What Are Telomeres (telomere)?
- Telomeres are specialized structures located at the ends of linear eukaryotic chromosomes. Composed of repetitive nucleotide sequences, telomeres serve as protective caps, preventing the degradation of chromosomal DNA and ensuring stability during cell division. These regions do not code for proteins but instead play a critical role in maintaining genomic integrity by safeguarding the terminal DNA sequences from being mistaken for double-strand breaks by DNA repair mechanisms.
- The term “telomere” was introduced by Hermann Joseph Muller, who, along with Barbara McClintock, independently identified the unique properties of chromosomal ends. McClintock observed that natural chromosomal ends were stable and resisted fusion, contrasting with the sticky behavior of broken chromosomes. Telomeres are primarily composed of tandem repeat sequences rich in guanine nucleotides. In humans, the repeat sequence is 5’-TTAGGG-3’, extending over a region that varies between 4 and 11 kilobases. The complementary sequence, 3’-AATCCC-5’, completes the structure.
- A complex of proteins, collectively known as the shelterin complex, binds to telomeric DNA. This assembly includes proteins such as TRF1, TRF2, POT1, TIN2, TPP1, RAP1, and Tankyrase. Shelterin proteins coordinate with telomerase and other enzymes to regulate telomere maintenance and functionality. Telomerase, a ribonucleoprotein enzyme, counteracts the progressive shortening of telomeres during cell division by synthesizing additional repeat sequences. This activity is critical in certain cell types, such as germline cells, stem cells, and cancer cells, where indefinite division is necessary.
- The discovery of telomeres and their mechanisms of protection was a significant milestone in molecular biology. Elizabeth Blackburn, Joseph G. Gall, and Alexey Olovnikov made foundational contributions to understanding telomere structure and the “end replication problem,” which refers to the inability of conventional DNA polymerases to fully replicate the ends of linear chromosomes. This phenomenon explains why telomeres shorten with each cell cycle in somatic cells, eventually triggering replicative senescence when critically short.
- Further studies revealed that telomerase plays a pivotal role in immortalized cells, such as those found in certain cancers, by preventing telomere shortening and enabling continuous cell proliferation. For their groundbreaking work on telomeres and telomerase, Elizabeth Blackburn, Carol Greider, and Jack Szostak were awarded the 2009 Nobel Prize in Physiology or Medicine.
- Telomeres exemplify the intricate regulatory systems that sustain cellular life and genetic stability. Their study has profound implications for understanding aging, cancer biology, and potential therapeutic interventions targeting telomere maintenance.
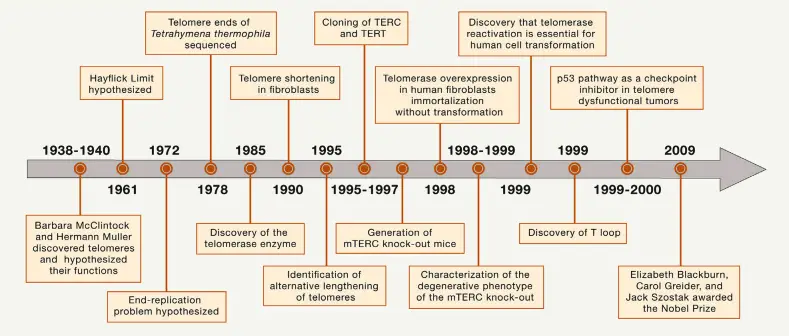
Telomeres Definition
Telomeres are repetitive nucleotide sequences located at the ends of eukaryotic chromosomes that protect DNA from degradation and prevent chromosomes from fusing together. They play a vital role in maintaining genomic stability and shorten with each cell division, a process linked to aging and cellular lifespan.
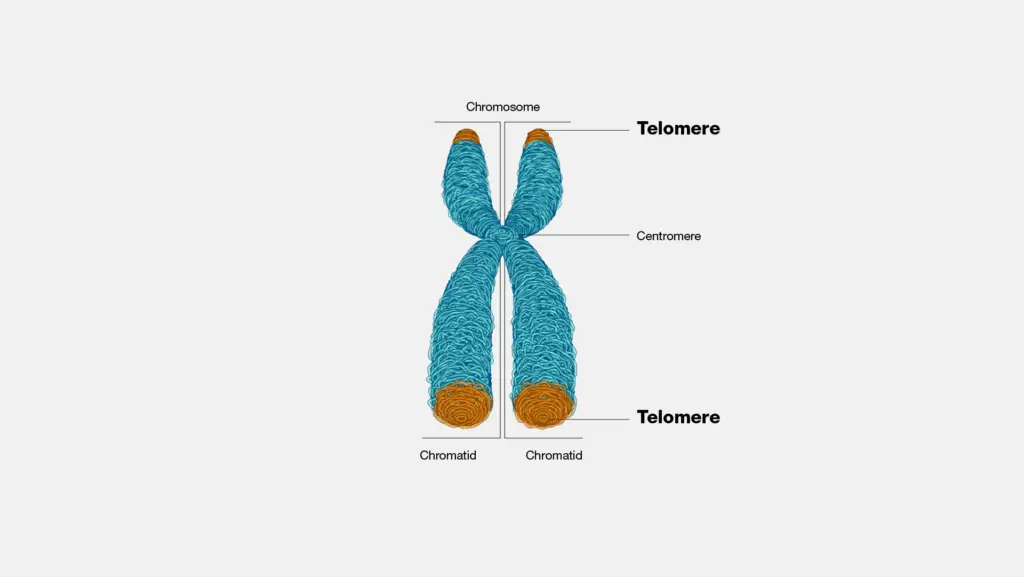
Telomere Structure
Telomeres are the repetitive DNA sequences located at the ends of chromosomes in eukaryotic cells. These structures serve to protect the chromosomal DNA from degradation and prevent fusion with other chromosomes. Their composition and arrangement vary slightly between species, but certain key features remain conserved across the eukaryotic kingdom.
- Composition: Telomeres are made up of short, non-coding nucleotide sequences that repeat multiple times. In humans, the repeating unit is 5′-TTAGGG-3′, a hexameric pattern that is consistent throughout most vertebrates. The sequence can vary among species, such as 5′-TTTAGGG-3′ in Arabidopsis thaliana.
- Structure: At the end of each telomere is a single-stranded 3’ overhang that is rich in guanine nucleotides. This overhang, which ranges from 50 to 500 nucleotides in length in mammals, plays a key role in forming the telomere’s protective structure. The 3’ overhang folds back into the chromosome, forming a T-loop, a protective structure that sequesters the chromosome end, preventing it from being exposed to DNA repair machinery. This loop formation helps protect the chromosome from degradation and prevents it from being mistaken for a break in the DNA, which could lead to inappropriate repair events, such as chromosome fusion.
- Proteins Involved: Telomeres are closely associated with a set of six key proteins that form the Shelterin complex. This protein complex is essential for maintaining the telomere’s integrity and function:
- TERF1 (TRF1): This protein regulates telomere length.
- TERF2 (TRF2): Stabilizes the T-loop structure, which is crucial for protecting the telomere ends.
- POT1: Binds to the single-stranded overhang, inhibiting DNA damage responses at the telomere ends.
- ACD (TPP1): Assists in the recruitment of POT1 to the telomere.
- TIN2: Tethers the various Shelterin components together, stabilizing the complex.
- RAP1: Works alongside other Shelterin proteins to regulate telomere length and prevent unwanted DNA repair responses.
- Length and Variation: The length of telomeres can vary greatly among species. In humans, telomeres can range from 5,000 to 15,000 base pairs long. This length can decrease over time due to cellular division, particularly in somatic cells, where telomeres shorten with each replication. In contrast, germline cells, stem cells, and certain cancerous cells possess mechanisms (such as telomerase activity) that prevent significant telomere shortening, allowing these cells to divide indefinitely.
- Species-Specific Differences: While the repeating sequence of telomeres is largely conserved in vertebrates, other species exhibit slight variations. For example, in plants like Arabidopsis thaliana, the telomere sequence is TTTAGGG, while in some prokaryotes with linear chromosomes, telomeres can form hairpin loops or bind to proteins, though these structures are different from those seen in eukaryotes.
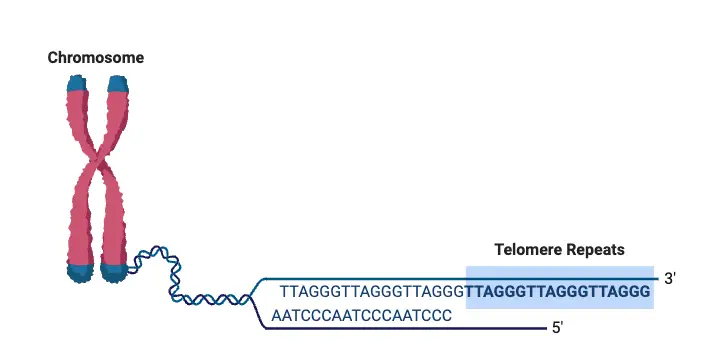
Shelterin Complex
The Shelterin complex is a group of proteins critical for the protection and maintenance of telomeres. These proteins collectively shield telomeric DNA from damage, regulate telomere length, and prevent inappropriate DNA repair responses at chromosome ends. The Shelterin complex is essential for telomere function across the cell cycle and ensures that the chromosome ends are properly capped and protected.
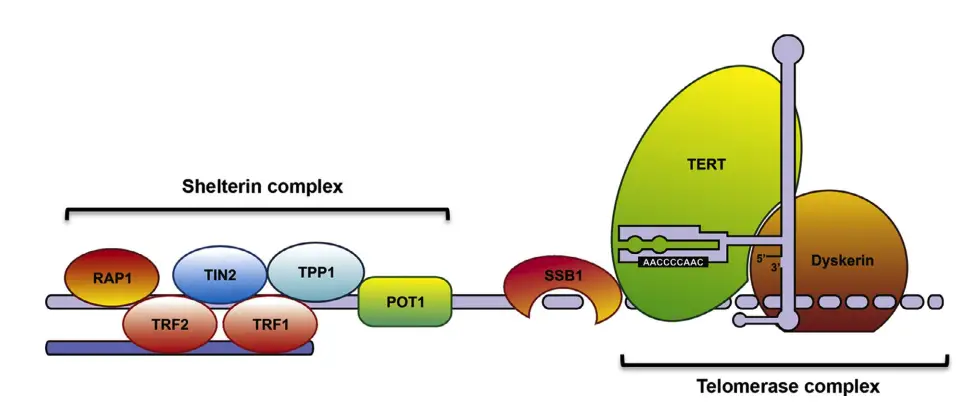
- Components of Shelterin: The Shelterin complex consists of six main proteins:
- TRF1 and TRF2 (Telomeric Repeat Binding Factors): These two proteins bind to the double-stranded DNA of the telomere and are essential for telomere protection and length regulation. TRF1 and TRF2 both recognize the ‘TTAGGG’ repeat sequence at the telomere ends.
- POT1 (Protection of Telomeres): POT1 specifically binds to the single-stranded TTAGGG repeats at the 3’ overhang or in the t-loop configuration. It plays a role in preventing DNA damage responses at the telomere ends.
- TIN2 (TRF1-Interacting Nuclear Protein 2): TIN2 acts as a central connector within the Shelterin complex. It bridges TRF1, TRF2, and TPP1, linking both the double-stranded and single-stranded components of the telomere.
- TPP1 (Telomerase Recruitment Factor): TPP1 helps to recruit and regulate POT1’s binding to the telomere. It also interacts with telomerase through its N-terminus, potentially influencing telomerase activity and telomere elongation.
- RAP1 (Repressor/Activator Protein 1): RAP1 is involved in regulating telomere length and interacts with other Shelterin components to maintain telomere structure.
- Mechanism of Shelterin: Shelterin protects the telomeres from being recognized as broken DNA. In mammalian cells, the DNA damage response is typically triggered by the kinases ATM and ATR. ATM responds to double-stranded breaks, while ATR is activated by single-stranded DNA. Without Shelterin, the telomere ends could be mistakenly identified as damaged, leading to inappropriate DNA repair or cell cycle arrest.
- ATM and ATR Signaling: Shelterin proteins prevent the activation of these kinases at the telomeres. For example, TRF2 plays a critical role in shielding the telomere from ATM activation. It forms a higher-order DNA structure at the telomere, hiding the DNA ends from ATM or Mre11, a protein that mediates the ATM signaling pathway.
- If TRF2 is removed or inhibited, it leads to the activation of DNA damage responses, marked by the accumulation of 53BP1, MDC1, and g-H2AX at the telomeres, termed telomere dysfunction-induced foci (TIFs). These signals activate the p53/p21 pathway, which can induce cell cycle arrest, senescence, or apoptosis.
- The POT1 protein, which works with TPP1, helps to inhibit ATR signaling. If both forms of POT1 are deleted, TIF formation occurs at all telomeres, triggering cell cycle arrest.
- ATM and ATR Signaling: Shelterin proteins prevent the activation of these kinases at the telomeres. For example, TRF2 plays a critical role in shielding the telomere from ATM activation. It forms a higher-order DNA structure at the telomere, hiding the DNA ends from ATM or Mre11, a protein that mediates the ATM signaling pathway.
- Function of Shelterin: The main role of Shelterin is to prevent telomeres from being misinterpreted as broken DNA. This protects cells from activating the DNA damage repair machinery, which could result in chromosome fusion or instability. The Shelterin complex also regulates the length of telomeres and recruits telomerase, which adds new DNA repeats to the telomeres, counteracting their natural shortening during cell division.
- Other Proteins Associated with Telomeres: While Shelterin is the primary protein complex protecting telomeres, there are additional factors involved in telomere maintenance. These include Ku70/80, XPF/XRCC1, Apollo, Mre11, RAD51D, RecQ helicases, and others. These proteins, although less abundant, play essential roles in the overall maintenance of telomere function and structure.
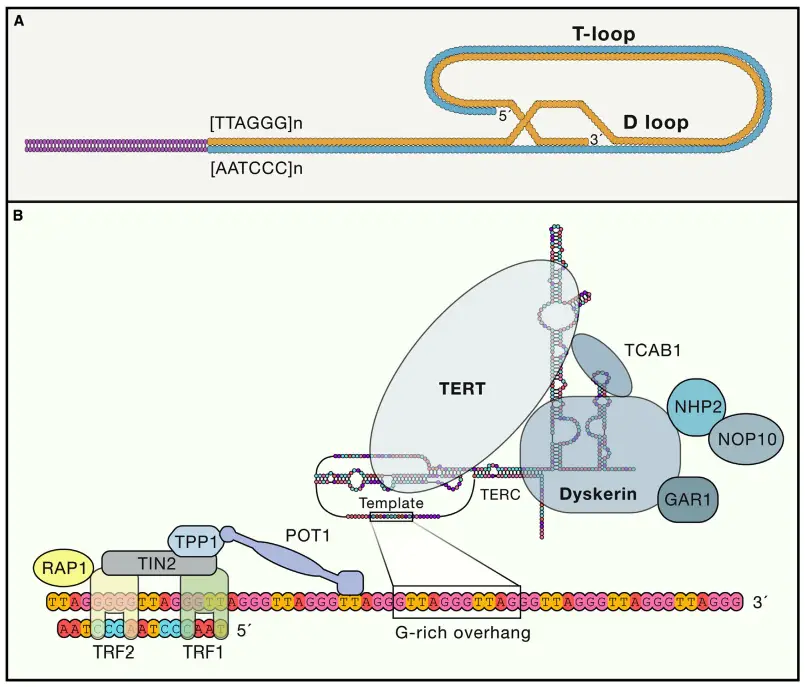
Telomere Length Measurements
Measuring telomere length is critical in understanding cellular aging, cancer progression, and genetic stability. Several techniques have been developed to quantify telomere length, each with unique strengths and limitations.
- Telomere Restriction Fragment Analysis (TRF)
- First method developed to measure the average telomere length in a cell population.
- Involves digesting genomic DNA with restriction enzymes that do not cut telomere sequences.
- The resulting fragments are analyzed via gel electrophoresis and Southern blotting, using a telomere-specific probe.
- TRF provides an estimate of median telomere length but lacks high resolution.
- Requires large DNA amounts, limiting its use mainly to cell lines and broad research questions.
- Quantitative Fluorescence In Situ Hybridization (Q-FISH)
- Uses fluorescently labeled peptide nucleic acid (PNA) probes, specific to telomere DNA repeats.
- The fluorescence signal is measured against standards of known telomere length in metaphase spreads.
- Q-FISH offers high-resolution data and is ideal for studying specific chromosome ends.
- Useful for detecting very short telomeres (down to 0.5 kb) and chromosome fusion events.
- Though well-established, Q-FISH requires specialized equipment and is labor-intensive.
- Flow Cytometry or Flow FISH
- Combines hybridization of fluorescently labeled probes with flow cytometry to measure telomere length in individual cells in suspension.
- Provides accurate measurements of median telomere length in a cell population.
- Allows the study of specific cell subsets by using cell surface markers.
- Flow FISH is efficient for high-throughput analysis and is commonly used to measure telomere length in peripheral blood cells.
- The method has been particularly valuable in clinical investigations, such as in age-related studies and telomerase deficiency cases.
- Single Telomere Length Analysis (STELA)
- A highly precise method that uses PCR amplification to measure the size distribution of single telomeres.
- Requires gel electrophoresis and Southern blotting to analyze the PCR products.
- Suitable for rare, short telomeres and single-cell analysis, making it the most precise method available.
- Labor-intensive and technically demanding, STELA is limited to chromosome ends for which specific primers can be designed.
- Quantitative PCR (Q-PCR)
- Estimates average telomere length by measuring the ratio of telomere DNA amplicons to single-copy gene amplicons.
- Offers a quicker alternative to other methods but requires further validation for accuracy.
- Q-PCR is less precise than STELA but useful for broader analyses where time is a limiting factor.
Factors Influencing Telomere Length
Telomere length (TL) is influenced by a variety of factors, which play key roles in determining the rate of cellular aging and susceptibility to various diseases. These factors can be broadly categorized into genetic, environmental, intrinsic, and demographic influences.
- Genetic Factors
- Heritability
Telomere length is significantly influenced by genetics, with estimates suggesting that 44% to 80% of TL variation among individuals is heritable. - Genetic Variants
Studies have identified specific genes, like TERC and TERT, which are vital for telomere maintenance. Variants in these genes can impact both the initial telomere length at birth and its rate of attrition over time. - Parental Influence
Maternal age at birth can affect the telomere length of offspring. In some studies, maternal factors are found to be more influential than paternal ones in determining TL.
- Heritability
- Environmental Factors
- Oxidative Stress
Environmental factors, particularly oxidative stress, can speed up telomere shortening. Oxidative damage, often seen in chronic diseases or prolonged exposure to stress, contributes to faster aging at the cellular level. - Lifestyle Choices
Smoking and obesity are strongly linked to shorter telomeres. For instance, smoking has been shown to accelerate telomere attrition across various studies. These lifestyle choices not only affect the rate of telomere shortening but also impact overall health.
- Oxidative Stress
- Intrinsic Factors
- Age
As individuals age, telomeres naturally shorten due to the end-replication problem that occurs during DNA replication. This shortening is a natural consequence of cell division in somatic cells. - Sex Differences
Some studies have suggested that women may have longer telomeres than men, especially in adulthood. However, this pattern is not universally observed and may vary depending on factors such as genetics or lifestyle.
- Age
- Racial and Ethnic Differences
Variations in telomere length are also observed among different racial and ethnic groups. These differences suggest that genetic background can play a role in determining baseline telomere length, adding another layer of complexity to how TL varies across populations.
Telomere Replication
Replicating telomeres presents a unique set of challenges due to their repetitive structure and complex DNA configurations. The replication process is fundamentally shaped by the behavior of the DNA polymerase and the specific telomeric features that hinder standard replication mechanics.
- The Basics of DNA Replication:
- DNA polymerase synthesizes new DNA in the 5′ to 3′ direction.
- RNA primers are first laid down by primase to provide a starting point for DNA synthesis.
- On the leading strand, only one RNA primer is required since it synthesizes continuously in the direction of the replication fork.
- The lagging strand is synthesized in short fragments, called Okazaki fragments, each requiring a new RNA primer. These fragments are later joined together by DNA ligase.
- The end replication problem occurs at the 5′ end of the lagging strand, where a small section of DNA is lost due to the removal of the final RNA primer, resulting in gradual telomere shortening after each round of DNA replication.
- Challenges in Telomere Replication: Telomere sequences, consisting of repetitive DNA (e.g., TTAGGG), create several hurdles during replication. These challenges arise due to the repetitive nature of the sequences and the specialized structure of the telomere.
- DNA Polymerase Slippage:
The repetitive nature of telomeres increases the risk of polymerase slippage during DNA replication. This slippage can cause insertions or deletions of nucleotide bases, as well as strand mispairing, leading to potential replication errors. - Formation of G-Quadruplexes:
The high content of guanine (G) nucleotides in telomeres facilitates the formation of G-quadruplexes. These G-rich structures are highly stable due to increased hydrogen bonding between guanine bases. While these structures provide stability to the telomere, they also present a challenge to replication by stalling replication forks. Specific helicases are required to unwind these structures. If these helicases fail to function properly, the replication process stalls. - T-Loop Structure and Replication:
At the chromosome ends, telomeres form T-loops, which are crucial for telomere protection. During replication, these T-loops must be unwound for the replisome to pass through and replicate the telomere end. The large structural complexity of the telomere can prevent complete unwinding, impeding the replication machinery and causing telomere sequence loss.
- DNA Polymerase Slippage:
- Telomeric Proteins and Their Role:
To overcome these replication challenges, cells utilize specialized telomeric proteins:- Helicases and Nucleases: These enzymes are essential for unwinding G-quadruplexes and resolving replication fork stalling.
- Homology-Dependent Recombination Factors: These proteins assist in maintaining telomere stability and function during replication, helping to ensure that the telomere is copied accurately despite its structural complexities.
What is End replication problem in Telomere?
The end replication problem is a challenge specific to the replication of linear chromosomes, particularly in eukaryotic cells. During DNA replication, DNA polymerase is unable to fully replicate the sequences at the 3′ ends of the parent strands due to the unidirectional nature of DNA synthesis. This occurs because DNA polymerase can only add nucleotides to an existing 3′ end, meaning that a primer is needed to initiate replication.
In the case of the leading strand, DNA polymerase can continuously synthesize new DNA from the replication origin to the end of the strand. However, for the lagging strand, which is oriented in the opposite direction, replication is more complex. DNA synthesis on the lagging strand occurs in small fragments known as Okazaki fragments, requiring repeated primer synthesis. The final primer, which is positioned near the 3′ end of the template strand, is particularly problematic.
The issue arises when this last primer is removed and replaced by DNA, as DNA polymerase is unable to extend the strand any further. In the past, it was believed that this would result in the loss of the terminal nucleotides, causing a loss of genetic material. It was later discovered, however, that the last primer is not located exactly at the 3′ end of the template, but instead, at a distance of approximately 70-100 nucleotides from the end. This explains why DNA in human cells shortens by about 50–100 base pairs with each cell division.
Telomeres, the repetitive, non-coding sequences located at the ends of linear chromosomes, serve to protect the genetic code from degradation during replication. These regions act as protective caps, preventing the loss of coding sequences. Over time, however, telomeres themselves are progressively shortened as a result of the end replication problem.
This issue is not present in circular chromosomes, as these lack the exposed ends that linear chromosomes have. Consequently, prokaryotes that use circular chromosomes do not need telomeres. However, certain bacteria with linear chromosomes, such as Streptomyces and Agrobacterium, do possess telomeres, though their structure and function differ from those found in eukaryotic chromosomes. These bacterial telomeres may consist of protein-bound ends or hairpin loops of single-stranded DNA, highlighting the diversity of telomeric structures across organisms.
What Happens When Telomeres Become Too Short, Too Long, or Damaged?
Telomeres, the protective caps at the ends of chromosomes, play a critical role in cellular stability and function. When these telomeres become too short, too long, or damaged, they trigger a range of cellular responses that can lead to serious consequences, including cell senescence, cancer, and aging.
What Happens When Telomeres Become Too Short?
- Telomere Structure: The ends of mammalian telomeres feature a 30 overhang of single-stranded DNA, which can range from 50 to 500 nucleotides in length.
- T-loop Formation: The telomere forms a T-loop structure through the strand invasion of the telomeric repeat by the 30 overhang.
- Critical Length: Telomeres must reach a minimum length to maintain this structure. Studies have shown that 13 TTAGGG repeats (78 bp) are needed to prevent telomere fusions, which would occur if the telomere length falls below this threshold.
- Consequences of Short Telomeres:
- When telomeres shorten beyond the critical length, T-loops cannot form, and end fusions become more likely.
- These chromosomal fusions lead to genomic instability, which can trigger senescence or contribute to the development of cancer.
What Happens When Telomeres Are Too Long?
- Telomere Length and Lifespan:
- While telomeres are essential for maintaining cellular function, excessively long telomeres may not necessarily be beneficial.
- Mice possess telomeres up to ten times longer than those of humans but have a lifespan that is 30 times shorter. This suggests that telomere quality may be more important than their length.
- Excessive Telomere Length:
- Studies show that exceptionally old individuals have a higher proportion of ultra-short telomeres, indicating a relationship between telomere shortening and aging.
- Excessively long telomeres have been linked to cognitive decline, which raises concerns about the potential detrimental effects of very long telomeres.
- Optimal Telomere Length: There may be an optimal range of telomere length that balances cellular function and longevity, with too long or too short being unfavorable.
- DNA Damage Exposure: Shorter telomeres may offer an advantage by reducing the exposure to DNA damage, as long telomeres could become more susceptible to harm.
What Happens When Telomeres Are Damaged?
- Telomere Damage and DNA Repair:
- Damage to telomeric DNA, such as strand breaks, is often poorly repaired and results in the accumulation of phosphorylated γH2AX.
- This damage leads to cellular responses aimed at repairing the damage but often results in senescence or apoptosis when the damage is irreparable.
- Senescence and SASP:
- The persistent DNA damage signal activates a senescence-associated secretory phenotype (SASP), a complex mixture of cytokines that triggers cell senescence mechanisms.
- SASP aims to clear damaged cells and promote tissue regeneration, but as the body ages, its ability to clear these cells diminishes, leading to an accumulation of senescent cells.
- Pro-inflammatory State:
- The accumulation of senescent cells contributes to a pro-inflammatory state, which is thought to accelerate aging and age-related diseases.
- With aging, the immune system’s ability to clear these senescent cells weakens, exacerbating inflammation and impairing tissue regeneration.
The length and integrity of telomeres are crucial for maintaining genomic stability and cellular health. Too short or too long telomeres, as well as telomere damage, are linked to various negative outcomes, including cellular senescence, cancer, and aging-related diseases.
How Do Telomeres Change with Age?
Telomeres, the protective caps at the ends of chromosomes, undergo significant changes as organisms age. These alterations contribute to various cellular and molecular aging processes. The mechanisms behind telomere shortening are complex and multifaceted, involving both genetic and environmental factors.
- Telomere Attrition:
- As cells divide, telomeres shorten due to the telomere end-replication problem. This is a natural consequence of DNA replication, where the very end of the chromosome cannot be fully copied.
- With each division, telomeres get progressively shorter, which limits the number of times a cell can divide before it enters a state of senescence or undergoes cell death.
- DNA Damage Accumulation:
- As individuals age, DNA within cells is exposed to various types of damage.
- Double-stranded DNA breaks, if not repaired properly, may lead to the formation of terminal fragments that contain telomeric sequences. These fragments are often lost during cell division because they lack centromeres, which are necessary for attachment to the mitotic spindle.
- The failure to repair these breaks can accelerate telomere loss.
- Genetic Mutations:
- Aging may lead to an accumulation of point mutations within the telomere sequence.
- Folate deficiency, for example, can result in the incorporation of uracil instead of thymidine, leading to errors in the DNA sequence.
- Additionally, exposure to environmental toxins can cause the formation of DNA adducts, such as 8-oxo-guanine or large adducts like benzo[a]pyrene diol epoxide. These modifications interfere with the telomere sequence.
- Impact of DNA Adducts on Telomere Proteins:
- Telomere maintenance requires proteins like shelterin, including TRF1 and TRF2. These proteins play crucial roles in telomere replication, the recruitment of helicases, and the formation of T-loops, which help stabilize the telomeres.
- The accumulation of DNA adducts disrupts the function of these proteins, impeding telomere maintenance and contributing to telomere degradation over time.
- Cumulative Effects Over Time:
- With aging, the compounded damage to telomeres, including shortened length, mutations, and disrupted protein binding, weakens their protective function.
- These changes ultimately influence cellular function and contribute to age-related decline in tissue function and regeneration.
Mechanisms of Telomere Maintenance
Telomere maintenance is a crucial aspect of genomic stability. The processes involved ensure that chromosomes retain their integrity, prevent fusion, and avoid the accumulation of genetic mutations, all of which could lead to diseases like cancer. Three primary mechanisms maintain telomere function: telomere length restoration, accurate replication, and prevention of damage from various genotoxic agents.
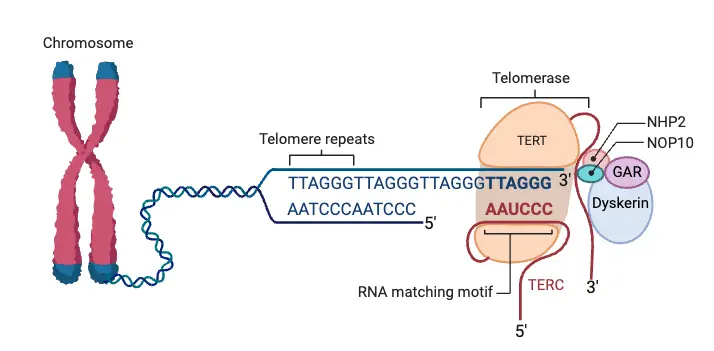
- Restoration of Telomere Length:
- For telomeres to function properly, they must be long enough to form the T-loop structure, which protects the chromosomal ends from being recognized as double-stranded breaks (DSBs).
- If telomeres are too short, this structure cannot form, and DNA repair mechanisms may mistakenly attempt to fix telomeric ends as DSBs.
- This repair can lead to fusion of broken ends, resulting in abnormal chromosomes like dicentric chromosomes. Such chromosomes can form anaphase bridges, which eventually break, leading to daughter chromosomes missing telomere sequences. This chain reaction contributes to genomic instability, which can promote cancer and other genetic disorders.
- Telomere Lengthening and Key Players:
- Telomerase is the primary enzyme complex responsible for restoring telomere length. It adds TTAGGG hexamer repeats to the telomere sequence.
- The telomerase complex consists of three main components: TERT (telomerase reverse transcriptase), TERC (telomerase RNA component), and Dyskerin.
- Tankyrase, a member of the shelterin complex, enhances telomerase access by inhibiting TRF1, another shelterin protein. This facilitates telomerase activity at the telomeres, supporting their maintenance.
- Accurate Replication of Telomeres:
- For the telomere sequence to be accurately replicated, certain nutrient cofactors are essential.
- These include folate, zinc, and magnesium, which are involved in the DNA replication process. Without these nutrients, telomere replication may become compromised, leading to defects in the newly formed telomere sequences.
- Prevention of Damage to Telomeres:
- Telomere integrity is susceptible to endogenous and exogenous genotoxic agents, including DNA strand breaks and UV-induced thymidine dimers. Surprisingly, DNA damage to the telomere sequence is not repaired by standard repair mechanisms.
- Such damage can lead to replication stress, which accelerates telomere attrition, further destabilizing the genome.
- Reducing exposure to genotoxic agents is key to maintaining telomere integrity. A strategy could involve optimizing dietary intake of nutrients that support the function of antioxidant enzymes—such as selenium and zinc—to mitigate oxidative damage to telomeres.
- Folate, known for preventing UV damage and thymidine dimer formation, also plays a role in protecting telomeres from damage caused by environmental factors like UV radiation.
These mechanisms collectively ensure that telomeres remain functional and contribute to genomic stability, preventing chromosomal abnormalities that can lead to diseases like cancer.
The Telomere Interactome – Protein-Protein Interacting Networks at Telomeres
Telomere maintenance is a complex process, relying on the orchestration of multiple protein interactions at the telomeres. At the core of this process is a six-protein complex, known as the telosome or shelterin, which forms a platform to recruit factors essential for telomere stability and function. This includes proteins such as TRF1, TRF2, RAP1, POT1, TIN2, and TPP1, all of which play distinct roles in telomere regulation and protection.
- TRF1 and TRF2:
TRF1 and TRF2 are key players in telomere maintenance, each binding to double-stranded telomeric DNA.- Both proteins contain a TRFH domain and SANT/Myb domain that recognize specific sequences within the telomeric DNA, ensuring precise interactions.
- TRF1 functions primarily as a negative regulator of telomere length. Its absence leads to embryonic lethality in mice, indicating its vital role in cellular function beyond just regulating telomere length.
- TRF2, identified later, acts as a protector of telomere ends. It works with various DNA repair factors like Apollo, ERCC1/XRF, and the MRN complex to safeguard the telomeres from damage and fusion events.
- The TRFH domain of TRF2 also plays a role in interacting with specific peptides that recruit additional regulatory proteins, including PNUTS and MCPH1, to manage telomere length and DNA repair processes at the telomeres.
- RAP1:
RAP1 is a protein that associates with TRF2 but lacks a direct telomere-binding domain. It relies on TRF2 for telomere localization.- RAP1’s role is implicated in DNA repair; its interactions with proteins such as Rad50, Mre11, and PARP1 suggest a connection to telomere recombination and stability.
- Although RAP1-deficient mice are viable, they exhibit increased telomere fragility, confirming RAP1’s involvement in telomere protection, though it is not absolutely essential for survival.
- POT1:
POT1 is a single-stranded telomeric DNA-binding protein. It directly interacts with telomeric DNA to prevent DNA damage signaling at the telomere ends.- It protects against ATR-mediated DNA damage response and regulates telomerase elongation by binding to telomeric ssDNA and inhibiting excessive DNA end resection.
- In mammals, POT1 combines the roles of two mouse orthologs, Pot1a and Pot1b, which separately control ATR-mediated responses and 5′-end resection, respectively.
- TPP1:
TPP1 is essential for the proper functioning of POT1 and is crucial for the recruitment of telomerase to the telomere.- It interacts directly with POT1 and enhances its affinity for telomeric ssDNA, ensuring telomere protection.
- TPP1 is involved in regulating telomere length and preventing ATR-mediated DNA damage signals, which are vital for telomere stability.
- Deletion of TPP1 results in reduced telomerase activity and accelerated telomere shortening, underscoring its importance in maintaining telomere length over time.
- TIN2:
TIN2 is the central hub of the telosome, bridging interactions between TRF1, TRF2, TPP1, and POT1.- It plays a critical role in telomere length regulation and protecting telomere ends from damage. Its absence leads to severe telomere instability and embryonic lethality in mice.
- TIN2 interacts with TRF2 to inhibit ATM-mediated DNA damage responses at telomeres. Additionally, it helps recruit telomerase for effective telomere elongation.
These six core proteins act as a platform, coordinating the interaction of additional factors that regulate telomere maintenance. High-throughput studies, such as BiFC-based screens, have identified hundreds of interacting partners, providing a glimpse into the vast network of proteins that converge at telomeres. This network involves protein kinases, ubiquitin ligases, and many other regulators of telomere stability, offering new insights into the complexity of telomere maintenance.
Extra-telomeric Functions of Telomere Proteins
Telomere proteins have traditionally been recognized for their roles in maintaining chromosome stability, primarily through their functions at the telomere ends. However, a growing body of research reveals that these proteins, including TIN2, TPP1, POT1, TRF1, TRF2, and RAP1, extend their influence beyond telomeric regions, contributing to various cellular processes. These non-canonical roles of telomere proteins offer insights into their broader biological significance.
- Cytoplasmic Localization and Interactions:
- Proteins such as TIN2, TPP1, and POT1 are not confined to the telomeres but also localize in the cytoplasm.
- These proteins interact in the cytoplasm, indicating a functional role outside their canonical telomeric location.
- Regulation of Stem Cells and Tumor Cells:
- TRF2 has been implicated in neural tumor progression and stem cell differentiation.
- Research suggests that TRF2 regulates proliferation and differentiation in these cells, linking telomere proteins to cellular development and cancer biology.
- Homologous Recombination Repair:
- TRF2 plays a critical role in homologous recombination (HR) repair, particularly in repairing double-strand breaks at non-telomeric regions.
- This function places TRF2 as a crucial player in maintaining genome integrity outside of its typical telomeric duties.
- RAP1 Binding and Transcriptional Regulation:
- RAP1 has been shown to bind extra-telomeric sites in subtelomeric regions, which are associated with gene expression changes.
- When RAP1 is deleted in mice, genes regulated by RAP1 are deregulated, highlighting its role in transcriptional control.
- Additionally, RAP1 associates with iκB kinases in the cytoplasm, influencing NF-κB-mediated gene expression, further emphasizing its extra-telomeric functions.
- Genomic Binding of Telomere Proteins:
- RAP1 and TRF2 have been shown to target interstitial sites in addition to their canonical telomeric sequences.
- This suggests that these proteins may regulate transcription at sites other than the telomeres.
- Telomerase and Mitochondrial Targeting:
- Telomerase has been found to contain mitochondria-targeting signals.
- Exogenous expression of telomerase can localize to the mitochondria, where it regulates apoptotic responses to oxidative stress, linking telomerase to cellular stress responses.
- TIN2 Mitochondrial Localization:
- TIN2 also harbors mitochondrial targeting signals within its N-terminal domain.
- Endogenous TIN2 can be directed to the mitochondria, where its depletion leads to altered metabolic activity, including increased oxygen consumption.
Telomeres and Pluripotency
Telomeres play a critical role in the pluripotency of stem cells, a factor that has become increasingly important in the fields of regenerative medicine and cell reprogramming. Studies on pluripotent cells such as embryonic stem cells (ESCs) and induced pluripotent stem cells (iPSCs) have highlighted the connection between telomere function and the maintenance of pluripotency.
- Telomere Length and Developmental Pluripotency:
- ESCs with long telomeres exhibit functional pluripotency, enabling the generation of complete ESC pups and germline-competent chimeras.
- These findings underscore the importance of functional telomeres in maintaining pluripotency during development.
- Telomeres as Pluripotency Markers:
- Telomere length and integrity can serve as markers for assessing the pluripotency of ESCs and iPSCs.
- The connection between telomere function and pluripotency highlights the need for proper telomere maintenance to sustain stem cell identity.
- Telomerase and Reprogramming of iPSCs:
- In iPSCs derived from telomerase-deficient cells, telomerase activity was shown to be critical for reprogramming and self-renewal.
- The re-activation of telomerase occurs earlier than the activation of pluripotency genes such as Oct4 and Nanog during reprogramming, pointing to the vital role of telomere regulation in establishing pluripotency.
- Telomere Function in Stem Cell Homeostasis:
- Telomere maintenance plays a role not only in the initial establishment of pluripotency but also in the maintenance of stem cell-based tissue homeostasis.
- The regulation of telomeres during reprogramming suggests that telomere length and function are essential for long-term stability and functionality of pluripotent cells.
These observations affirm that telomere length and telomerase activity are integral to both the generation and maintenance of pluripotency, offering key insights into the molecular mechanisms that govern stem cell behavior.
Mechanisms of Telomere Shortening
Telomere shortening is a crucial biological event that happens during cellular division. Several mechanisms drive this process, contributing to the gradual decline in telomere length. These mechanisms include both intrinsic processes, like DNA replication, and external factors, such as oxidative stress or environmental influences.
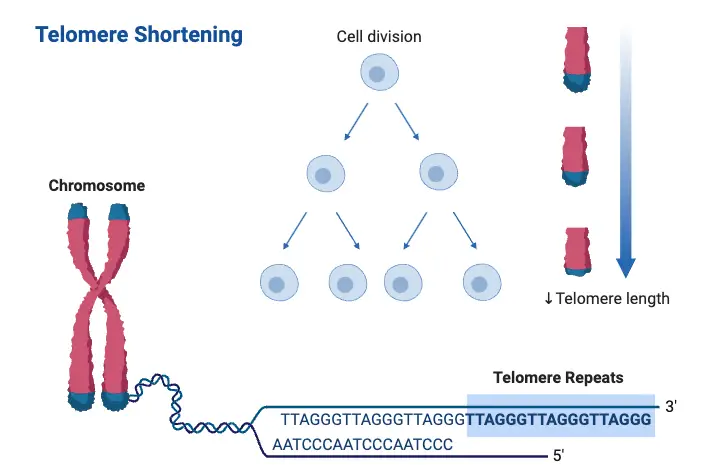
- End Replication Problem
During DNA replication, DNA polymerases cannot fully replicate the ends of chromosomes. This is known as the end replication problem. As a result, the telomeric DNA at the chromosome ends is progressively lost with each cell division. Over time, this continuous shortening limits the number of times a cell can divide before entering senescence or apoptosis. - Oxidative Stress
Telomeres are highly susceptible to damage caused by reactive oxygen species (ROS), which are molecules that can damage DNA. Oxidative stress leads to structural changes in telomeric DNA, accelerating its attrition. This process is further aggravated by external environmental factors such as pollution, smoking, or chronic inflammation, which can cause elevated levels of ROS, speeding up telomere shortening. - Replication Stress
Telomeres are vulnerable to replication stress, which can occur due to various factors like limited nucleotide availability or the formation of secondary DNA structures, such as G-quadruplexes. These structures can stall the replication machinery, leading to incomplete DNA replication and subsequent telomere loss. This type of stress is especially prominent in rapidly dividing cells, such as cancerous cells, where the accelerated cell division intensifies telomere attrition. - Telomere Structure and Shelterin Complex
The shelterin complex is a group of proteins that bind to telomeric DNA and play a critical role in preserving telomere integrity. Shelterin protects telomeres from being recognized as DNA damage sites and also regulates telomerase activity, the enzyme responsible for elongating telomeres. When shelterin components are dysfunctional or displaced, the telomeres become unprotected, leading to a DNA damage response that further contributes to telomere shortening. - Cellular Senescence
As telomeres shorten and reach a critically low length, they trigger a process known as cellular senescence. In this state, cells can no longer divide. While cellular senescence serves as a protective measure against tumor development, it also contributes to tissue aging and dysfunction. Senescent cells can secrete pro-inflammatory molecules that exacerbate tissue damage and accelerate the aging process. - Environmental and Lifestyle Factors
External factors, including chronic stress, poor diet, lack of physical activity, and exposure to harmful substances, can impact the rate at which telomeres shorten. Research shows that regular physical activity correlates with longer telomeres, while chronic psychological stress leads to accelerated telomere attrition. These lifestyle factors further highlight how both internal biological processes and external influences work together in driving telomere shortening.
Influencing Factors of Telomere Shortening
Telomere shortening is a multifactorial process driven by a combination of genetic, environmental, and lifestyle factors. These factors play distinct roles in determining the rate at which telomeres shorten, contributing to aging and susceptibility to various diseases.
- Genetic Factors
Telomere length (TL) is highly heritable, with estimates ranging from 34% to 82%.- Specific genetic variations, particularly single nucleotide polymorphisms (SNPs), influence telomere maintenance.
- Genes like TERT and TERC, which are involved in telomere maintenance, play a key role in determining both the initial length of telomeres and their rate of shortening over time.
- Parental age also impacts offspring TL, with older mothers generally associated with shorter telomeres in their children, while paternal age tends to correlate with longer telomeres due to the accumulation of longer telomeres in sperm as fathers age.
- Environmental Factors
- Oxidative Stress: Exposure to environmental toxins, pollution, and other stressors can accelerate telomere shortening. Reactive oxygen species (ROS) damage telomeric DNA, leading to its attrition.
- Chronic Inflammation: Long-term inflammation can further contribute to telomere shortening. Inflammatory cytokines activate cellular stress responses that negatively affect TL.
- Lifestyle Choices
- Smoking: Smoking is strongly linked to shorter telomeres. The harmful chemicals in tobacco induce oxidative stress and inflammation, accelerating telomere attrition.
- Diet and Physical Activity: A diet rich in antioxidants and regular exercise are associated with longer telomeres. In contrast, obesity and sedentary lifestyles increase oxidative stress and inflammation, contributing to shorter telomeres.
- Intrinsic Factors
- Cellular Replication Stress: Cells that replicate rapidly, such as immune cells during immune responses, experience more telomere shortening due to the end replication problem and replication stress.
- Age: As individuals age, their telomeres naturally shorten due to cumulative cell divisions and limitations in DNA replication. Older individuals typically have shorter telomeres across various tissues.
Implications of Telomere Shortening
Telomere shortening plays a pivotal role in aging, disease progression, and overall health outcomes. It impacts a range of biological processes and contributes to several health conditions.
- Aging and Cellular Senescence
- Telomere shortening is a key marker of biological aging.
- As telomeres shorten to critical lengths, cells enter a state of senescence, where they stop dividing, driving aging and tissue dysfunction.
- Telomeres act as a mitotic clock, restricting the number of times a cell can divide. This helps maintain genomic stability and prevents the risk of cancer from uncontrolled cell proliferation.
- Age-Related Diseases
- Cardiovascular Disease (CVD): Individuals with shorter telomeres face a higher risk of cardiovascular diseases, including myocardial infarction, heart failure, and stroke. Short telomeres in leukocytes are linked to increased cardiovascular events, highlighting their potential role in cardiovascular aging.
- Cancer: The relationship between telomere length and cancer is complex. Shorter telomeres may increase genomic instability, which can promote cancer. On the other hand, longer telomeres could also be linked to cancers due to enhanced cellular proliferation, complicating cancer treatments targeting telomerase.
- Neurodegenerative Diseases: Short telomeres have been associated with neurodegenerative diseases like Alzheimer’s. Telomere attrition may affect neuronal health, contributing to cognitive decline by impairing cellular resilience against stress.
- Mortality Risk
- Individuals with shorter telomeres show a higher risk of all-cause mortality. This correlation suggests that telomere length could be a valuable biomarker for predicting lifespan and general health status.
- Genetic and Environmental Interactions
- Genetic Factors: Genetic predispositions play a crucial role in determining telomere length and its rate of attrition. Variants in genes related to telomerase activity can influence susceptibility to age-related diseases.
- Environmental Stressors: External factors like oxidative stress, chronic inflammation, and poor lifestyle choices (such as smoking and poor diet) can accelerate telomere shortening. These factors highlight the importance of managing environmental stress to maintain telomere health and slow the aging process.
- Potential Therapeutic Targets
- Telomerase Activation: Research is ongoing into the therapeutic potential of activating telomerase to elongate telomeres and treat age-related diseases. However, there are risks, such as promoting the growth of cancer cells. Any potential therapy would need to carefully balance the benefits of telomere elongation with the risks of uncontrolled cell growth.
Telomere Dysfunction and the p53/p16 Pathways
Telomere dysfunction is linked to several critical cellular processes, including senescence and cell death. The disruption of telomeres triggers a cascade of events, activating specific signaling pathways like p53 and p16, which are central to how cells respond to telomere damage. The p53/p16 pathways are crucial in the progression of aging, cancer, and the maintenance of cellular integrity.
- Telomere Dysfunction and Cellular Senescence:
- Telomere damage directly contributes to cellular senescence, a state where cells lose their ability to divide.
- This damage often leads to the accumulation of DNA damage at the telomeres, which is a hallmark of aging and various diseases.
- Key Pathways Involved:
- p53 and p16 are central to the cellular response to telomere dysfunction.
- p53 acts as a tumor suppressor that can trigger cell cycle arrest or apoptosis in response to telomere shortening or damage.
- p16 (a cyclin-dependent kinase inhibitor) plays a role in inducing senescence when telomeres become critically short, further contributing to cell aging and limiting the proliferative capacity of damaged cells.
- Research Insights on p16/p53 Regulation:
- Pioneering studies from researchers like Tong and Zhang have highlighted the interplay between the p16/p21/p53 pathway and telomere function, illustrating how these pathways influence aging and cancer progression.
- Their work shows that telomere shortening can trigger these pathways, leading to senescence or cell death as a protective mechanism against the accumulation of mutations or cancerous growth.
- Telomere-Targeted Interventions:
- Recent findings suggest potential therapeutic avenues involving traditional Chinese medicine to combat telomere shortening and aging.
- Compounds from Astragalus membranaceous, such as HDTIC, have been shown to prevent telomere shortening and enhance DNA repair by reducing oxidative stress, offering a promising approach for anti-aging therapies.
- In related studies, the Blasco group found that the compound TA-65, also derived from Astragalus membranaceous, could activate telomerase, elongating telomeres and preventing DNA damage. This, in turn, extended the tumor-free lifespan of mice, suggesting potential benefits for therapeutic applications.
These studies underscore the importance of telomere regulation and how the p53 and p16 pathways mediate cellular responses to telomere dysfunction, influencing both aging and cancer processes.
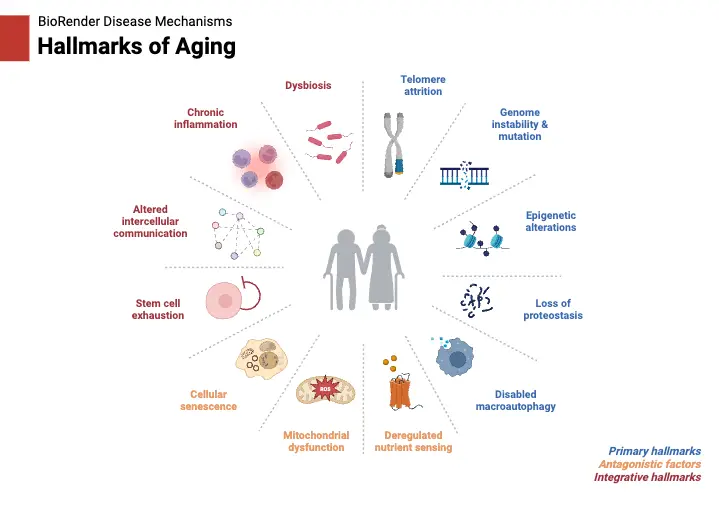
Dysfunctional Telomeres and the Hallmarks of Cellular Aging – Telomeres and aging
Telomere dysfunction plays a critical role in aging, interacting with various biological processes to accelerate aging phenotypes. These dysfunctional telomeres drive multiple hallmarks of aging, such as cellular senescence, stem cell exhaustion, genomic instability, mitochondrial dysfunction, epigenetic dysregulation, loss of proteostasis, altered nutrient sensing, and inflammation. Below is a detailed examination of how telomere dysfunction links to these aging processes:
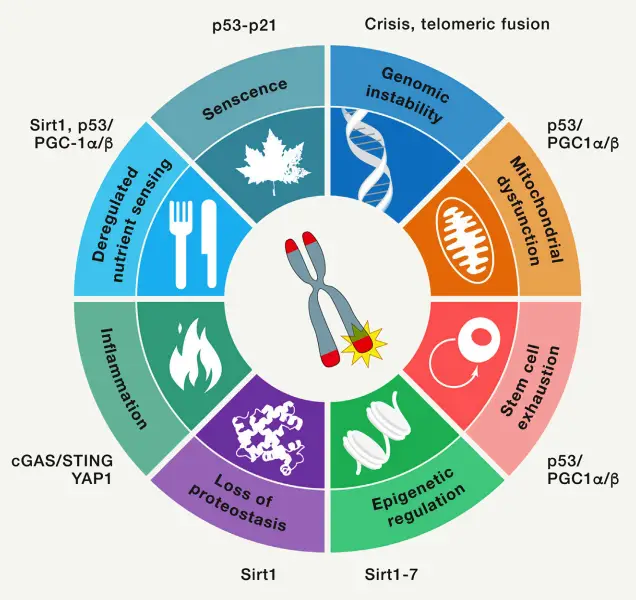
- Cellular Senescence
- Senescent cells accumulate in aging tissues and contribute to aging and age-related diseases.
- Telomere dysfunction can trigger cellular senescence, particularly in low-proliferative stroma tissue. This is often linked to reactive oxygen species (ROS)-induced damage to telomeres, which leads to senescence through telomere dysfunction-induced foci (TIF) formation.
- Senescent cells release pro-inflammatory factors, such as IL-6 and TNF-α, which disrupt organ function and tissue homeostasis.
- Stem Cell Exhaustion
- Tissue stem cell exhaustion is a key characteristic of aging. In the case of telomere dysfunction, progressive telomere erosion in stem cells can activate the p53-dependent apoptotic pathway, leading to cell loss.
- In TERT- or TERC-null mice, this loss of stem cells contributes to organ atrophy, especially in tissues with high regenerative needs such as skin, intestine, and blood. In some models, telomerase can also affect stem cell biology through the WNT pathway, further influencing homeostasis.
- Genomic Instability
- Telomere dysfunction drives genomic instability by causing chromosomal abnormalities, such as amplifications, deletions, and translocations. This instability is especially noticeable in tumors, including colorectal cancer.
- Eroded telomeres undergo end-to-end fusions, leading to breakage-fusion-bridge (BFB) cycles, aneuploidy, and chromosomal rearrangements, contributing to cancer progression and increased mutational burden with aging.
- The loss of p53 function in these cells allows them to survive these DNA double-strand breaks, producing aberrant chromosomal imbalances that drive cancer initiation.
- Mitochondrial Dysfunction
- Mitochondria and telomeres are closely connected. In aging, telomere dysfunction leads to mitochondrial decline, characterized by decreased ATP production and increased ROS levels.
- Dysfunctional mitochondria contribute to aging-related phenotypes such as frailty and impaired oxidative defense. Mice with mitochondrial DNA polymerase mutations exhibit premature aging that mirrors the effects of telomere dysfunction.
- The interaction between telomeres and mitochondria is especially pronounced through the p53-mediated repression of PGC1a/b, which reduces mitochondrial biogenesis and oxidative defense mechanisms.
- Epigenetic Dysregulation
- Aging-related changes in the epigenetic landscape include modifications like increased local methylation and altered acetylation patterns. Telomere dysfunction contributes to epigenetic changes by affecting sirtuins (SIRTs), which regulate longevity and stress resistance.
- Telomere dysfunction can lead to the repression of SIRT1 and other sirtuins, further exacerbating aging phenotypes related to epigenetic regulation. This includes a disruption in the balance of histone modifications that are crucial for maintaining genome stability and cellular function.
- Loss of Proteostasis
- Telomere dysfunction is linked to the hallmark of altered proteostasis. It disrupts protein homeostasis by impeding the heat shock response, critical for maintaining proper protein folding.
- Dysfunctional telomeres repress SIRT1, which normally activates heat shock factor-1 (HSF-1) to induce heat shock proteins like HSP70. Without this activation, protein misfolding increases, contributing to the accumulation of misfolded proteins in neurons and other tissues.
- The buildup of misfolded proteins is particularly harmful in neurodegenerative diseases, such as Alzheimer’s and Parkinson’s, where it leads to neuronal death and cognitive decline.
- Altered Nutrient Sensing
- Telomere dysfunction also affects nutrient sensing pathways, particularly those involving IGF-1, mTOR, and AMPK, which regulate metabolic homeostasis.
- Telomere dysfunction activates p53, which represses PGC1a and SIRT1, disrupting glucose metabolism and reducing gluconeogenesis. This has consequences on nutrient sensing, leading to metabolic dysregulation in aging tissues.
- The impairment in mitochondrial function resulting from telomere dysfunction shifts metabolism toward glycolysis, further disturbing energy homeostasis and the NAD/NADH balance.
- Inflammation
- Telomere dysfunction has a profound effect on the inflammatory response. As cells with dysfunctional telomeres undergo senescence, they secrete inflammatory cytokines like IL-6 and TNF-α, contributing to tissue inflammation.
- Telomere-induced inflammation can also activate autophagic cell death pathways via the cGAS/STING pathway, generating extrachromosomal DNA fragments that exacerbate the inflammatory response.
- The connection between telomere dysfunction and inflammation is also linked to the activation of the ATM/cABL/YAP1 axis, which drives the production of IL-18, further amplifying inflammation and immune cell recruitment.
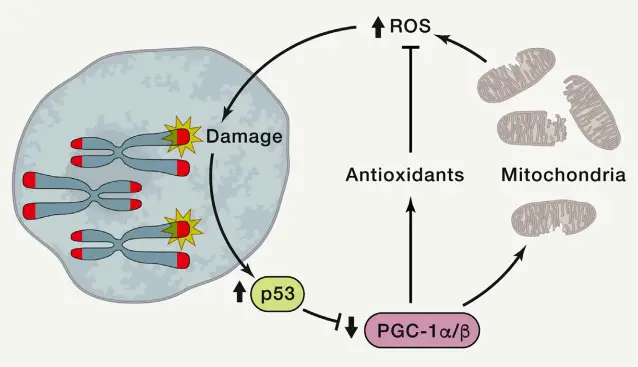
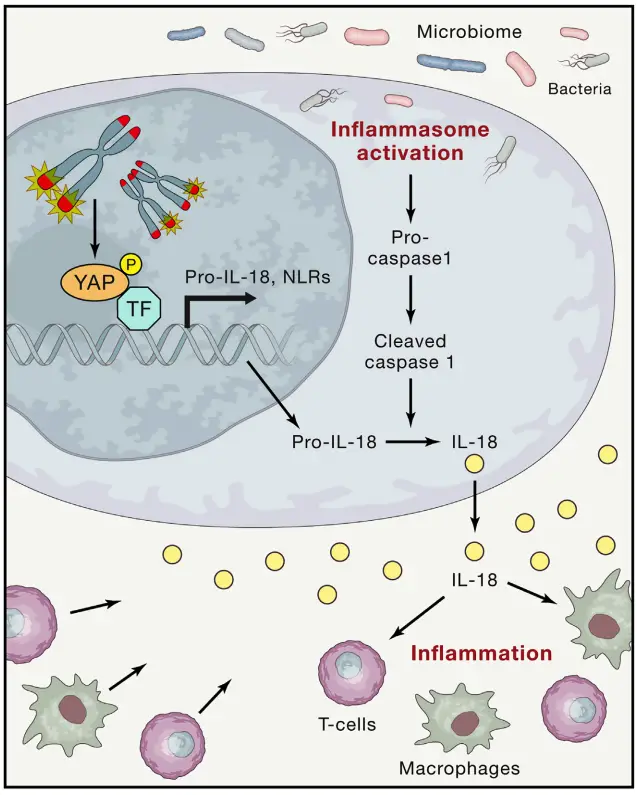
inflammation (Image Source: https://doi.org/10.1016/j.cell.2020.12.028)
Clinical Significance of Telomeres
Telomeres play a crucial role in cellular function, aging, and the development of certain diseases. Their length and stability are key to understanding a variety of clinical conditions, from aging to cancer. The impact of telomere dynamics on health is significant, as they are involved in processes such as oxidative stress, cellular senescence, and cancer progression.
- Telomeres and Oxidative Stress:
- Telomeres are vulnerable to oxidative stress, which is caused by both endogenous and external factors.
- These stressors include mitochondrial dysfunction, smoking, alcohol consumption, inflammation, and a poor diet.
- Oxidative stress leads to the production of reactive oxygen species (ROS), which can damage telomeres.
- Telomeres, particularly their G-rich sequences, are sensitive to this oxidative damage.
- When exposed to ROS, telomeres also face a suppressed DNA damage response, leading to inefficient repair mechanisms.
- Chronic inflammation, as seen in obesity, increases ROS production, contributing to telomere shortening.
- There is also an observed correlation between telomere length and psychosocial stress, with conditions like major depressive disorder leading to increased oxidative stress and inflammation.
- On the other hand, physical activity has been shown to maintain telomere length, highlighting its potential for promoting cellular health.
- Telomeres and Cancer:
- Most human somatic cells lack telomerase, the enzyme responsible for synthesizing telomeres.
- However, more than 90% of tumor cells exhibit telomerase activity, allowing them to bypass normal cell division limits and become immortalized.
- Cancer cells gain a functional advantage through the upregulation of telomerase, which is often due to the amplification of the hTERT gene.
- DNA breakage or chromosomal fusions can trigger this upregulation, leading to tumorigenesis.
- Telomerase activity in cancer cells may also be enhanced through promoter methylation, which prevents the binding of transcriptional repressors and promotes further transcription of the telomerase gene.
- TERT mutations are implicated in various cancers, including uroepithelial cancers, thyroid, melanoma, basal cell carcinomas, and glioblastomas.
- Telomerase-Targeted Cancer Immunotherapy:
- Given that telomerase is overactive in most cancer cells, it presents a valuable target for immunotherapy.
- Telomerase-targeted therapies aim to exploit this enzyme’s presence in tumors to selectively destroy cancer cells.
- Oligonucleotide inhibitors: These modified nucleic acids inhibit telomerase activity, leading to telomere shortening and triggering cellular senescence or apoptosis.
- Immunotherapeutic approaches: These use T lymphocytes that are highly reactive against the catalytic enzyme telomerase, aiming to eliminate tumor cells.
- Telomerase-directed gene therapy: This strategy involves targeting telomerase promoters to induce cell death in cancer cells that rely on telomerase for immortality.
Telomeres Functions
Telomeres serve critical functions that are essential for maintaining genomic integrity and regulating cellular processes. These functions primarily focus on protecting chromosome ends from degradation, fusion, and other forms of damage that could disrupt cellular operations.
- Chromosomal Stability and Protection:
- Telomeres maintain the stability of chromosomes by protecting their ends from degradation.
- They prevent the chromosome ends from mistakenly being recognized as double-stranded breaks. This is achieved through the formation of T-loops at the 3′ G-rich end overhang.
- The T-loop structure prevents inappropriate DNA end-joining or activation of the DNA damage response, ensuring that chromosomes are not repaired inappropriately.
- Prevention of Chromosomal Fusion:
- Role in Replication and Aging:
- Due to the nature of DNA replication, telomeres are produced as a result of incomplete replication at the chromosome ends.
- DNA loss occurs with each replication cycle, but telomeres ensure that critical genetic information is not lost.
- This protective function is essential for cellular longevity, influencing processes like aging and cell division.
- Regulation and Stabilization of Chromosomes:
- Telomeres are essential for attracting telomerase, the enzyme responsible for maintaining telomere length and function.
- They also contribute to stabilizing eukaryotic chromosomes, helping ensure proper chromosome dynamics during cell division.
- Interaction with Nuclear Envelope and Meiotic Recombination:
- Telomeres often localize beneath the nuclear envelope, and their specific interaction with structures like the spindle pole body is crucial for meiotic recombination in organisms such as fission yeast.
- This ensures that meiotic processes are properly executed, maintaining the integrity of genetic information passed on to the next generation.
Importance of Telomeres
Telomeres are critical structures at the ends of chromosomes that play an essential role in maintaining genomic stability. As a cell divides, the telomeres shorten with each cycle, which acts as a biological clock for cellular lifespan. The integrity of telomeres is fundamental to the functioning of cells and overall organismal health.
- Mitotic Clock:
- Telomeres function as a mitotic clock, shortening with each division cycle.
- As a cell undergoes repeated divisions, the telomeres get progressively shorter, signaling when the cell has reached its division limit.
- This process is crucial for limiting the number of times a cell can divide, preventing unchecked proliferation.
- When telomeres reach a critically short length, it triggers a DNA damage response, resulting in cellular senescence or apoptosis (programmed cell death).
- Telomere shortening thus serves as a safeguard against uncontrolled cell growth, which is linked to cancer and other age-related diseases.
- Chromosomal Integrity:
- Telomeres are composed of repeat sequences that protect the chromosomal ends from damage.
- These structures ensure that the chromosomes do not undergo degradation or fusion during cell division, which would lead to genomic instability.
- Without intact telomeres, the chromosomes can break, fuse, or form abnormal structures, leading to chromosomal instability.
- This instability is a contributing factor to genetic mutations, which can drive the development of various diseases, including cancers.
- By maintaining chromosomal integrity, telomeres are essential for cellular function and longevity.
- Link to Aging and Disease:
- Excessive telomere shortening is associated with accelerated aging and the onset of age-related diseases.
- Shortened telomeres impair the cell’s ability to divide properly, leading to reduced tissue regeneration and repair.
- This process is linked to conditions such as heart disease, neurodegenerative disorders, and other age-associated health issues.
- Telomere attrition, therefore, is not just a marker of aging but a fundamental mechanism behind age-related decline in cellular and tissue function.
Telomeres and Telomerase in Age-Related Diseases and Cancer
Telomeres are critical to cellular aging, tissue regeneration, and cancer development. Their maintenance, primarily through the enzyme telomerase, plays a role in both age-related diseases and cancer. The interaction between telomeres and telomerase, and their dysfunction, has been linked to a variety of diseases, highlighting their importance in genomic stability.
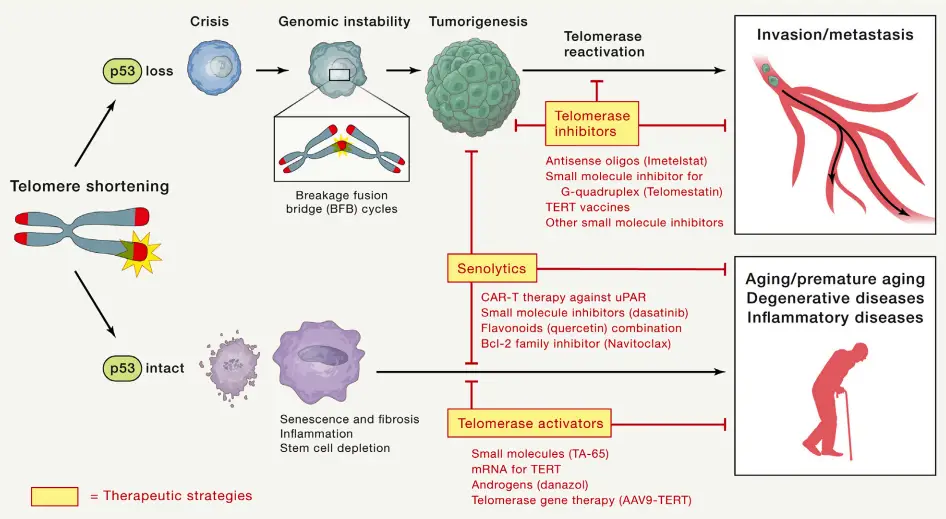
- Telomeres and Aging
- Telomeres protect the ends of chromosomes, but they shorten as cells divide. In aging, this progressive telomere attrition leads to cellular dysfunction.
- Mouse studies have shown how telomeres and telomerase impact aging. Mice with shorter, human-like telomeres develop accelerated aging features, such as tissue stem cell depletion, reduced memory, and weakened cardiac function.
- Human studies also link telomere dysfunction to aging processes. As telomeres shorten, cells experience DNA damage responses, such as cell cycle arrest or apoptosis. This is particularly evident in tissues like the skin, gastrointestinal tract, and hematopoietic system, where high turnover accelerates telomere attrition.
- In low-turnover tissues such as the heart and brain, oxidative stress plays a major role in telomere degradation. This damage leads to telomere uncapping and contributes to aging and disease development.
- Diseases linked to telomere dysfunction include cardiovascular diseases (atherosclerosis, coronary artery disease), neurological diseases (Parkinson’s), and metabolic disorders (type II diabetes). Inflammatory diseases, such as osteoarthritis and inflammatory bowel disease, have also been associated with telomere shortening.
- Telomere Dysfunction and Inflammation
- Telomere dysfunction can contribute to inflammation and age-related diseases. As telomeres shorten, they can drive a pro-inflammatory environment, which exacerbates tissue damage.
- Telomeropathy, a condition caused by mutations in telomere-maintenance genes, results in depletion of stem cells and immune cells, leading to diseases like pulmonary fibrosis and liver cirrhosis.
- The link between telomere shortening and inflammation is significant. For example, high ROS levels from mitochondrial dysfunction can accelerate telomere attrition, amplifying inflammatory responses in tissues.
- Removal of senescent cells from aging tissues, such as in Alzheimer’s disease models, has shown that senescence and inflammation contribute to disease progression. These findings have driven the development of senolytic therapies aimed at clearing senescent cells to mitigate disease symptoms.
- Telomerase and Cancer
- Telomerase is commonly reactivated in cancer cells, providing a mechanism to bypass the normal telomere shortening seen in most somatic cells. Telomere dysfunction plays a major role in cancer initiation and progression.
- In mice with short telomeres and deficient telomerase, chromosome instability increases. This instability results in genetic alterations that promote cancer. These findings were supported by studies showing that telomere dysfunction, particularly in the absence of p53, leads to chromosomal breakage and genomic instability.
- Cancer cells with dysfunctional telomeres enter a crisis state, which is followed by the activation of telomerase. This process stabilizes the genome, allowing the cancer to progress. This mechanism is observed in various types of epithelial cancers in humans.
- The telomere-based crisis mechanism is crucial for the initiation of cancer. In prostate cancer models, telomere dysfunction induced apoptosis and reduced proliferation, leading to smaller tumors. However, upon reactivation of telomerase, the tumors progressed, illustrating how telomerase reactivation is pivotal for cancer progression.
- Alternative Telomere Maintenance Mechanisms in Cancer
- While telomerase is the most common mechanism for telomere maintenance in cancer, some cancers utilize an alternative mechanism known as ALT (Alternative Lengthening of Telomeres).
- ALT, which occurs in 5-15% of human cancers, uses homologous recombination to extend telomeres. This mechanism is associated with poor prognosis, especially in cancers like osteosarcoma and glioblastoma.
- ALT-positive cancers face challenges such as immune surveillance and senescence. Despite these barriers, ALT can maintain telomere length, though it is less efficient at driving aggressive malignancy compared to telomerase reactivation.
- In mouse models, ALT has been shown to resist telomerase inhibitors, suggesting it could be a mechanism of resistance to therapies targeting telomerase. ALT-positive cells often exhibit mitochondrial dysfunction and high levels of ROS, making them susceptible to treatments targeting antioxidant systems.
- Telomere Dysfunction and Cancer Therapy
- The role of telomeres in cancer has led to the development of therapies targeting telomerase. However, alternative mechanisms like ALT complicate treatment strategies.
- In lymphoma models, telomerase inhibition caused tumors to re-enter telomere crisis, but some tumors switched to ALT to continue growing. This highlights the potential for ALT to act as a resistance mechanism against telomerase-targeting therapies.
- Targeting ALT in combination with telomerase inhibitors, or therapies aimed at mitochondrial stress, might offer more effective cancer treatment strategies by preventing both telomerase reactivation and ALT.
- Dhillon, V., Bull, C., & Fenech, M. (2016). Telomeres, Aging, and Nutrition. Molecular Basis of Nutrition and Aging, 129–140. doi:10.1016/b978-0-12-801816-3.00010-8
- Singh, D. K., Mattoo, A. R., & Pandita, T. K. (2015). Telomeres and Telomerase☆. Reference Module in Biomedical Sciences. doi:10.1016/b978-0-12-801238-3.98751-8
- Lee J, Pellegrini MV. Biochemistry, Telomere And Telomerase. [Updated 2022 Dec 11]. In: StatPearls [Internet]. Treasure Island (FL): StatPearls Publishing; 2024 Jan-. Available from: https://www.ncbi.nlm.nih.gov/books/NBK576429/
- Lu W, Zhang Y, Liu D, Songyang Z, Wan M. Telomeres-structure, function, and regulation. Exp Cell Res. 2013 Jan 15;319(2):133-41. doi: 10.1016/j.yexcr.2012.09.005. Epub 2012 Sep 21. PMID: 23006819; PMCID: PMC4051234.
- Aubert G, Lansdorp PM. Telomeres and aging. Physiol Rev. 2008 Apr;88(2):557-79. doi: 10.1152/physrev.00026.2007. PMID: 18391173.
- Chakravarti, D., LaBella, K. A., & DePinho, R. A. (2021). Telomeres: history, health, and hallmarks of aging. Cell, 184(2), 306–322. doi:10.1016/j.cell.2020.12.028
- Wellinger, Raymund & Zakian, Virginia. (2012). Everything You Ever Wanted to Know About Saccharomyces cerevisiae Telomeres: Beginning to End. Genetics. 191. 1073-105. 10.1534/genetics.111.137851.
- https://study.com/academy/lesson/telomeres-definition-function-quiz.html
- https://www.jax.org/news-and-insights/minute-to-understanding/what-are-telomeres
- https://www.news-medical.net/life-sciences/What-are-Telomeres.aspx
- https://www.yourgenome.org/theme/what-is-a-telomere/
- https://www.genome.gov/genetics-glossary/Telomere
- https://byjus.com/neet/telomeres/
- https://www.khanacademy.org/science/biology/dna-as-the-genetic-material/dna-replication/a/telomeres-telomerase
- https://learn.genetics.utah.edu/content/basics/telomeres/