In Taxonomy, evidence is the information used in context for a purpose such as identification or classification. The foundation of plant taxonomy was laid on a number of characters (attributes) of plants and plant groups studied from time to time. Such taxonomic information can be realised in many ways to prove a hypothesis, solve a problem, characterize a taxon, classify a group of plants or derive evolutionary relationship among plants. The gross morphology provides the foundation of plants taxonomy and it is supplemented by the information from various other fields of botany. In this Unit you will study about taxonomic information that can be collected from a few specific fields such as: palynology; cytology; phytochemistry and molecular biology.
What is Alpha taxonomy and omega taxonomy?
The evolution of taxonomy has introduced various methods and phases to classify and understand plant life. Two significant approaches in this field are Alpha Taxonomy and Omega Taxonomy, which represent different stages and methodologies in the process of plant classification.
Alpha Taxonomy
Alpha Taxonomy primarily deals with the initial stages of taxonomic work, including the characterization, identification, classification, and naming of organisms. This approach is rooted in what is often referred to as classical or formal taxonomy, which has been in practice for centuries. The core of Alpha Taxonomy involves the empirical analysis of external morphology. It relies heavily on observable characteristics to classify organisms, and its methodologies are largely descriptive and analytical.
In the pioneering or exploratory phase of Alpha Taxonomy, taxonomists collect plant specimens to analyze their features. This phase is essential for laying the groundwork for further classification efforts. It involves the basic classification of plants based on their morphological attributes, forming a preliminary system that is later refined. As the field progresses, the initial data collection and classification efforts lead to a more systematic approach, often called the consolidation phase. Here, the vast amount of collected data is evaluated and used to develop comprehensive classification systems.
Omega Taxonomy
In contrast, Omega Taxonomy represents a more advanced and integrative approach to taxonomy. After establishing a basic classification system through Alpha Taxonomy, Omega Taxonomy seeks to enhance and refine this system by incorporating evolutionary and phylogenetic perspectives. This approach employs a broader range of features, including cytology and genetics, to provide a deeper understanding of taxonomic relationships.
The experimental or biosystematic phase of Omega Taxonomy involves detailed studies of cytology and genetics to explore the evolutionary relationships between plant species. By utilizing a variety of scientific disciplines, Omega Taxonomy aims to offer a more nuanced interpretation of plant classifications. This phase progresses into the encyclopedic or holotaxonomic phase, where taxonomists compile extensive data from multiple sources to gain a comprehensive view of plant biology.
Omega Taxonomy, also known as Beta Taxonomy or Neotaxonomy, integrates findings from various scientific fields, such as morphology, anatomy, palynology, cytology, biochemistry, and molecular biology. This approach emphasizes the importance of synthesizing information from diverse sources to create a robust and accurate classification system.
Taxonomic evidences from palynology
Palynology, the scientific study of pollen and spores, offers crucial insights into the taxonomy of plants. By analyzing the characteristics of pollen grains, taxonomists can gain valuable information about plant classification and evolutionary relationships. Below are key aspects of palynological evidence used in taxonomy.
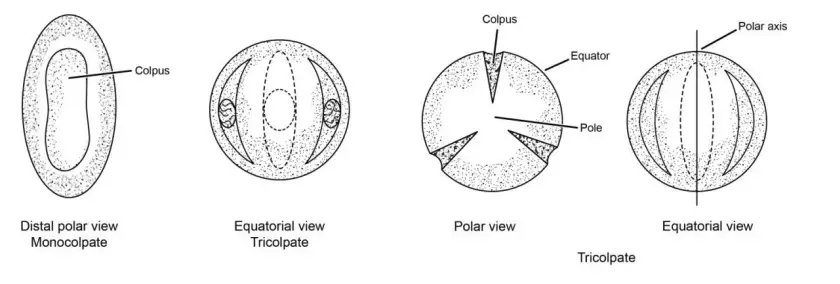
Key Characteristics of Pollen Grains
- Wall Structure:
- The structure of the pollen wall, including its architecture and stratification, is essential for classification. This can include aspects such as the exine’s structure and sculpture.
- Polarity and Symmetry:
- Polarity refers to the orientation of the pollen grain’s features, while symmetry pertains to the overall shape and balance of the grain.
- Shape and Size:
- The shape and size of pollen grains are variable and can be used to distinguish between species or genera. For instance, pollen grains can be boat-shaped or globose.
- Apertures:
- The number and type of apertures (germinal openings) on a pollen grain are significant. These can vary widely, from monocolpate (one aperture) to tricolpate (three apertures) forms.
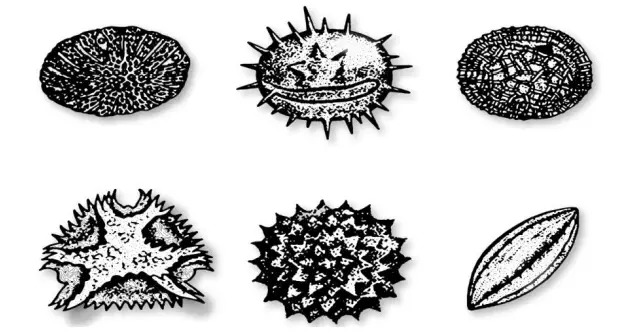
Examples of Pollen Grain Characteristics
- Monocolpate and Tricolpate Pollen Grains:
- Monocolpate: Boat-shaped with a single long germinal furrow. Typical of primitive dicotyledons like Piperaceae.
- Tricolpate: Globose with three germinal apertures. Found in advanced dicotyledons.
- Family-Specific Characteristics:
- Betulaceae:
- Betula: Knob-like exine.
- Corylus: Club-shaped exine.
- Carpinus: Unexpanded exine.
- Alnus: Arch-like exine.
- Epacridaceae:
- Brachyloma: Solitary pollen grains.
- Epacris: Pollen grains in tetrads.
- Betulaceae:
- Pollen Size Variability:
- Malva rotundifolia: Pollen size ranges from 74-84 µm.
- Malva sylvestris: Pollen size ranges from 105-126 µm.
Taxonomic Case Study: Trisyngyne
The genus Trisyngyne illustrates the importance of palynological evidence in taxonomy. Initially classified in the family Euphorbiaceae based on morphological evidence, it was later reassigned to Fagaceae after pollen grain analysis. The pollen grains from the male specimens were found to closely resemble those of Fagaceae, leading to the reclassification. Some taxonomists suggest the name Nothofagus for this genus, placing it in the family Nothofagaceae.
Taxonomic evidences from Cytology
Cytology, the study of cell structure and function, provides critical taxonomic evidence through the analysis of chromosomes. This includes chromosome number, structure, and behavior during meiosis. These cytological features offer insights into plant classification and evolutionary relationships.
Chromosome Numbers
- Chromosome Constancy:
- Each species typically has a constant number of chromosomes in its cells. Chromosome numbers are used as a taxonomic character because closely related species often share similar chromosome counts, while distantly related ones have distinct numbers.
- Range and Variability:
- In angiosperms, chromosome numbers vary widely. For example:
- Haplopappus gracilis: 2n = 4
- Poa litorosa: 2n = 530
- Chromosome numbers provide valuable taxonomic data. For instance, the genus Festuca shows a series of chromosome numbers (2n = 14, 28, 42, etc.), indicating a polyploid series where species have multiples of a basic chromosome number.
- In angiosperms, chromosome numbers vary widely. For example:
Chromosome Structure
- Centromere Position:
- The position of the centromere divides chromosomes into categories: metacentric, acrocentric, and telocentric. These structural differences are significant in taxonomy, with metacentric chromosomes often being considered more advanced than acrocentric ones.
- Karyotype Analysis:
- The karyotype, or the complete set of chromosomes in a cell, is analyzed to understand chromosome number, size, volume, and type.
- Paeonia: Large chromosomes suggest placement in the family Paeoniaceae rather than Ranunculaceae.
- Polygonum: Despite morphological differences, similar chromosome structures led to the unification of different species under Polygonum amphibium.
- Chromosome karyotype studies have also influenced taxonomic classifications, such as separating Butomus into its own family Butomaceae.
- The karyotype, or the complete set of chromosomes in a cell, is analyzed to understand chromosome number, size, volume, and type.
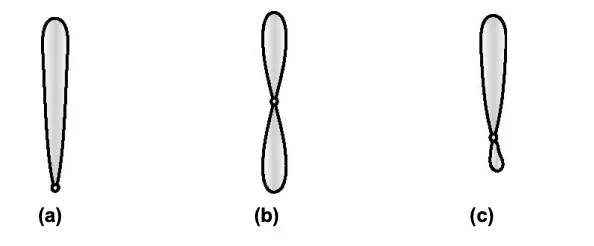
Chromosome Behavior
- Meiotic Analysis:
- Observing chromosome pairing and behavior during meiosis helps in understanding fertility and genetic relationships. This analysis aids in comparing genomes across species.
- Polyploidy and Genome Analysis:
- Genome Nature:
- Analyzing whether a genome is homozygous or heterozygous provides insights into genetic variation and plant relationships.
Taxonomic evidences from Phytochemistry
Chemotaxonomy is a scientific discipline that employs chemical information to aid in the classification and identification of plant species. This approach leverages the chemical characteristics of plants to delineate taxonomic relationships, complementing traditional morphological methods. The significance of chemotaxonomy arises from its ability to provide stable, unambiguous, and non-variable characters that are essential for accurate plant classification.
Directly Visible Chemical Characters
- Starch Grains: Starch grains are a prime example of directly visible chemical characters. They serve as crucial taxonomic markers due to their distinct shapes and sizes, which are specific to various plant species. Historical studies, such as those by Reichert (1913) and Tateoka (1962), have utilized starch grain morphology to classify plant genera and families, such as distinguishing between the Hordeae tribe in grasses.
- Raphides: These needle-like calcium oxalate crystals are found in certain plant families, notably the Rubiaceae. Their presence or absence aids in classification, such as differentiating between subfamilies within the Rubiaceae or within the Order Scitamineae.
- Druses and Cystoliths: Druses, aggregates of calcium oxalate crystals, and cystoliths, composed of calcium carbonate, are other visible chemical characters. They provide taxonomic insights, particularly within families like Caricaceae and Apocyanaceae, and can be used to differentiate between plant groups based on crystal type.
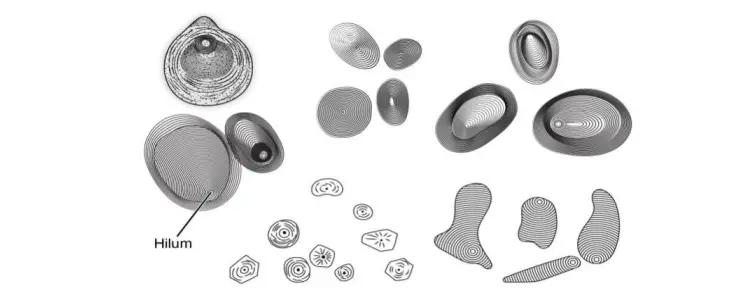
Primary Metabolites
Primary metabolites are essential compounds involved in fundamental biological processes. While they are universally present across plant species, variations in their concentrations can be taxonomically significant.
- Sedoheptulose: This sugar is notably abundant in the genus Sedum. Its presence allows for easy identification of species within this genus, as it is not commonly found in other plants.
- Amino Acids: The 22 amino acids are vital for protein synthesis and can provide taxonomic clues through their varying sequences. Although only a few species have been extensively analyzed, amino acid data supports classification and phylogenetic studies, as demonstrated in research on wheat and barley.
Secondary Metabolites
Secondary metabolites are specialized compounds that are not directly involved in primary metabolic processes but are highly valuable for taxonomic classification due to their restricted occurrence and distinct chemical properties.
- Flavonoids: These phenolic compounds are widespread in plant leaves and exhibit significant structural variability. They have been used extensively for taxonomic classification across various plant families. For instance, the flavonoid profiles of Ulmaceae species reveal distinct subfamilies, Ulmoideae and Celtoideae, based on their flavonoid content.
- Terpenes: These volatile compounds, including monoterpenes, diterpenes, and triterpenes, are prominent in essential oils. They are valuable for distinguishing between plant families and genera. The terpene composition in Salvia species, for example, helps in identifying and classifying these plants.
- Iridoids: These monoterpenoid derivatives are predominantly found in Asterids. Their presence can be used to classify plants within this group.
- Alkaloids: Structurally diverse and derived from amino acids or mevalonic acid, alkaloids are significant in the taxonomy of plant families where they are predominant, such as in the classification of Solanaceae.
- Betalains and Anthocyanins: Betalains, found in Caryophyllales, and anthocyanins, present in many other plants, are mutually exclusive. Their distribution helps in distinguishing between plant groups.
- Glucosinolates: These compounds, characteristic of Brassicaceae and related families, are hydrolyzed to produce mustard oils. Their presence is useful for taxonomic classification within these families.
- Cyanogenic Glycosides: Found in various families, these compounds release hydrogen cyanide upon hydrolysis. Their distribution helps in understanding evolutionary relationships among plant species.
- Polyacetylenes: These non-nitrogenous secondary metabolites are important for classifying related groups of plants, such as those within the Asteraceae and Apiaceae families.
Semantides
Semantides include DNA, RNA, and proteins, which carry genetic information and play a role in chemotaxonomy.
- Proteins: Plant proteins are analyzed using electrophoresis and serological methods to understand taxonomic relationships. For example, studies on wheat proteins support the classification of wheat species based on their protein profiles. Serological studies, based on antibody-antigen reactions, further aid in resolving taxonomic relationships across various plant families.
Taxonomic evidences from Molecular data
Molecular data plays a crucial role in modern taxonomic studies, providing insights into phylogenetic relationships among plant species. This approach primarily focuses on variations in nucleic acids—DNA and RNA—which are pivotal for understanding genetic similarities and differences among plants.
1. Molecular Data Sources
- Nuclear DNA (nDNA)
- Characteristics: Comprises millions to billions of kilobase pairs (kbp), biparentally inherited, and evolves relatively rapidly.
- Significance: Although stable, its complexity and rapid evolution make it challenging to analyze. It provides critical data on evolutionary processes but requires sophisticated methods for study.
- Mitochondrial DNA (mtDNA)
- Characteristics: Typically consists of 200-2500 kbp, uniparentally inherited, and undergoes considerable rearrangement.
- Significance: Due to its variability and complexity, mtDNA is considered less reliable for phylogenetic analysis compared to other DNA types.
- Chloroplast DNA (cpDNA)
- Characteristics: Generally 120-140 kbp, smallest among the three DNA types, and is uniparentally inherited.
- Advantages for Taxonomy:
- Smaller Size: Facilitates easier isolation and analysis.
- Abundance: Multiple chloroplasts per cell enhance data reliability.
- Stability: Less prone to change compared to nuclear DNA.
- Consistency: Despite variations, cpDNA carries a consistent set of genes across species, making it a stable marker for comparison.
2. Applications in Phylogenetic Analysis
Molecular data, particularly from cpDNA, is used to construct phylogenetic trees and understand evolutionary relationships at various taxonomic levels. This approach allows taxonomists to establish connections both within and between plant families.
- Phylogenetic Trees: Constructed using molecular data to depict evolutionary relationships. For instance:
- Monophyly: Molecular evidence supports the monophyly of families such as Apiaceae, Caprifoliaceae, Plantaginaceae, Scrophulariaceae, Apocynaceae, and Saxifragaceae.
- Family Unions: Molecular data has led to the merging of certain families, such as Apocynaceae with Asclepiadaceae, and Brassicaceae with Capparaceae.
3. Integration with Classical Taxonomy
While molecular data provides significant insights, it is essential to integrate these findings with classical taxonomic methods. Traditional morphological and anatomical evidence continues to be valuable for a comprehensive understanding of plant relationships. Thus, a holistic approach that combines molecular data with classical taxonomy is crucial for accurate phylogenetic analysis.