What is Phytochrome?
- Phytochromes are specialized photoreceptor proteins predominantly found in plants, but also present in bacteria and fungi. These proteins are essential for detecting light in the red and far-red regions of the visible spectrum. Phytochromes can be categorized into two main types based on their light activation: Type I phytochromes respond to far-red light, whereas Type II phytochromes are activated by red light.
- Recent research indicates that phytochromes also function as temperature sensors. Elevated temperatures are known to accelerate the deactivation of phytochromes, influencing their role in plant responses to environmental conditions. This additional function contributes to the regulation of seed germination, highlighting the complex interplay between light and temperature in plant development.
- Phytochromes play a crucial role in controlling various aspects of plant growth and development. They are involved in photoblasty, the process by which seeds detect light and initiate germination. Phytochromes also regulate chlorophyll synthesis, which is vital for photosynthesis. Furthermore, they influence the elongation of seedlings, the morphology and movement of leaves, and the timing of flowering in mature plants. Their expression spans multiple tissues and developmental stages, underscoring their integral role in plant physiology.
- In addition to phytochromes, plants possess other photoreceptors such as cryptochromes and phototropins. Cryptochromes respond to blue and ultraviolet-A light, while phototropins also detect blue light but primarily affect phototropic responses, such as the bending of plants toward light. UVR8 is another photoreceptor sensitive to ultraviolet-B light, further illustrating the diversity of light-responsive mechanisms in plants. These photoreceptors collectively enable plants to adapt to their light environment effectively.
Structure of Phytochrome
Phytochrome is a complex protein pigment that plays a crucial role in plant photoreception and signaling. Here are the key aspects of its structure:
- Protein Composition: Phytochrome is a homodimer, meaning it consists of two identical subunits. Each subunit has a molecular mass of approximately 125 kDa.
- Chromophore: The active form of phytochrome contains a chromophore, which is a light-absorbing molecule. This chromophore is derived from phytochromobilin, a linear tetrapyrrole, and is responsible for the absorption of red and far-red light.
- Two Forms: Phytochrome exists in two interconvertible forms:
- Pr (red light-absorbing form): This form absorbs red light (around 660 nm) and is typically found in dark-grown or etiolated plants.
- Pfr (far-red light-absorbing form): This form absorbs far-red light (around 730 nm) and is produced when Pr is exposed to red light. Pfr is the active form that initiates various physiological responses in plants.
- Conformational Changes: Upon light absorption, phytochrome undergoes significant conformational changes. The transition from Pr to Pfr involves a structural rearrangement that activates the signaling pathways associated with light responses.
- Functional Domains: Phytochrome contains several functional domains that are involved in its signaling capabilities. These domains interact with various components of signal transduction pathways, leading to changes in gene expression and physiological responses.
- Gene Family: The phytochrome gene family consists of multiple members, each encoding different types of phytochromes that regulate distinct processes in photomorphogenesis.
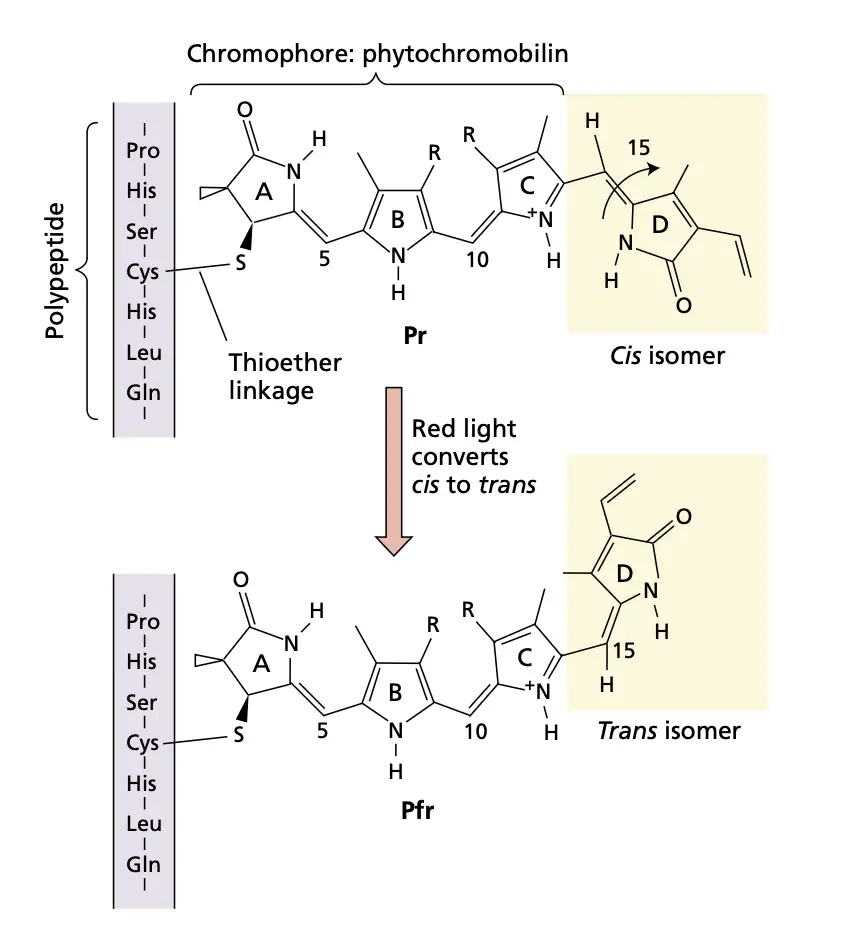
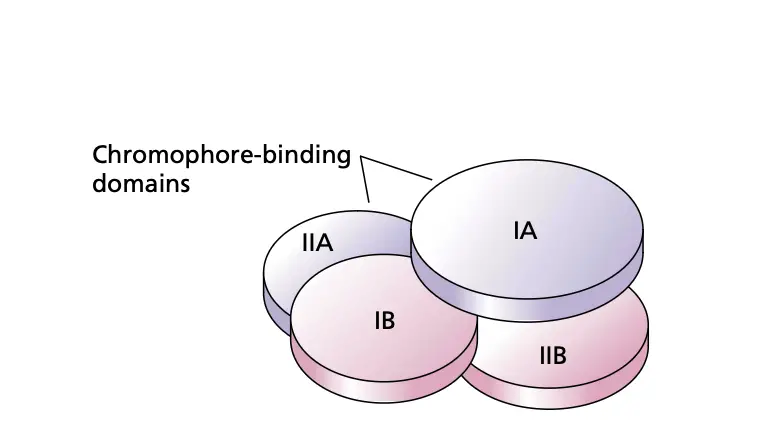
Types of Phytochrome
Phytochromes are classified into different types based on their genetic encoding and functional roles in plants. The main types of phytochrome include:
- Phytochrome A (phyA):
- Type: Type I phytochrome.
- Function: Primarily involved in the very low fluence response (VLFR) and plays a crucial role in seed germination and de-etiolation. It is sensitive to red light and is essential for detecting far-red light.
- Characteristics: PhyA is unique in that it is transcriptionally active in dark-grown seedlings but its expression is inhibited in light, particularly in monocots.
- Phytochrome B (phyB):
- Type: Type II phytochrome.
- Function: Mediates a wide range of light responses, including shade avoidance, flowering time regulation, and photomorphogenesis. It is the primary photoreceptor for red light responses.
- Characteristics: PhyB is known for its role in the red/far-red light signaling pathway and is involved in the regulation of gene expression in response to light
- Phytochrome C (phyC):
- Type: Type II phytochrome.
- Function: While its specific roles are less well-defined than those of phyA and phyB, phyC is believed to have overlapping functions with phyB, particularly in regulating light responses and development.
- Characteristics: PhyC is involved in the shade avoidance response and may act redundantly with other phytochromes .
- Phytochrome D (phyD):
- Type: Type II phytochrome.
- Function: Similar to phyB, phyD is involved in mediating shade avoidance responses and may also influence flowering time and leaf petiole elongation.
- Characteristics: PhyD acts redundantly with phyB and phyE in various developmental processes.
- Phytochrome E (phyE):
- Type: Type II phytochrome.
- Function: PhyE is involved in the regulation of light responses, particularly in the context of shade avoidance and flowering time. It acts redundantly with phyB and phyD.
- Characteristics: Recent studies suggest that phyE may also play a role in the inhibition of internode elongation
The photochemical and biochemical properties of phytochrome
The photochemical and biochemical properties of phytochrome are fundamental to its role as a light receptor in plants. Here are the key aspects of these properties:
- Structure and Composition:
- Phytochrome is a chromoprotein, meaning it consists of a protein component and a chromophore, which is the light-absorbing part. The chromophore is typically a linear tetrapyrrole known as phytochromobilin, which is derived from biliverdin.
- Phytochrome exists as a homodimer, composed of two identical subunits, and has a molecular mass of approximately 125 kDa. This dimerization is crucial for its function and stability.
- Photoconversion:
- Phytochrome can exist in two interconvertible forms: the inactive form (Pr) and the active form (Pfr). The Pr form absorbs red light (around 660 nm) and converts to the Pfr form upon light absorption. Conversely, the Pfr form can be converted back to Pr by far-red light (around 730 nm).
- This reversible photoconversion is essential for phytochrome’s role in mediating light responses, as the Pfr form is the biologically active state that initiates signal transduction pathways.
- Light Absorption and Action Spectra:
- Phytochromes have distinct absorption spectra, with the Pr form absorbing red light and the Pfr form absorbing far-red light. This property allows plants to detect changes in light quality, such as the R:FR ratio, which is important for shade avoidance and other growth responses 26.
- The action spectra of phytochrome responses vary depending on the specific physiological process being regulated, such as germination, flowering, or phototropism.
- Signal Transduction Mechanisms:
- Upon conversion to the Pfr form, phytochrome translocates to the nucleus, where it interacts with various transcription factors and regulatory proteins. This interaction leads to changes in gene expression that drive physiological responses.
- The signaling pathways activated by phytochrome involve a complex network of proteins, including kinases, transcription factors, and other signaling molecules, which mediate the plant’s response to light.
- Regulation of Gene Expression:
- Phytochrome regulates the transcription of numerous genes involved in various processes, including greening, flowering, and shade avoidance. For example, it promotes the expression of genes like FT (FLOWERING LOCUS T), which is crucial for flowering induction.
- Phytochrome can also repress the transcription of certain genes, demonstrating its role in both activation and inhibition of gene expression depending on the light conditions and the specific developmental context.
- Physiological Responses:
- The biochemical properties of phytochrome enable it to mediate a wide range of physiological responses, including seed germination, stem elongation, leaf expansion, and flowering. These responses can be classified into rapid biochemical events and slower morphological changes 8.
- Rapid responses may include changes in ion fluxes and membrane potential, while slower responses involve alterations in growth patterns and developmental processes.
Localization of Phytochrome in Tissues and Cells
Understanding the distribution of phytochrome within plant tissues and cells provides crucial insights into its functional roles. This section outlines how phytochrome localization has been studied and what these studies reveal about its role in plant biology.
Detection of Phytochrome in Plant Tissues
- Spectrophotometric Detection:
- Phytochrome can be quantified in plant tissues using a spectrophotometer. This technique leverages the pigment’s unique photoreversible properties.
- In green tissues, detecting phytochrome is challenging due to the masking effect of chlorophyll. Consequently, its presence is more readily observed in dark-grown plants devoid of chlorophyll.
- Phytochrome is found in various plant groups, including angiosperms, gymnosperms, ferns, mosses, and algae.
- Tissue Distribution in Etiolated Seedlings:
- In etiolated seedlings, phytochrome is predominantly located in regions with high meristematic activity or those recently engaged in such processes.
- For example, in pea seedlings, the highest concentrations of phytochrome are found in the bud and first node. In oat coleoptiles, it is concentrated at the tip and node regions.
Differential Expression in Various Tissues
- Gene Expression Analysis:
- Advances in molecular biology have enabled the determination of phytochrome expression patterns using gene cloning techniques.
- By creating fusions of phytochrome genes (e.g., PHYA or PHYB) with reporter genes like β-glucuronidase (GUS), researchers can visually track gene expression.
- In this approach, the promoter of a phytochrome gene is linked to GUS, which produces a color change in response to enzyme activity, indicating gene expression levels in different tissues.
- Findings from Reporter Gene Studies:
- Research using PHYA–GUS and PHYB–GUS fusions in tobacco and Arabidopsis has shown that phytochrome expression is most intense in specific regions such as the apical hook and root tips in dark-grown seedlings.
- In light-grown seedlings, expression levels are lower but still detectable.
- Studies indicate that PHYA is expressed at higher levels than PHYB in all tissues examined.
- Expression Patterns of Type II Phytochromes:
- Recent research comparing PHYB–GUS, PHYD–GUS, and PHYE–GUS fusions in Arabidopsis has demonstrated that Type II phytochromes, though less abundant than Type I, have distinct but overlapping expression patterns.
- These findings suggest that while phytochromes share common sites of action, they also have unique roles in various developmental processes.
Implications of Phytochrome Localization
- Role in Development:
- Phytochromes are most prevalent in young, undifferentiated tissues where significant developmental changes occur. This distribution aligns with their role in regulating growth and development in response to light signals.
- The correlation between high phytochrome levels and dynamic developmental changes highlights their essential role in plant adaptation and growth.
- Functional Cooperation and Distinct Pathways:
- Overlapping expression patterns of different phytochromes suggest cooperative functions. However, each phytochrome might also utilize distinct signal transduction pathways to mediate specific responses.
- This functional diversity is supported by studies on phytochrome mutants, which illustrate the complex regulatory networks involving phytochrome signaling.
Biosynthesis of Phytochrome in Plants
The biosynthesis of phytochrome involves a series of well-coordinated biochemical events that lead to the formation of its functional form, which plays a vital role in photomorphogenesis.
- Apoprotein and Chromophore Synthesis
- Apoprotein Formation: Phytochrome is initially synthesized as an apoprotein through the transcription of nuclear DNA into messenger RNA (mRNA). This mRNA is subsequently translated by 80-S ribosomes in the cytoplasm to produce the phytochrome apoprotein.
- Chromophore Production: The chromophore, known as phytochromobilin, is synthesized within the plastids. After its synthesis, phytochromobilin is released into the cytosol where it associates with the apoprotein. The chromophore cannot absorb light independently; it requires association with the apoprotein to form a functional phytochrome molecule.
- Assembly of Phytochrome
- Bilin Lyase Activity: The assembly of phytochrome involves the covalent attachment of the phytochromobilin chromophore to the apoprotein. This process is facilitated by bilin lyase, which operates through a thioester linkage at the GAF-domain. This combination of apoprotein and chromophore constitutes the holoprotein, which is the complete and functional phytochrome.
- Photoreceptor Properties
- Phytochrome Forms: Phytochrome exists in two interconvertible forms: PR (phytochrome red) and PFR (phytochrome far-red).
- PR (Inactive State):
- Color: Blue-green.
- Nature: Inactive.
- Light-Mediated Response: Does not mediate photoresponses.
- Maximum Light Absorption: Absorbs light primarily in the red region around 680 nm.
- Conformational Change: Converts to PFR upon exposure to red light.
- PFR (Active State):
- Color: Light green.
- Nature: Active.
- Light-Mediated Response: Mediates photomorphogenic responses.
- Maximum Light Absorption: Absorbs light primarily in the far-red region around 730 nm.
- Conformational Change: Converts back to PR upon exposure to far-red light.
- PR (Inactive State):
- Phytochrome Forms: Phytochrome exists in two interconvertible forms: PR (phytochrome red) and PFR (phytochrome far-red).
- Function and Signal Transduction
- Activation and Function: In its active form (PFR), phytochrome acts as a serine-threonine kinase. It phosphorylates serine residues in the hinge region of the protein.
- Signal Transduction: The phosphorylation of the hinge region exposes nucleolar organization signals. This exposure enables PFR to enter the nucleus.
- Stabilization: Within the nucleus, PFR is converted into a more stable form of phytochrome through the action of protein phosphatases, which remove the phosphate groups.
How do phytochromes respond to different light wavelengths?
Phytochromes respond to different light wavelengths through their ability to absorb specific wavelengths of light, leading to conformational changes that activate signaling pathways in plants. Here’s how they respond to various light wavelengths:
- Red Light (660 nm):
- Phytochromes, particularly phytochrome B (phyB), are highly sensitive to red light. When phyB absorbs red light, it converts from the inactive form (Pr) to the active form (Pfr). This conversion triggers a series of physiological responses, including seed germination, de-etiolation, and the promotion of chlorophyll synthesis.
- The activation of phyB by red light is crucial for initiating many light-dependent developmental processes.
- Far-Red Light (730 nm):
- Phytochromes can also absorb far-red light, which causes the active form (Pfr) to revert back to the inactive form (Pr). This process is known as photoreversibility. The ability to switch between these two forms allows plants to respond dynamically to changes in light quality, such as those caused by shading from neighboring plants .
- The conversion of Pfr back to Pr in response to far-red light can inhibit processes like germination and de-etiolation, effectively signaling the plant to adjust its growth strategy in shaded conditions.
- Blue Light:
- While phytochromes primarily respond to red and far-red light, they can also interact with blue light through other photoreceptors, such as cryptochromes. The integration of signals from both phytochromes and cryptochromes allows plants to fine-tune their responses to a broader spectrum of light.
- Light Quality and Ratio:
- Phytochromes are particularly sensitive to the ratio of red to far-red light (R:FR ratio). In natural environments, this ratio changes when plants are shaded by others, as red light is absorbed by chlorophyll in leaves, while far-red light is transmitted. Phytochromes detect these changes and initiate shade avoidance responses, such as elongating stems and petioles to reach for more light.
- Photoperiodism:
- Phytochromes also play a role in photoperiodism, which is the ability of plants to measure day length. This is crucial for regulating flowering time in response to seasonal changes in light availability. Phytochrome B, in particular, is involved in detecting the length of light periods and triggering flowering in long-day or short-day plants
How do phytochrome responses differ among various plant species?
Phytochrome responses can vary significantly among different plant species due to several factors, including genetic differences, ecological adaptations, and the specific roles of various phytochrome types. Here are some key ways in which phytochrome responses differ among plant species:
- Types of Phytochromes:
- Different plant species may express varying combinations of phytochrome types (e.g., phyA, phyB, phyC, phyD, phyE). For instance, some species may rely more heavily on phyA for germination and de-etiolation, while others may depend on phyB for shade avoidance and flowering responses.
- The presence of specific phytochrome types can influence how a species responds to light conditions. For example, some species may have a more pronounced response to red light due to a higher expression of phyB, while others may be more sensitive to far-red light due to phyA.
- Ecological Adaptations:
- Plants that grow in different environments (e.g., shaded forest understories vs. open fields) may exhibit distinct phytochrome responses. Shade-tolerant species often have adaptations that allow them to respond quickly to changes in light quality, such as increased elongation in response to low R:FR ratios, while shade-intolerant species may prioritize rapid growth and light capture.
- For example, species like Arabidopsis thaliana have been extensively studied for their phytochrome responses, showing rapid elongation in shaded conditions, while other species may exhibit different growth patterns or developmental changes.
- Photoperiodic Responses:
- Different species have evolved unique photoperiodic responses mediated by phytochromes. Some plants are classified as long-day plants, which flower in response to longer daylight periods, while others are short-day plants, which flower when days are shorter. The specific phytochrome types involved in these responses can vary among species.
- For instance, Lactuca sativa (lettuce) is a long-day plant that requires specific light conditions to flower, while Xanthium strumarium (cocklebur) is a short-day plant that responds to shorter light periods.
- Developmental Stages:
- The role of phytochromes can also differ depending on the developmental stage of the plant. For example, during seed germination, some species may rely more on phyA, while during vegetative growth, phyB may play a more significant role. This variation can lead to different growth strategies and adaptations among species.
- Genetic and Molecular Mechanisms:
- The genetic regulation of phytochrome signaling pathways can differ among species, leading to variations in how plants respond to light. For example, the downstream signaling components and gene expression patterns activated by phytochromes can vary, resulting in different physiological outcomes .
- Additionally, mutations or variations in phytochrome genes can lead to altered light responses, which can be observed in various plant mutants used in research.
What roles do phytochromes play in plant growth and development?
Phytochromes play several crucial roles in plant growth and development, primarily by acting as light sensors that help plants respond to their light environment. Here are the key roles of phytochromes:
- Regulation of Photomorphogenesis: Phytochromes are essential for photomorphogenesis, which is the process by which plants develop in response to light. They influence various developmental processes, including seed germination, stem elongation, leaf expansion, and flowering.
- Shade Avoidance Response: Phytochromes help plants detect the presence of neighboring plants by sensing the ratio of red to far-red light. When a plant is shaded, the ratio shifts, prompting the plant to elongate its stems and leaves to reach for more light, a phenomenon known as shade avoidance.
- Control of Flowering Time: Phytochromes play a significant role in regulating flowering in response to day length (photoperiod). They help determine the appropriate timing for flowering based on light conditions, which is crucial for reproductive success.
- Seed Germination: Many seeds require light to germinate, and phytochromes are involved in this process. They help initiate germination when the appropriate light signals are detected, ensuring that seeds germinate under favorable conditions.
- Circadian Rhythm Regulation: Phytochromes contribute to the regulation of circadian rhythms in plants, helping them maintain a biological clock that aligns their physiological processes with the day-night cycle. This synchronization optimizes growth and metabolic activities according to light availability.
- Response to Environmental Stress: Phytochromes can mediate plant responses to various environmental stresses, such as drought or high temperatures. By regulating growth patterns and metabolic processes, phytochromes help enhance plant survival under adverse conditions.
- Interaction with Other Signaling Pathways: Phytochromes interact with other signaling pathways, including those involving other photoreceptors (like cryptochromes) and hormonal signals (such as auxins and gibberellins). This interaction allows for a coordinated response to light and environmental changes, integrating multiple signals to optimize plant development.
How do phytochromes influence flowering?
Phytochromes influence flowering primarily through their role in detecting light conditions, particularly the duration of light exposure, which is crucial for determining the timing of flowering in many plants. Here are the key ways in which phytochromes affect flowering:
- Photoperiodism: Phytochromes are essential for photoperiodism, the physiological response of plants to the relative lengths of day and night. They help plants measure the duration of light (day length) and dark periods, which is critical for triggering flowering in response to seasonal changes. Different plants have different photoperiod requirements (short-day, long-day, or day-neutral), and phytochromes play a central role in this process.
- Regulation of Flowering Genes: Phytochromes influence the expression of flowering-related genes. When phytochromes detect the appropriate light conditions, they activate or repress specific genes involved in the flowering process. For example, in long-day plants, exposure to extended light periods leads to the activation of genes such as CONSTANS (CO), which promotes flowering.
- Interaction with Other Photoreceptors: Phytochromes interact with other light-sensitive proteins, such as cryptochromes, to integrate light signals. This interaction helps fine-tune the plant’s response to light and ensures that flowering occurs under optimal conditions.
- Hormonal Regulation: Phytochromes also influence the levels and activity of plant hormones, such as gibberellins and auxins, which are involved in the flowering process. By modulating hormone levels, phytochromes can promote or inhibit flowering depending on the environmental conditions.
- Environmental Cues: In addition to light, phytochromes help plants respond to other environmental cues, such as temperature. For instance, some plants require a period of cold (vernalization) before they can flower. Phytochromes can integrate these signals to ensure that flowering occurs at the right time for successful reproduction.
- Light Quality and R:FR Ratio:
- Phytochromes respond to the ratio of red to far-red light (R:FR ratio), which changes in shaded environments. When plants are shaded, the R:FR ratio decreases, which can signal the plant to initiate flowering as a strategy to escape competition for light.
- The ability of phytochromes to detect these changes allows plants to adapt their flowering time to optimize reproductive success in their specific environments.
- Signal Transduction Pathways:
- Upon activation by light, phytochromes undergo a conformational change from the inactive form (Pr) to the active form (Pfr). This active form translocates to the nucleus, where it interacts with various transcription factors and regulatory proteins to modulate the expression of flowering-related genes.
- For example, phytochrome signaling can influence the expression of genes such as FT (FLOWERING LOCUS T), which is a key integrator of flowering signals. The activation of FT leads to the promotion of flowering by triggering the development of floral meristems.
- Interaction with Other Hormones:
- Phytochromes also interact with plant hormones, such as gibberellins and auxins, which play roles in flowering. The balance between these hormones can be influenced by light conditions detected by phytochromes, further affecting the timing and development of flowers.
- For instance, gibberellins are known to promote flowering in some species, and their synthesis can be regulated by phytochrome signaling pathways.
- Genetic Regulation:
- The genetic pathways regulated by phytochromes involve a complex network of genes that control flowering time. Mutations in phytochrome genes or their signaling components can lead to altered flowering responses, demonstrating the importance of phytochromes in this process.
- Research on various plant mutants has shown how different phytochrome types contribute to the regulation of flowering, highlighting the redundancy and specificity of these pathways in different species.
How do phytochromes undergo photoconversion?
Phytochromes undergo photoconversion through a process that involves the reversible transformation between two distinct forms: the red-light-absorbing form (P_r) and the far-red-light-absorbing form (P_fr). This photoconversion is central to the function of phytochromes as light sensors in plants. Here’s a detailed overview of the process:
- Structure and Chromophore: Phytochromes are biliproteins that contain a covalently attached chromophore, typically a bilin (such as phytochromobilin). The chromophore is responsible for absorbing light and undergoing structural changes during photoconversion.
- Absorption of Light: When phytochromes absorb red light (around 660 nm), the P_r form is converted to the P_fr form. This process involves the isomerization of the chromophore, where the double bonds in the chromophore change configuration, leading to a structural rearrangement of the entire phytochrome molecule .
- Photoconversion Mechanism: The photoconversion process can be summarized as follows:
- Red Light Absorption: The P_r form absorbs red light, causing the chromophore to undergo a conformational change, resulting in the formation of the P_fr form.
- Far-Red Light Absorption: Conversely, when the P_fr form absorbs far-red light (around 730 nm), it reverts back to the P_r form. This reversibility is a key feature of phytochromes and allows them to respond dynamically to changes in light conditions.
- Thermal Reversion: In addition to light-induced conversion, phytochromes also undergo a thermal reversion process. The P_fr form can slowly revert to the P_r form in the absence of light, a process known as dark reversion. This intrinsic property allows phytochromes to reset and be ready for subsequent light signals.
- Signal Transduction: The conversion between P_r and P_fr forms is not just a physical change; it also triggers a series of biochemical signaling pathways within the plant. The P_fr form is typically the biologically active form that initiates downstream signaling events, leading to various physiological responses, such as changes in gene expression and developmental processes.
- Role of Structural Changes: The structural changes that occur during photoconversion are crucial for the activation of signaling pathways. The conformational changes in the phytochrome protein upon conversion can expose or hide specific interaction sites for downstream signaling partners, thereby influencing the plant’s response to light.
What is the significance of the chromophore in phytochrome function?
The chromophore in phytochrome function is of paramount significance for several reasons:
- Light Absorption: The chromophore, typically a bilin such as phytochromobilin, is responsible for the absorption of specific wavelengths of light. Phytochromes can absorb red light (around 660 nm) and far-red light (around 730 nm), which are critical for their role as photoreceptors. The ability to absorb these wavelengths allows phytochromes to detect changes in light conditions, which is essential for plant growth and development.
- Photoconversion: The chromophore undergoes structural changes during photoconversion, transitioning between the red-light-absorbing form (P_r) and the far-red-light-absorbing form (P_fr). This isomerization is crucial for the function of phytochromes, as it triggers the activation of signaling pathways that lead to various physiological responses in plants, such as flowering, seed germination, and shade avoidance.
- Signal Transduction: The conformational changes in the chromophore upon light absorption are linked to the activation of the phytochrome protein itself. These changes can influence the interaction of phytochromes with other proteins and signaling molecules, thereby initiating a cascade of biochemical events that result in changes in gene expression and plant behavior.
- Regulation of Biological Processes: The chromophore’s ability to switch between different forms allows phytochromes to regulate a wide range of biological processes in response to light. For example, the P_fr form is typically the active form that promotes flowering and other developmental processes, while the P_r form is often associated with a state of inactivity. This dynamic regulation is crucial for plants to adapt to their environment.
- Integration of Environmental Signals: The chromophore enables phytochromes to integrate various environmental signals, such as light quality, quantity, and duration. This integration is vital for processes like photoperiodism, where plants use the information about day length to time their flowering and other developmental transitions accurately.
- Evolutionary Adaptation: The presence of different types of chromophores in various phytochrome families (e.g., bacterial, fungal, and plant phytochromes) reflects evolutionary adaptations to different light environments. This diversity allows organisms to optimize their light-sensing capabilities according to their ecological niches.
How can understanding phytochromes contribute to agricultural practices?
Understanding phytochromes can significantly contribute to agricultural practices in several ways:
- Enhanced Crop Yield: By manipulating phytochrome signaling pathways, agricultural scientists can develop crops that are more responsive to light conditions. This can lead to improved growth rates, better resource use efficiency, and ultimately higher crop yields. For instance, understanding how phytochromes regulate flowering can help in breeding or engineering plants that flower at optimal times for maximum yield.
- Improved Shade Tolerance: Phytochromes play a crucial role in how plants respond to shade. By understanding the mechanisms of shade avoidance and the role of phytochromes in this process, farmers can select or breed for varieties that are more tolerant to shading from neighboring plants. This can be particularly beneficial in densely planted crops or intercropping systems, where competition for light is intense.
- Optimized Planting Strategies: Knowledge of phytochrome function can inform planting strategies that take advantage of light conditions. For example, understanding the light requirements for germination and early growth can help farmers time their planting to coincide with optimal light availability, leading to better establishment and growth of crops.
- Manipulation of Growth Cycles: By understanding the photoperiodic responses mediated by phytochromes, farmers can manipulate the timing of flowering and fruiting in crops. This can be particularly useful in regions with variable climates, allowing for the adjustment of planting times to maximize harvests and reduce the risk of crop failure due to adverse weather conditions.
- Development of Novel Crop Varieties: Advances in genetic engineering and biotechnology allow for the development of crop varieties with modified phytochrome responses. For example, crops can be engineered to have altered sensitivity to light, enabling them to thrive in low-light conditions or to respond more effectively to specific wavelengths of light, which can enhance photosynthesis and growth.
- Sustainable Practices: Understanding how phytochromes influence plant responses to environmental stresses can lead to more sustainable agricultural practices. For instance, crops that are better able to cope with drought or nutrient deficiencies due to enhanced light sensing can reduce the need for chemical fertilizers and irrigation, promoting more sustainable farming practices.
- Precision Agriculture: The integration of phytochrome knowledge with precision agriculture technologies can lead to more targeted and efficient use of resources. For example, sensors that monitor light conditions can be used to adjust irrigation, fertilization, and pest control measures based on the specific needs of crops at different growth stages.
What potential biotechnological applications arise from phytochrome research?
Phytochrome research has opened up several potential biotechnological applications that can significantly impact agriculture, horticulture, and even biotechnology industries. Here are some key applications:
- Crop Improvement and Genetic Engineering: Understanding the molecular mechanisms of phytochrome signaling can facilitate the development of genetically modified crops with enhanced traits. For example, crops can be engineered to have altered phytochrome sensitivity, allowing them to optimize growth and yield under varying light conditions, such as low light or shade.
- Manipulation of Flowering Time: By targeting phytochrome pathways, scientists can develop crops that flower at specific times, which is crucial for maximizing yield and ensuring that crops are harvested at the right moment. This can be particularly beneficial in regions with variable climates or for crops that require specific photoperiods to flower.
- Enhanced Stress Tolerance: Phytochromes play a role in how plants respond to environmental stresses such as drought, salinity, and extreme temperatures. By understanding these pathways, researchers can develop crops that are more resilient to such stresses, potentially reducing the need for chemical inputs and improving food security.
- Light Management in Controlled Environments: In greenhouse and indoor farming, manipulating light conditions is crucial for optimizing plant growth. Phytochrome research can inform the design of lighting systems that provide the optimal light spectrum for different growth stages, enhancing photosynthesis and growth rates.
- Bioremediation: Phytochromes can be utilized in the development of plants that are better suited for bioremediation, the process of using plants to clean up contaminated environments. By engineering plants with enhanced light-sensing capabilities, it may be possible to improve their growth and efficiency in absorbing pollutants.
- Development of Novel Photoreceptors: The insights gained from phytochrome research can lead to the development of synthetic photoreceptors that can be used in various applications, including biosensors and bioengineering. These synthetic systems could be designed to respond to specific light conditions, enabling innovative solutions in environmental monitoring and biotechnology.
- Precision Agriculture: Phytochrome research can contribute to precision agriculture by informing the development of smart farming technologies that monitor light conditions and adjust agricultural practices accordingly. This can lead to more efficient use of resources, such as water and fertilizers, and improved crop management.
- Horticultural Applications: In ornamental horticulture, understanding phytochrome function can help in breeding and cultivating plants with desirable traits, such as improved flowering times, color, and growth habits. This can enhance the aesthetic value of ornamental plants and improve their marketability.
- Synthetic Biology: Phytochromes can be integrated into synthetic biology platforms to create novel biological systems that respond to light. This can lead to the development of new bio-based products, such as biofuels, pharmaceuticals, and other valuable compounds, by harnessing the natural light-sensing capabilities of phytochromes.
Phytochrome functional domains
Phytochromes are complex photoreceptor proteins crucial for regulating various plant processes in response to light. Understanding the functional domains of phytochrome proteins provides insight into how they manage these diverse roles. This section details the functional domains of phytochrome and their contributions to its activity.
- Overview of Phytochrome Structure
- Multigene Family: Phytochrome proteins are encoded by a multigene family, including members such as phyA through phyE. Each phytochrome has distinct expression patterns and functions, leading to specific regulatory roles in plant development.
- Domain Structure: Phytochromes are composed of two primary domains connected by a flexible hinge. These domains are crucial for the protein’s light-sensing and signal-transmitting functions.
- Functional Domains of Phytochrome
- N-terminal Domain:
- Chromophore Binding: The N-terminal domain contains the chromophore-binding site. This domain is responsible for the light-sensing capabilities of the phytochrome. It binds to the chromophore, phytochromobilin, which is essential for the protein’s photoactivity.
- Light Specificity and Stability: The N-terminal domain determines the specificity of light absorption, whether in the red or far-red spectrum. This domain also contributes to the stability of the protein in its photoactive form.
- PEST Sequence: This sequence within the N-terminal domain is involved in the regulation of protein degradation. The PEST sequence signals for the protein’s breakdown when it is no longer needed, thus regulating the protein levels within the cell.
- C-terminal Domain:
- Signal Transmission: The C-terminal domain is crucial for transmitting the light-induced signals from the N-terminal domain to downstream signaling proteins. This domain ensures that the light signal is effectively converted into a cellular response.
- Dimerization Site: Phytochromes often function as dimers. The C-terminal domain contains the site necessary for the formation of phytochrome dimers, which is essential for their full biological activity.
- Ubiquitination Site: This domain includes a site for ubiquitination, a process that tags the protein for degradation. Ubiquitination helps regulate the levels of phytochrome in the cell, thus controlling its activity.
- Regulatory Region: This area of the C-terminal domain is involved in modulating the activity of the phytochrome, influencing how it interacts with other proteins and signaling pathways.
- N-terminal Domain:
- Experimental Insights
- Overexpression Studies: Plants genetically engineered to overexpress specific phytochrome genes, such as PHYA or PHYB, often exhibit distinct phenotypes. Typically, these plants are dwarfed, dark green due to elevated chlorophyll, and show reduced apical dominance. These alterations indicate the necessity of balanced phytochrome levels for normal growth and development.
- Mutant Analysis: Plants expressing only the N-terminal domain or mutated forms of phytochrome often display normal phenotypes if the mutated forms cannot bind chromophore or if only the N-terminal domain is present. These studies suggest that both the N-terminal and C-terminal domains are necessary for full phytochrome functionality.
- Significance of Functional Domains
- Photosensory Specificity: The N-terminal domain is integral for determining the specificity of light response, influencing whether phytochrome responds to red or far-red light.
- Signal Transduction: The C-terminal domain plays a key role in signal transduction, facilitating the communication of light-induced changes from the phytochrome to the cell’s internal signaling pathways.
- Regulation and Degradation: The C-terminal domain’s involvement in dimerization and ubiquitination highlights its role in regulating phytochrome activity and ensuring proper turnover of the protein.
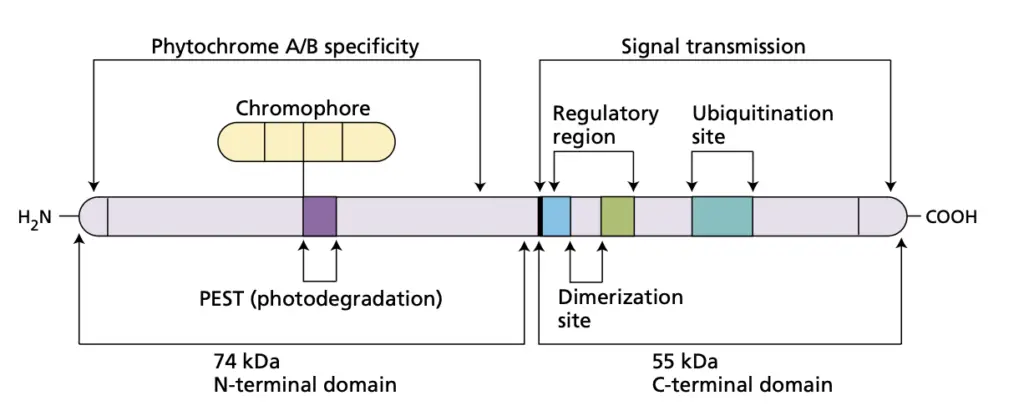
Functions of Phytochrome
Phytochrome serves several critical functions in plants, primarily related to their ability to sense and respond to light. Here are the key functions of phytochrome:
- Light Perception: Phytochrome is a primary photoreceptor that detects red and far-red light. It plays a crucial role in helping plants perceive their light environment, which is essential for their growth and development.
- Photomorphogenesis: Phytochrome regulates various developmental processes in response to light, a phenomenon known as photomorphogenesis. This includes:
- Seed Germination: Phytochrome promotes germination in seeds, particularly in species like lettuce, where red light stimulates the process.
- De-etiolation: In etiolated seedlings, exposure to red light leads to de-etiolation, which includes the unrolling of leaves and the development of chlorophyll.
- Shade Avoidance: Phytochrome helps plants detect the presence of neighboring plants through changes in light quality (e.g., the ratio of red to far-red light). When plants sense shade, they can initiate growth responses, such as stem elongation, to outcompete their neighbors for light.
- Regulation of Flowering: Phytochrome plays a significant role in the regulation of flowering time in response to photoperiod (the length of day and night). It helps plants determine the appropriate time to flower based on light conditions.
- Chloroplast Movement: In some algae, such as Mougeotia, phytochrome regulates the movement of chloroplasts in response to light direction, optimizing light capture for photosynthesis.
- Gene Expression: Phytochrome influences gene expression by regulating transcription factors and other signaling pathways. It can activate or repress the expression of specific genes in response to light, leading to various physiological changes.
- Rapid Responses: In addition to long-term developmental changes, phytochrome can induce rapid physiological responses, such as alterations in membrane potential and ion fluxes, which are crucial for immediate adaptations to changing light conditions.
- Shade Avoidance: Phytochromes enable plants to detect the ratio of red to far-red light, which changes when plants are shaded by others. In response to perceived shade, phytochromes trigger elongation growth to help the plant reach for more light, a phenomenon known as shade avoidance 2.
- Circadian Rhythms: Phytochromes play a role in regulating circadian rhythms, helping plants maintain a biological clock that aligns their physiological processes with the day-night cycle. This regulation ensures that plants optimize their growth and metabolic activities according to the availability of light.
- Flowering Regulation: Phytochromes are involved in the regulation of flowering time. They help determine when a plant should flower based on the light conditions, which is crucial for reproductive success. This process is often linked to the length of day (photoperiod.
- Seed Germination: Phytochromes influence seed germination by detecting light conditions. Many seeds require light to germinate, and phytochromes help initiate the germination process when the appropriate light signals are present.
- Response to Environmental Stress: Phytochromes can also mediate plant responses to various environmental stresses, such as drought or high temperatures, by regulating growth patterns and metabolic processes that enhance survival under adverse conditions.
- Interaction with Other Signaling Pathways: Phytochromes interact with other signaling pathways, including those involving other photoreceptors (like cryptochromes) and hormonal signals (such as auxins and gibberellins), to coordinate a comprehensive response to light and environmental changes.
Ecological Functions: Phytochrome Specialization
Phytochromes are a family of photoreceptor proteins crucial for plant adaptation to light conditions. Encoded by a multigene family including PHYA through PHYE, each phytochrome plays distinct roles in plant ecology and development. This section elucidates the ecological functions of different phytochromes, with a primary focus on phytochromes A (phyA) and B (phyB).
- Phytochrome B (phyB)
- Responses to Continuous Red or White Light:
- Mutant Studies: Phytochrome B’s role was initially identified through the study of the hy3 mutant (now phyB), which exhibited elongated hypocotyls under continuous white light due to a defective PHYB gene. These mutants showed reduced or absent PHYB mRNA and phyB protein, while levels of PHYA mRNA and phyA protein remained normal.
- Shade Avoidance: PhyB is integral in regulating shade avoidance by modulating hypocotyl elongation in response to red light. In low-fluence pulses or continuous red light, phyB influences hypocotyl length and is involved in the end-of-day far-red response. Mutants lacking phyB fail to extend their hypocotyls under these conditions, indicating its crucial role in shade avoidance and light perception.
- Chlorophyll and Hormone Response: The phyB mutant also displays deficiencies in chlorophyll and chloroplast protein mRNAs and shows impaired hormone responsiveness, suggesting that other phytochromes cannot compensate for the loss of phyB in continuous red or white light conditions.
- Photoreversible Seed Germination: PhyB mediates photoreversible seed germination, a phenomenon discovered with phytochrome research. Arabidopsis seeds, which require light for germination, exhibit red/far-red reversibility in the low-fluence range. Mutants deficient in phyB cannot respond to low-fluence red light, underscoring phyB’s role in this process.
- Responses to Continuous Red or White Light:
- Phytochrome A (phyA)
- Responses to Continuous Far-Red Light:
- Mutant Identification: The role of phyA was clarified through mutants that showed tall, spindly growth under continuous far-red light, a condition requiring light-labile phytochrome. PhyA mutants exhibited normal growth under continuous red light but were unresponsive to continuous far-red light, distinguishing their role in detecting far-red light from other phytochromes.
- Function in Photomorphogenesis: PhyA is essential for responses to continuous far-red light and is particularly important during seed germination under canopy conditions, where far-red light predominates. It contributes to de-etiolation and far-red light responses, though its role in white light sensing is minimal.
- Very-Low-Fluence Response: PhyA is involved in the very-low-fluence response (VLFR) during seed germination. Mutants lacking phyA cannot germinate in response to red light at very low fluences but respond normally to higher fluences, highlighting phyA’s primary role in VLFR. However, recent studies also implicate phyE in this process.
- Responses to Continuous Far-Red Light:
- Phytochromes C, D, and E
- Emerging Roles:
- Functional Overlap: Phytochromes C, D, and E have overlapping functions with phyA and phyB. Research involving double and triple mutants has shown that phyD, like phyB, is involved in regulating leaf petiole elongation and flowering time. PhyE also acts redundantly with phyB and phyD in these processes and with phyA and phyB in inhibiting internode elongation.
- Characterization and Redundancy: Phytochrome C remains the least characterized, but studies indicate that its functions overlap with those of other phytochromes. The creation of quadruple mutants has revealed that phyC, phyD, and phyE have roles that are largely redundant with phyA and phyB, with phyB being involved in all stages of development, whereas the others are more specialized.
- Emerging Roles:
- Interactions in Early Germination
- Mutually Antagonistic Roles: The interactions between phyA and phyB are critical during early germination. Continuous red light stimulates de-etiolation through phyB by maintaining high levels of PfrB, while continuous far-red light inhibits this response by reducing PfrB levels. The photostationary state of phytochrome determines the effectiveness of phyA in de-etiolation, particularly under far-red light conditions.
- Shade versus Sunlight: In full sunlight, de-etiolation is mediated primarily by phyB. However, under canopy shade enriched in far-red light, phyA initiates de-etiolation. As phyA is labile, the response shifts to phyB in the presence of red light, facilitating accelerated stem elongation associated with the shade avoidance response.
- Rockwell NC, Su YS, Lagarias JC. Phytochrome structure and signaling mechanisms. Annual Review of Plant Biology. 2006 ;57:837-858. DOI: 10.1146/annurev.arplant.56.032604.144208. PMID: 16669784; PMCID: PMC2664748.
- Memon, Abdul & Duraković, Ćamil. (2015). Signal Perception and Transduction in Plants. Periodicals of Engineering and Natural Scineces. 2. 15-29. 10.21533/pen.v2i2.42.
- https://europepmc.org/backend/ptpmcrender.fcgi?accid=PMC2664748&blobtype=pdf
- https://www.brainkart.com/article/Phytochrome,-photoperiodism-and-photomorphogenesis_14124/