process begins with water being absorbed by plant roots from the soil. The water then travels up through the plant's vascular system, primarily the xylem vessels, and exits the plant through tiny openings in the leaves called stomata. During this process, water evaporates into the atmosphere, which is crucial for the plant's internal water regulation, cooling, and nutrient transport.
Transpiration refers to the physiological process by which plants lose water vapor to the atmosphere. This term encompasses both the movement of water through the plant and its subsequent evaporation from aerial parts. The process of transpiration helps plants manage water loss, maintain internal pressure, and facilitate the uptake of essential minerals from the soil. It is an integral part of the plant's ability to regulate its internal environment and contribute to the broader water cycle.
Transpiration works through a sequence of steps involving water uptake, movement, and release. It begins with the absorption of water from the soil by plant roots, which then travels through the plant's vascular system (xylem). As water reaches the leaves, it moves into the stomata, small pores on the leaf surface. Once in the stomata, water evaporates into the atmosphere. This loss of water vapor creates a negative pressure within the plant, which helps pull more water from the roots through the plant. Transpiration is influenced by factors such as temperature, humidity, and wind, which affect the rate of evaporation.
Transpiration occurs primarily through the stomata, which are small pores located on the surface of leaves. However, it can also occur through other parts of the plant such as stems and flowers, where similar openings called lenticels are present. The majority of transpiration takes place in the leaves because they have a large surface area and are equipped with numerous stomata to facilitate gas exchange and water vapor release. The overall process is essential for maintaining the plant’s water balance and supporting nutrient transport.
If the context is about water movement and vapor release in plants, the step shown is transpiration. Transpiration involves the release of water vapor from plants into the atmosphere. On the other hand, translocation refers to the movement of nutrients and sugars through the plant’s phloem, while transcription and translation are processes related to genetic expression and protein synthesis in cells.
In biology, transpiration is a physiological process in plants where water is absorbed from the soil, transported through the plant's vascular system, and released as water vapor into the atmosphere. This process is vital for several reasons: it aids in cooling the plant, facilitates nutrient uptake, maintains water balance, and contributes to the water cycle. Transpiration also influences plant growth and overall health, making it a key focus of study in plant biology and ecology.
The hydrological cycle, also known as the water cycle, facilitates the movement of water between different spheres (atmosphere, lithosphere, hydrosphere, and biosphere) through the process of transpiration. In this cycle, water is transferred from the soil and plant surfaces into the atmosphere as vapor. This vapor eventually condenses to form clouds and returns to the Earth's surface as precipitation, continuing the cycle. Transpiration is an essential component of this cycle, contributing to the regulation of global water distribution and climate.
If location W is associated with the release of water vapor from plants into the atmosphere, then the process occurring at location W is transpiration. However, if location W is related to water changes in the atmosphere or Earth's surface, the process could also be precipitation (falling water), evaporation (water turning into vapor), or condensation (vapor turning into liquid). The specific process at location W depends on the context provided.
Transpiration occurs when plants lose water vapor through their stomata and other surface openings. This process is most active during daylight hours when the stomata are open for gas exchange, allowing water vapor to escape into the atmosphere. Factors influencing transpiration include environmental conditions such as temperature, humidity, wind speed, and light intensity. High temperatures and low humidity typically increase transpiration rates, while high humidity and cooler temperatures can reduce it.
Humidity has a significant impact on transpiration rates. High humidity reduces the rate of transpiration because the concentration gradient between the water vapor inside the leaf and the surrounding air is smaller, resulting in less driving force for water to evaporate. Conversely, low humidity increases the rate of transpiration because the air is drier, creating a larger concentration gradient and enhancing the movement of water vapor from the plant to the atmosphere. Changes in humidity levels can therefore influence a plant’s water loss and overall water balance.
Transpiration is important for several reasons. It helps regulate the plant’s internal temperature by cooling the plant through the evaporation of water. This process also aids in the uptake and transport of essential nutrients and minerals from the soil to various parts of the plant. Additionally, transpiration contributes to the water cycle by adding water vapor to the atmosphere, which is crucial for cloud formation and precipitation. By maintaining water balance and facilitating nutrient transport, transpiration supports plant health and growth, and impacts broader ecological and climatic systems.
The definition of transpiration is the physiological process by which plants absorb water from the soil through their roots, transport it through their vascular system, and release it into the atmosphere as water vapor through small pores called stomata. This process is vital for maintaining the plant’s internal water balance, cooling, and nutrient uptake. Transpiration also plays a role in the water cycle by contributing to atmospheric moisture and influencing weather patterns.
In science, transpiration is defined as the movement of water within plants from the soil, through the plant’s vascular system, and into the atmosphere as water vapor. This process is essential for various physiological functions in plants, including water regulation, nutrient transport, and cooling. Transpiration is also a key component of the water cycle, affecting global water distribution and climate. Scientific studies of transpiration help understand plant physiology, ecology, and the broader impacts on environmental systems.
During transpiration, water is first absorbed by plant roots from the soil. This water travels through the plant’s vascular system (xylem vessels) and reaches the leaves. In the leaves, water moves into the stomata, small pores on the leaf surface, and evaporates into the atmosphere as water vapor. This evaporation creates a negative pressure within the plant, which helps draw more water from the roots through the plant. Transpiration also helps in cooling the plant, maintaining its water balance, and supporting the uptake of essential nutrients.
The process of transpiration involves several steps: first, water is absorbed from the soil by plant roots, which then moves up through the plant's vascular system, primarily through the xylem vessels. As water reaches the leaves, it travels to the stomata, tiny openings on the leaf surface. Through these stomata, water vapor evaporates into the atmosphere. This evaporation creates a negative pressure within the plant, which helps pull more water from the roots through the plant. The overall process of transpiration is influenced by environmental factors such as temperature, humidity, and wind.
"Transpiration" is not a physical object but rather a process, so the term "a transpiration" is not accurate. It refers to the complex physiological process in which water is absorbed by plant roots from the soil, transported through the plant, and released as water vapor into the atmosphere. This process is essential for plant health, water regulation, and contributes to the water cycle. The term encompasses the entire series of actions involved in the movement and release of water within and from the plant.
Transpiration is caused by the evaporation of water from the surface of plant leaves and other aerial parts. This evaporation is driven by environmental factors such as temperature, which increases the rate of water vapor loss, and wind, which can enhance the removal of water vapor from the leaf surface. Low humidity levels create a steep concentration gradient between the inside of the leaf and the surrounding air, promoting increased transpiration. Additionally, the process of transpiration is facilitated by the opening of stomata, which allows water vapor to escape into the atmosphere.
- https://byjus.com/biology/transpiration/
- https://www.slideshare.net/slideshow/transpiration-and-factors-affecting-transpiration/238632381
- https://www.brainkart.com/article/Transpiration-in-Plants_39726/
- https://www.sciencelearn.org.nz/images/3034-transpiration
- https://www.geeksforgeeks.org/transpiration/
- https://en.wikipedia.org/wiki/Transpiration#:~:text=Transpiration%20is%20the%20process%20of,mass%20flow%20of%20mineral%20nutrients.