Wha is Stomata?
- Stomata are microscopic pores located primarily on the epidermis of plant leaves, with occasional presence in stems and other organs. The term “stomata” is derived from the Greek word “stoma,” meaning “mouth,” reflecting their function in gas exchange. A single pore is referred to as a stoma. These structures play a crucial role in the physiological processes of plants, including photosynthesis and transpiration.
- Each stoma is surrounded by two specialized cells known as guard cells. These guard cells are essential for regulating the size of the stomatal opening. By expanding or contracting, guard cells control the aperture of the stomata, thus modulating the exchange of gases. When the guard cells are turgid, they cause the stomata to open, allowing carbon dioxide to enter for photosynthesis and enabling the release of oxygen. Conversely, when the guard cells lose turgidity, the stomata close, reducing water loss and preventing excessive transpiration.
- Stomatal distribution varies among plant species. In many dicotyledons, stomata are predominantly found on the abaxial (lower) leaf surface, while in some monocotyledons, such as corn and onions, they can be evenly distributed on both surfaces. Some plants exhibit adaptations based on their environment; for example, species with floating leaves may have stomata only on the upper surface, while submerged leaves may lack stomata entirely. This variation in stomatal arrangement is significant for optimizing gas exchange in different habitats.
- The number of stomata can vary widely across species, with sizes typically ranging from 10 to 80 micrometers in length and widths from a few to 50 micrometers. Stomata are classified based on their position: amphistomatous leaves have stomata on both surfaces, hypostomatous leaves possess stomata solely on the lower surface, and epistomatous leaves have stomata only on the upper surface. These classifications indicate the adaptive strategies plants employ to optimize gas exchange while minimizing water loss.
- Stomata are vital for maintaining the plant’s internal environment and overall health. They facilitate the diffusion of gases—carbon dioxide for photosynthesis and oxygen for respiration—between the internal air spaces of the leaf and the external atmosphere. Additionally, through the process of transpiration, stomata contribute to the regulation of water vapor loss, which helps in nutrient uptake and temperature regulation within the plant.
Definition of Stomata
Stomata are tiny pores located on the epidermis of plant leaves and stems that regulate gas exchange, allowing carbon dioxide, oxygen, and water vapor to enter and exit the plant. Each stoma is flanked by guard cells that control its opening and closing, facilitating processes such as photosynthesis and transpiration.
Structure of Stoma
Stomata are intricate structures critical for gas exchange in plants, comprising several specialized components. The design of stomata allows for the regulation of carbon dioxide, oxygen, and water vapor movement, facilitating essential processes such as photosynthesis and transpiration. The structural characteristics of a stoma include:
- Shape: Stomata typically exhibit an elliptical shape, although variations exist among different plant species, reflecting adaptations to their environments.
- Size: When fully opened, a stoma generally measures between 3 to 12 micrometers in width and 10 to 40 micrometers in length, enabling effective gas exchange.
- Guard Cells: Each stoma is flanked by a pair of guard cells, which are responsible for regulating the size of the stomatal opening. In dicots, these guard cells often have a kidney shape, while in monocots, they tend to be dumbbell-shaped. The inner wall of the guard cells is thickened and inelastic, providing structural integrity, while the outer wall is thin, flexible, and semi-permeable. This structural distinction enables the guard cells to swell or shrink in response to osmotic changes, thus opening or closing the stomatal pore.
- Cellular Composition: Guard cells contain a single nucleus and several chloroplasts, although these chloroplasts lack the enzyme Rubisco, resulting in limited photosynthetic capability. This is significant, as it emphasizes their primary function in gas regulation rather than photosynthesis.
- Subsidiary Cells: Adjacent to the guard cells are subsidiary or accessory cells, which play a supportive role in the functioning of the guard cells. These cells, also modified epidermal cells, lack chloroplasts and possess a similar cytoplasmic composition, including a large central vacuole and a single nucleus. Their presence assists in the effective movement of guard cells, thereby influencing the overall dynamics of stomatal opening and closing.
- Epidermal Layer: Stomata are located within the epidermis, the outermost layer of plant tissue composed of irregularly shaped cells that provide mechanical support. The epidermal cells also play a role in protecting the plant from environmental stressors.
Types of Stomata
Stomata can be classified into various types based on their location, development, and structural characteristics. This classification is essential for understanding the diverse adaptations in different plant species, reflecting their ecological niches and evolutionary paths.
Classification Based on Location
- Epistomatic: Stomata in this category are located on the upper surface of the leaf. An example of an epistomatic plant is the waterlily.
- Heterostomatic: In heterostomatic plants, stomata are predominantly found on the lower surface of the leaf. Common examples include potato and cabbage.
- Isostomatic: This type features stomata distributed equally on both the upper and lower surfaces of the leaf. Oats and many grasses represent isostomatic plants.
- Astomatic: Astomatic plants lack stomata entirely, both on the upper and lower leaf surfaces. Examples include submerged aquatic plants like Potamogeton.
Classification Based on Development
- Mesogynous: In mesogynous development, both guard cells and accessory cells originate from similar mother cells. This type is found in members of the Brassicaceae family.
- Perigynous: Here, guard cells and accessory cells develop from different mother cells, with guard cells arising from one cell and accessory cells from neighboring ones. Plants in the Cucurbitiaceae family exhibit this type of development.
- Mesoperigynous: This type combines characteristics of both mesogynous and perigynous types. In mesoperigynous stomata, the guard cells and one accessory cell develop from a single mother cell, while other accessory cells may arise independently from adjacent cells. Members of the Brassicaceae family can also display this type.
Classification Based on Structure
- Anomocytic (Irregular-celled): These stomata have guard cells surrounded by a few subsidiary cells that are similar in size, shape, and arrangement to surrounding epidermal cells. This type is common in the families Ranunculaceae and Malvaceae.
- Anisocytic (Unequal-celled): Anisocytic stomata feature guard cells that are flanked by three subsidiary cells of unequal sizes, with one being significantly smaller. This type is prevalent in the Brassicaceae and Solanaceae families.
- Paracytic (Parallel-celled): In paracytic stomata, one or more subsidiary cells lie parallel to the guard cells. These stomata can be found in numerous dicot families, including Rubiaceae and Convolvulaceae.
- Diacytic (Cross-celled): Diacytic stomata are characterized by guard cells that are surrounded by two subsidiary cells, which contact each other opposite the middle of the opening. This structure can be observed in Caryophyllaceae and Acanthaceae families.
- Actinocytic (Star-celled): This rare type consists of guard cells encircled by at least five radiating subsidiary cells, forming a star-like arrangement. Members of the Ebenaceae family may exhibit actinocytic stomata.
- Cyclocytic (Ring-celled): Cyclocytic stomata are characterized by four or more subsidiary cells that form a narrow ring around the guard cells. Examples include plants from the Palmae and Cyclanthaceae families.
- Gramineous (Grass-like): Gramineous stomata have guard cells surrounded by two lens-shaped accessory cells, with the guard cells narrower in the middle and bulbous at both ends. This structure is typical of monocot families such as Poaceae and Cyperaceae.
Opening and Closing of Stomata
The opening and closing of stomata are vital physiological processes that regulate gas exchange in plants. Mediated by two guard cells, these processes are essential for maintaining the plant’s water balance and ensuring access to carbon dioxide (CO₂). The dynamics of stomatal movement depend heavily on the solute concentration and water potential within the guard cells.
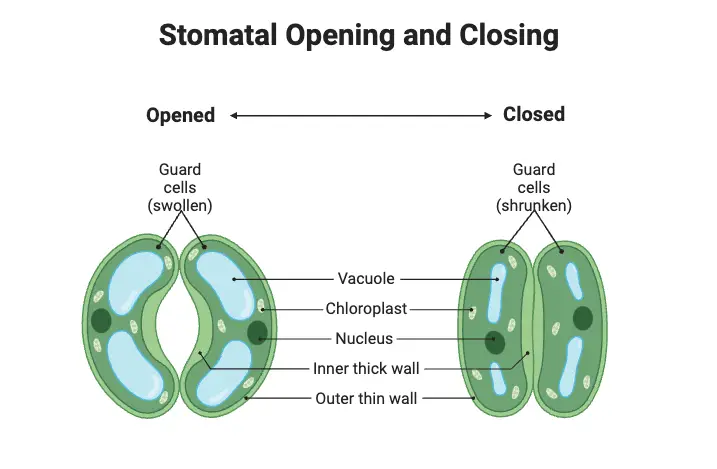
Mechanisms of Stomatal Opening
- High Water Potential: Stomata open when guard cells have a high water potential. This is achieved through osmotic movement, where solutes from the surrounding environment move into the guard cells. The influx of solutes causes the guard cells to become turgid, resulting in swelling that opens the stomatal pore.
- Gas Exchange: When the stomatal pore is open, CO₂ can enter the leaf for photosynthesis, while water vapor and oxygen can diffuse out. This gas exchange is crucial for the plant’s metabolic processes.
- Proton Pump Activity: Under favorable conditions, such as high light intensity and humidity, the proton pump expels protons (H⁺) from the guard cells. This activity increases the negative electrical potential within the cells, allowing potassium ions (K⁺) to enter. The influx of K⁺, often balanced by chloride ions (Cl⁻), increases the turgor pressure and cell volume, leading to the elongation of guard cells. This elongation, constrained by cellulose microfibrils, creates an open pore for gas diffusion.
Mechanisms of Stomatal Closing
- Low Water Potential: Stomata close when guard cells experience low water potential. This situation arises when there is an osmotic movement of solutes from the guard cells to the surrounding areas, causing the cells to become flaccid. As the guard cells shrink, the stomatal pore closes to prevent water loss.
- Abscisic Acid (ABA) Signaling: In response to water scarcity, the roots release abscisic acid (ABA). ABA binds to receptor proteins in the guard cells, triggering a cascade of intracellular changes. Initially, this process raises the pH within the guard cells and increases the concentration of free calcium ions (Ca²⁺) in the cytosol.
- Ion Flux Changes: The increase in cytosolic Ca²⁺ causes the efflux of chloride (Cl⁻) and organic ions from the guard cells. Simultaneously, the uptake of potassium ions (K⁺) is halted, leading to the loss of K⁺ from the cells. As a result, the solute concentration decreases, which raises the water potential and causes water to diffuse out of the cells. This plasmolysis of the guard cells results in the closure of the stomatal pores.
Influencing Factors
Several environmental factors affect stomatal movement:
- Factors Causing Opening: Conditions such as high water concentration, elevated CO₂ levels, and high temperatures generally promote stomatal opening.
- Factors Causing Closing: Conversely, low temperatures, insufficient light, and mechanical stress can trigger the closing of stomata, thereby conserving water and regulating gas exchange effectively.
What are Guard Cells?
Guard cells are specialized cells located in the epidermis of leaves, crucial for regulating gas exchange and water loss in plants. These cells flank each stoma, the opening through which gases pass, and their ability to change shape enables them to control the stomatal pore’s size. The functionality of guard cells is vital for the overall health and efficiency of the plant’s physiological processes.
- Structure of Guard Cells:
- Shape and Arrangement: Guard cells exhibit distinct shapes based on the type of plant. In dicots, such as Arabidopsis thaliana, they are typically kidney-shaped, while in monocots, like grasses, they adopt a dumbbell shape. This morphological specialization allows them to effectively regulate the stomatal aperture.
- Cell Wall Composition: The walls of guard cells are made up of cellulose, pectins, and mixed-linkage glucans. This composition imparts strength and elasticity, with inner walls adjacent to the stomatal pore being thicker and more rigid, while the outer walls are thinner, allowing for flexibility during turgor changes.
- Chloroplasts: Guard cells contain chloroplasts, enabling them to perform photosynthesis. This capability contributes to the production of sugars that are crucial for creating the osmotic pressure changes necessary for the function of stomata.
- Function of Guard Cells:
- Gas Exchange: Guard cells play a direct role in facilitating the uptake of carbon dioxide (CO₂) for photosynthesis while also allowing for the release of oxygen (O₂). This exchange is essential for the plant’s energy production and overall growth.
- Water Regulation: Through the mechanism of opening and closing stomata, guard cells minimize water loss during transpiration. This regulation is particularly important under drought conditions, where conserving water is vital for survival.
- Mechanism of Opening and Closing Stomata: The dynamics of stomatal opening and closure are governed by changes in turgor pressure within the guard cells, influenced by the movement of ions and water.
- Stomatal Opening:
- Stimuli: Environmental factors such as light, carbon dioxide concentration, and humidity serve as triggers for stomatal opening. For instance, light activates photoreceptors in guard cells, initiating signaling cascades that promote stomatal opening.
- Ion Uptake: In response to these stimuli, guard cells actively take up potassium ions (K+) and chloride ions (Cl−) from adjacent cells, leading to an increase in solute concentration within the guard cells.
- Water Influx: The heightened solute concentration lowers the water potential inside the guard cells, causing water to enter through osmosis. As the guard cells swell and become turgid, they bend outward due to their asymmetric wall structure, thus opening the stomatal pore.
- Stomatal Closure:
- Loss of Water: In conditions of high temperatures or drought, guard cells lose potassium ions back to neighboring cells, which decreases the solute concentration within the guard cells.
- Water Exit: As solutes exit, water follows, leading to a reduction in turgor pressure. Consequently, guard cells become flaccid and collapse inward, closing the stomatal pore.
- Stomatal Opening:
Describe the role of abscisic acid in the closure of stomata during times of water stress, including the role of calcium ions as a second messenger
Abscisic acid (ABA) plays a pivotal role in the closure of stomata during periods of water stress, primarily by influencing ion movements and signaling pathways within guard cells. When a plant experiences water deficiency, ABA is synthesized and released into the plant tissues, triggering physiological responses that lead to stomatal closure.
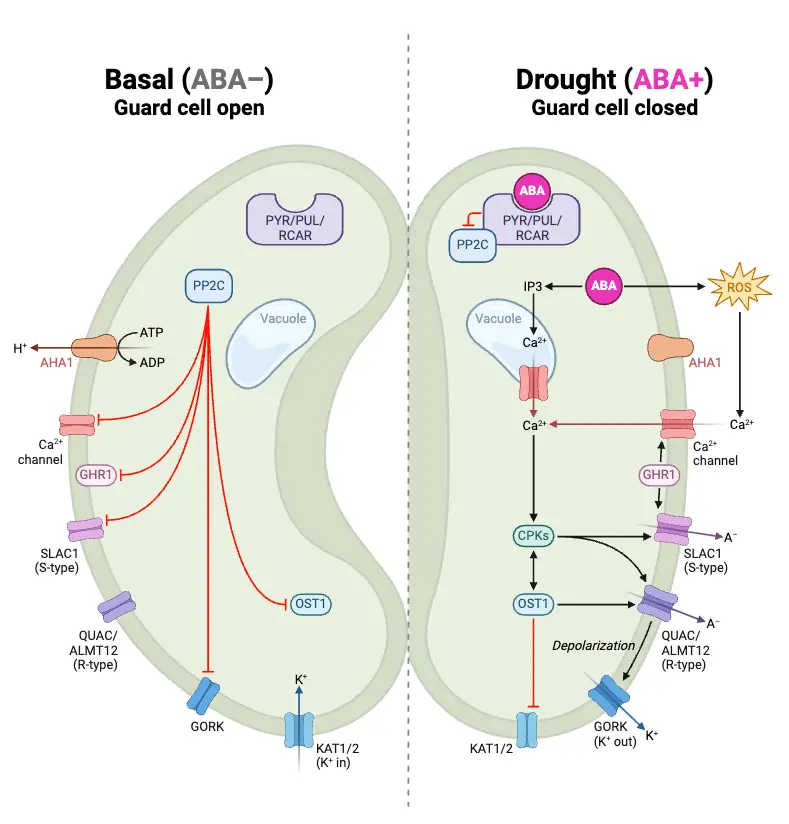
Role of Abscisic Acid in Stomatal Closure
- Stomatal Response to Water Stress: Under conditions of drought or water stress, the concentration of ABA increases significantly. This hormone acts as a signal to initiate stomatal closure, thereby reducing water loss through transpiration. The presence of ABA enhances the sensitivity of guard cells to environmental cues that promote closure, such as high concentrations of calcium ions (Ca²⁺).
- Activation of Ion Channels: ABA promotes the activation of specific ion channels in guard cells, particularly anion channels like SLAC1 (Slow Anion Channel 1). The activation of these channels facilitates the efflux of potassium (K⁺) and chloride (Cl⁻) ions from guard cells. As K⁺ ions exit the cells, water follows osmotically, leading to a decrease in turgor pressure within the guard cells and resulting in stomatal closure.
Role of Calcium Ions as a Second Messenger
- Calcium Signaling: Calcium ions serve as a crucial second messenger in the signaling pathway initiated by ABA. When ABA binds to its receptors on guard cells, it triggers an increase in intracellular Ca²⁺ concentration. This rise in Ca²⁺ levels activates calcium-dependent protein kinases (CPKs), which are essential for further signaling events that lead to stomatal closure.
- Interaction with SLAC1: The elevated Ca²⁺ concentration enhances the activation of SLAC1 channels. CPKs phosphorylate proteins that facilitate the opening of these anion channels, allowing for the efflux of Cl⁻ and malate ions from guard cells. This ion release causes depolarization of the cell membrane, which further activates potassium efflux channels, reinforcing the loss of K⁺ ions and promoting cell shrinkage.
- Regulation by Protein Phosphatases: ABA also inhibits protein phosphatases (PP2Cs) that negatively regulate CPK activity. By inhibiting these phosphatases, ABA ensures that CPKs remain active, thus maintaining high levels of Ca²⁺ signaling necessary for effective stomatal closure.
- Dual Pathways: Research indicates that there may be both Ca²⁺-dependent and Ca²⁺-independent pathways involved in ABA-induced stomatal closure. While Ca²⁺ is vital for accelerating closure and enhancing responsiveness to ABA, some studies suggest that stomatal closure can occur even without significant Ca²⁺ elevation, indicating a complex interplay between different signaling mechanisms.
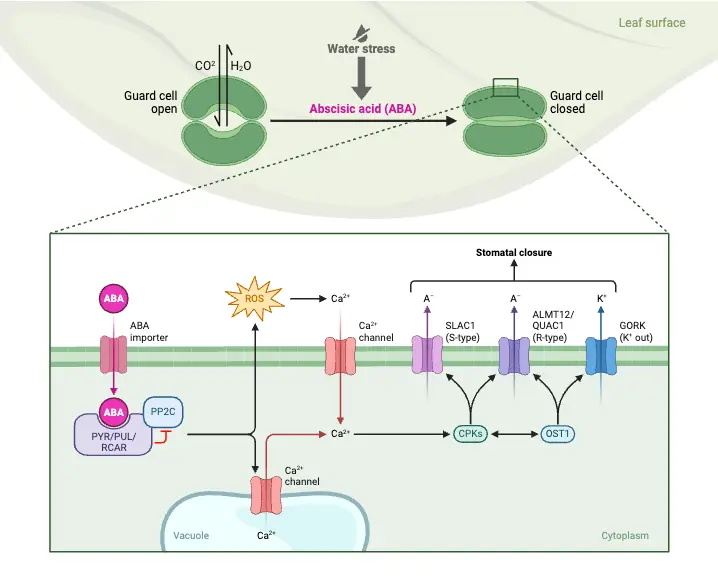
Explain that stomata have daily rhythms of opening and closing
Stomata exhibit daily rhythms of opening and closing, a phenomenon driven by various environmental and internal cues. This rhythmic behavior is crucial for optimizing gas exchange and water conservation in plants.
Daily Rhythms of Stomatal Opening and Closing
- Circadian Regulation: Stomata typically open during the day and close at night, following a circadian rhythm that aligns with the light-dark cycle. This pattern allows plants to maximize carbon dioxide uptake during photosynthesis while minimizing water loss when light is absent. Interestingly, this rhythm persists even in the absence of light, indicating an internal biological clock that regulates stomatal behavior independent of external light conditions.
- Environmental Influences: The opening of stomata during daylight hours facilitates the inward diffusion of carbon dioxide necessary for photosynthesis and the outward diffusion of oxygen and water vapor. Conversely, stomatal closure at night helps conserve water by reducing transpiration rates when photosynthesis cannot occur.
- Physiological Responses: Various environmental factors can influence stomatal movements:
- Light: Stomata generally open in response to light, which triggers photosynthesis.
- Humidity and Temperature: High humidity and moderate temperatures promote stomatal opening, while low humidity and high temperatures can lead to closure to prevent excessive water loss.
- Water Availability: In times of water stress, plants may close their stomata to conserve moisture, a response mediated by hormones like abscisic acid (ABA).
- Mechanism of Action: The rhythmic opening and closing of stomata are primarily regulated by changes in turgor pressure within guard cells. When guard cells take up potassium ions (K+), they become turgid, causing the stomatal pore to open. Conversely, when K+ ions exit the guard cells, they lose turgor pressure and become flaccid, leading to stomatal closure.
Functions of Stomata
Stomata are microscopic openings found primarily on the leaves of plants, playing a pivotal role in the plant’s interaction with its environment. They facilitate essential processes such as gas exchange, transpiration, and moisture regulation, all of which are vital for the plant’s survival and growth.
- Gaseous Exchange: The primary function of stomata is to enable the exchange of gases between the plant and the atmosphere. They allow for the uptake of carbon dioxide (CO₂), which is crucial for photosynthesis, while simultaneously facilitating the release of oxygen (O₂) as a byproduct. This process is fundamental for plant metabolism and energy production.
- Transpiration: Stomata are instrumental in transpiration, a process that involves the removal of excess water from the plant in the form of water vapor (H₂O). This process not only aids in nutrient transport but also plays a critical role in regulating the plant’s temperature. As water vapor escapes, it cools the plant, helping to prevent overheating.
- Moisture Regulation: Stomata help maintain moisture balance within the plant by opening and closing in response to environmental conditions. During dry or hot weather, stomata can close to reduce water loss, effectively conserving moisture. Conversely, they can open when conditions are more favorable, allowing for gas exchange and transpiration to occur.
- Respiration: In addition to their role in photosynthesis, stomata facilitate respiration by allowing oxygen to enter the plant and carbon dioxide to exit. This exchange is vital for cellular respiration, where the plant breaks down glucose to release energy.
- Defense Mechanism: The ability of stomata to close also serves as a protective mechanism against pathogens and pests. By limiting the entry points into the plant, stomatal closure can help mitigate the risk of infection or damage.
- Nutrient Uptake: Stomata can contribute to the uptake of essential minerals, such as potassium, through ion exchange processes. This function, while secondary to gas exchange, highlights the diverse roles stomata play in plant health and nutrient management.
- Nighttime Closure: The closure of stomata at night is crucial for conserving water. By preventing water vapor loss during the cooler, darker hours, plants can maintain hydration levels essential for their physiological processes.
- Shtein, I., & others. (2018). Balancing strength and flexibility: How the synthesis, organization, and modification of guard cell walls govern stomatal development and dynamics. Frontiers in Plant Science, 9, 1202. https://www.frontiersin.org/articles/10.3389/fpls.2018.01202/full
- Lawson, T., Oxborough, K., Morison, J. I. L., & Baker, N. R. (2002). Photosynthetic electron transport is involved in guard cell signaling for stomatal closure. Plant Physiology, 158(2), 1360-1370. https://pmc.ncbi.nlm.nih.gov/articles/PMC6589527/
- AskNature. (n.d.). How guard cells function — Biological strategy. Retrieved from https://asknature.org/strategy/guard-cells-regulate-gas-and-moisture-exchange/
- Wikipedia contributors. (2024). Guard cell. In Wikipedia, The Free Encyclopedia. Retrieved from https://en.wikipedia.org/wiki/Guard_cell
- Superprof. (n.d.). What role stomata plays in homeostasis in plants? Retrieved from https://www.superprof.co.uk/resources/academic/academic-science/biology-science/biology-a-level/homeostasis-in-plants.html
- Westgeest, A. J., Dauzat, M., Simonneau, T., & Pantin, F. (2023). Leaf starch metabolism sets the phase of stomatal rhythm. The Plant Cell, koad158. https://doi.org/10.1093/plcell/koad158
Loftfield, J. V. G. (1921). The behavior of stomata. Carnegie Institution of Washington, Publication No. 314. - BYJU’S. (n.d.). Stomata- Structure, functions, types & mechanism of stomata. Retrieved from https://byjus.com/biology/stomata/
- https://biologyreader.com/stomata-in-plants.html
- https://plantlet.org/stomata-structure-types-and-functions/
- https://www.tutoroot.com/blog/what-is-stomata-definition-structure-types/
- https://www.sciencefacts.net/stomata.html
- https://www.geeksforgeeks.org/stomata/
- https://en.wikipedia.org/wiki/Stoma
- https://byjus.com/biology/stomata
- https://www.plantscience4u.com/2014/04/types-of-stomata.html#google_vignette