Nutrient transport in plants is a crucial process that ensures the distribution of essential minerals and organic compounds necessary for growth, development, and metabolic functions. This transport occurs through various tissues, primarily the xylem and phloem, each serving distinct roles in the movement of nutrients. The following points outline the key mechanisms and pathways involved in nutrient transport in plants.
- Xylem Transport:
- Function: The xylem is primarily responsible for the upward movement of water and dissolved mineral nutrients from the roots to the shoots and leaves.
- Mechanism:
- The transport in xylem is driven by root pressure and transpiration pull, creating a gradient of hydrostatic pressure and water potential.
- Water evaporates from the stomata in leaves, creating negative pressure that pulls water and minerals upward through the xylem vessels.
- Loading and Unloading:
- Nutrients enter the xylem through root hairs, where they move via apoplastic and symplastic pathways.
- Once in the xylem, nutrients are transported against concentration gradients through active transport mechanisms.
- Phloem Transport:
- Function: The phloem is responsible for the bidirectional transport of organic compounds, primarily sucrose, and other nutrients throughout the plant.
- Mechanism:
- Nutrients are loaded into the phloem from source tissues (e.g., leaves) and are then transported to sink tissues (e.g., roots, fruits, or developing leaves) as needed.
- The process relies on turgor pressure differences between source and sink regions, with nutrients moving from areas of higher concentration to lower concentration.
- Loading and Unloading:
- Loading typically involves active transport of sucrose into the phloem, which increases osmotic pressure and facilitates water influx from the xylem, enhancing nutrient transport.
- Unloading occurs in sink tissues, where sucrose is either utilized or converted to other forms for storage.
- Nutrient Uptake in Roots:
- Root Hairs: The primary site for nutrient absorption, root hairs increase surface area, facilitating the uptake of water and nutrients from the soil.
- Active Transport: Many essential nutrients, such as potassium (K+), calcium (Ca2+), and nitrate (NO3-), are taken up against concentration gradients, requiring energy from ATP.
- Symplastic and Apoplastic Pathways: Nutrients can move through the symplast (via plasmodesmata between cells) or the apoplast (through cell walls and intercellular spaces) until they reach the endodermis, where the Casparian strip forces them into the symplast for further transport.
- Nutrient Redistribution:
- Nutrient transport is not solely upward; plants can also redistribute nutrients based on developmental needs or environmental conditions.
- For example, during periods of stress or dormancy, nutrients can be mobilized from older tissues to support new growth.
- Influence of Environmental Factors:
- Nutrient transport can be influenced by various environmental factors, including soil moisture, temperature, and light conditions.
- Mycorrhizal associations with fungi can enhance nutrient uptake by extending the root system and increasing the surface area available for absorption.
- Nutrient Deficiency Symptoms:
- Deficiencies in essential nutrients can lead to specific symptoms, such as chlorosis (yellowing of leaves), stunted growth, and poor fruit development, indicating the need for adequate nutrient transport and availability.
Nutrient absorption
Nutrients and the Soil
Soil serves as the critical medium for the storage and exchange of essential mineral nutrients necessary for plant growth. Understanding the dynamics of nutrient uptake by plants involves delving into the ionic forms of minerals, soil properties, and the complex interactions between soil components and plant roots.
- Mineral Uptake Basics:
- Early research conducted by Hoagland, Stout, and Arnon in 1923 established that plants absorb minerals primarily in ionic form.
- The uptake rate of various ions by plant roots is not uniform; one ion can influence the absorption of others, demonstrating the interdependence of nutrients in the soil.
- Soil as a Medium:
- Soil acts as a reservoir for mineral ions, and several factors—including its properties, ion exchange capacity, pH, and the types of cations and anions present—affect the availability of these ions for plant uptake.
- Simply having an abundance of a specific mineral in the soil does not guarantee that it is accessible to plants. Ions can adhere to clay particles or precipitate as insoluble salts, reducing their availability.
- Soil Composition and Water Holding Capacity:
- Soils with high water retention typically exhibit higher mineral holding capacity due to the presence of fine particles like clay and humus.
- Clay and humus have a large surface-to-volume ratio and are predominantly negatively charged. Their high specific surface area (up to 10,000-fold larger than coarse particles) allows for significant interaction with mineral elements in the soil solution.
- Colloidal Properties:
- Colloidal clay particles, such as micelles, have numerous negative surface charges. Cations in the soil are loosely bound to these negative charges via ionic bonds.
- Cations can exchange rapidly and reversibly with those in the soil solution. Notably, H⁺ ions exhibit a greater affinity for charged soil particles than do cations like Ca²⁺, Mg²⁺, or K⁺, which facilitates the release of these nutrients into the soil water for plant uptake.
- Influence of Soil Acidity:
- The acidity of soil can increase due to the respiration process, where CO₂ released interacts with soil water to form carbonic acid. This acidification results in a higher concentration of H⁺ ions, which can displace other cations due to their strong attraction to the colloidal surface charges on clay micelles.
- A common colloidal clay found in soils is kaolinite (Al₂Si₂O₅(OH)₄). The ionization of alumina and silica at the edges of clay particles generates a large number of negative charges. Additionally, colloidal carbon derived from decomposed organic matter also contributes negative charges to the soil.
- Ion Exchange Capacity and pH:
- The ion exchange capacity, which determines how well soil can hold and exchange cations, is heavily influenced by soil pH. Changes in pH affect the availability of different ions to plants, highlighting the importance of maintaining optimal pH levels for nutrient uptake.
Uptake of Mineral Ions
The uptake of mineral ions by plants is a complex process influenced by several factors, including ion concentration, plant species, and soil properties. Through various experiments, particularly those examining ion concentrations in the root cells, insights have emerged about how plants selectively absorb essential nutrients from their environment.
- Experimental Insights:
- In a controlled experiment, two plant species, maize and beans, were analyzed for changes in ion concentration over four days in a nutrient solution. The results displayed varying concentrations of key ions in both the external nutrient solution and the root sap, highlighting differences in nutrient uptake capabilities.
- Ion Concentrations:
- Observations indicated that certain ions, such as potassium, phosphate, and nitrate, showed a marked decline in concentration in the bathing medium after four days.
- Conversely, sodium and sulphate concentrations increased, suggesting that water was absorbed more rapidly than these ions or that leaching occurred.
- Ion Uptake Characteristics:
- Selectivity: Plants exhibit selective absorption, preferentially taking up specific mineral elements while discriminating against others. For instance, potassium (K⁺) absorption is not significantly influenced by sodium (Na⁺) presence, demonstrating selectivity even among chemically similar ions.
- Accumulation: Mineral elements can accumulate in plant sap at concentrations significantly higher than those found in the external solution. This indicates that the uptake process operates against a concentration gradient.
- Genotype: Different plant species show varying capacities for nutrient uptake, attributed to their unique genetic makeups.
- Ion Exchange Mechanisms:
- The binding affinity of cations follows a specific order, known as the lyotropic series, where Al³⁺ has the highest affinity, followed by H⁺, Ca²⁺, Mg²⁺, K⁺, NH₄⁺, and Na⁺. The capacity for cation adsorption is reversible, meaning that ions with a lower binding affinity, such as Ca²⁺, can be replaced by ions with higher affinity, such as H⁺.
- In this context, sodium ions exchange readily while aluminum ions exhibit minimal exchangeability. The colloidal fraction of soil, which holds these adsorbed ions, acts as the main nutrient reservoir, continuously replenished by the weathering of rock particles that release ions into the soil solution.
- Specificity of Ion Uptake:
- While some ions are selectively absorbed, competitive inhibition can occur. For example, K⁺ absorption may be inhibited by the presence of rubidium (Rb⁺), showcasing that the uptake mechanisms can be influenced by similar ions.
- Additionally, the presence of calcium (Ca²⁺) is essential for the selectivity of K⁺ absorption, as a deficiency of Ca²⁺ can lead to increased inhibition by Na⁺.
- Influencing Factors:
- The rate and selectivity of nutrient uptake are affected by several factors, including temperature, oxygen availability, toxic substances, carbohydrate content in tissues, and light conditions. These influences can parallel enzyme-mediated reactions, indicating the involvement of specific proteins in the solute uptake processes.
Movement of Nutrients in the Root
The movement of nutrients within plant roots is a vital process for plant health and development. This movement primarily occurs through two main pathways: the apoplast and symplast. Understanding how these pathways function and how nutrients are transported to the xylem provides critical insights into nutrient dynamics in plants.
- Transport Pathways:
- The apoplast route involves the movement of water and dissolved solutes through the cell wall spaces and intercellular spaces, which are virtually continuous with the external soil solution. This pathway allows for passive movement without crossing cell membranes.
- In contrast, the symplast route requires the movement of solutes through the cytoplasm of cells, facilitated by plasmodesmata—small channels that connect adjacent plant cells. When an ion is absorbed by an epidermal cell, it can move toward the xylem either by crossing several layers of cells through their plasma membranes or via the plasmodesmata without needing to cross plasma membranes.
- Cellular Barriers:
- The endodermis of the root presents a significant barrier to nutrient movement through the apoplastic pathway. This layer of cells is lined with Casparian strips, which are bands of cell wall material that are impregnated with suberin—a waxy substance that is impermeable to water and solutes.
- Consequently, once nutrients reach the endodermis, they cannot continue through the apoplast and must instead enter the symplast. This requirement ensures that all minerals must pass through the cytoplasm of the endodermal cells to reach the xylem, facilitating selective uptake and preventing harmful substances from entering the vascular system.
- Tracing Nutrient Movement:
- To illustrate the path of a nutrient entering the root from the surrounding soil solution, consider the journey of a solute. Upon absorption by root hair cells, the nutrient travels through the epidermis and several layers of cortical cells. Then, it must cross the endodermis and enter the pericycle before reaching the xylem vessels.
- This movement involves crossing various structures, including the primary cell walls, middle lamella, and plasma membranes of adjacent cells.
- Diffusion Dynamics:
- If a cell that has absorbed a high concentration of ions is placed in a lower-salt solution, some of the absorbed ions will naturally diffuse back into the external medium. This process follows the principles of simple diffusion, demonstrating the movement of solutes based on concentration gradients.
- The part of the cell wall involved in this free movement of ions is referred to as the Outer Space, highlighting the importance of the physical environment in nutrient dynamics.
Free Space
Free space within plant roots plays a crucial role in the initial stages of mineral ion uptake. It refers to the regions within plant tissue that facilitate the diffusion of ions and nutrients before they reach the cellular interior. This understanding is essential for educators and students exploring plant physiology and nutrient dynamics.
- Definition and Composition:
- Free space, specifically termed Apparent Free Space (AFS), denotes the portion of root volume that is readily accessible for the diffusion of solutes dissolved in water.
- It is primarily constituted by intercellular spaces, the amorphous matrix of cell walls, and the small pores present in cellulose microfibrils.
- Cell Wall Structure:
- The primary cell wall of plant cells is composed mainly of cellulose microfibrils embedded in a matrix made of hemicelluloses and pectic substances.
- Hemicelluloses comprise various sugars other than glucose, such as xyloglucans, while pectic substances are derived from polygalacturonic acids, which possess weak carboxylic acid groups (-COOH) that ionize and generate negative charges.
- These negatively charged areas in the cell wall are referred to as cation adsorption sites, also known as cation exchange sites or Donnan Free Space.
- Cation Adsorption:
- When positively charged ions, such as K+^++, Mg2+^{2+}2+, and Ca2+^{2+}2+, diffuse through the cell wall, they can displace the loosely held hydrogen ions associated with the carboxyl groups.
- The cations are held in place through weak inter-ionic attractive forces, indicating that ions with a higher adsorptive capacity (like Ca2+^{2+}2+) can displace those with lower affinity (such as K+^++).
- Pore Characteristics:
- The cellulose microfibrils in the cell wall are not tightly packed, allowing the formation of small pores that facilitate the free movement of water and dissolved substances.
- The dimensions of these pores typically exceed 5.0 nm, while hydrated ions like K+^++ (0.66 nm) and Ca2+^{2+}2+ (0.82 nm) are small enough to diffuse readily through them.
- Diffusion Dynamics:
- Water, dissolved nutrients, and metabolites, including glucose, sucrose, and amino acids, can diffuse efficiently across the primary cell walls due to the accessible nature of the free space.
- This area is bounded by the plasma membrane of the epidermal and cortical cells and the Casparian strip in the endodermis, effectively forming a barrier to further inward movement of substances.
- Role of the Plasma Membrane:
- Upon reaching the protoplasm’s outer surface, substances must pass through the plasma membrane, which serves as a selective barrier.
- While it inhibits certain ions and molecules from entering the cell, it can allow the passage of others, indicating its dynamic and selective properties.
- Estimation of Free Space:
- The AFS, which comprises about 10-25 percent of the root volume, includes cell walls and intercellular spaces, functioning similarly to the apoplast of the root’s epidermal and cortical cells.
- This space is characterized by its negative charge and the capacity to hold cations via electrostatic attraction, accommodating ions through free diffusion without requiring membrane crossing.
Transport of ions across plasma Membrane
The transport of ions across the plasma membrane is a fundamental process essential for cellular functions in organisms, including roundworms. This process ensures that necessary nutrients are absorbed, while waste products are expelled, facilitating homeostasis and metabolic activity. Various mechanisms govern this transport, depending on the nature of the ions, their concentration gradients, and the energy requirements.
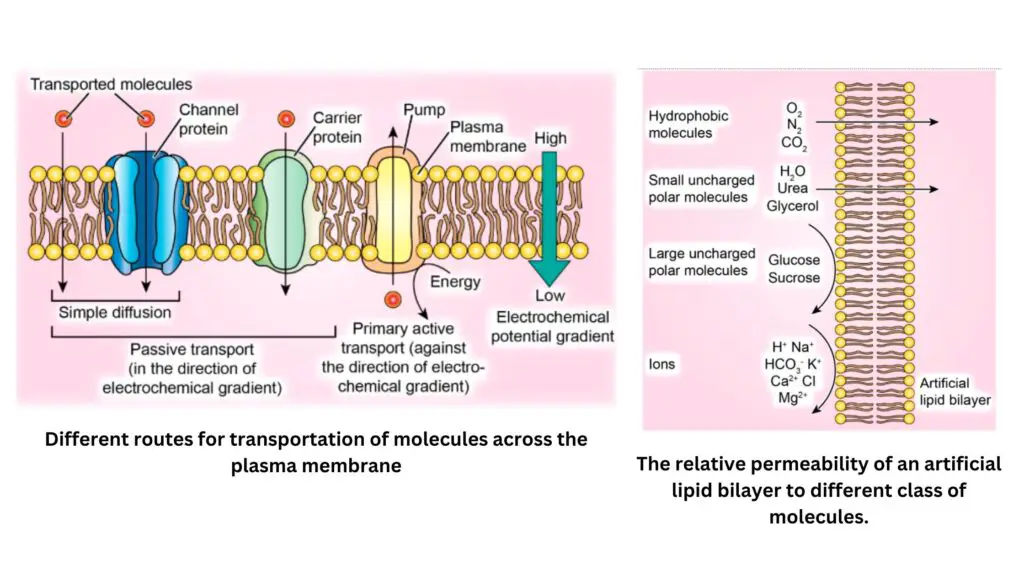
- Mechanisms of Ion Transport: The transport of ions across the plasma membrane can be categorized into three primary modes: simple diffusion, facilitated diffusion, and active transport. Each of these mechanisms has distinct characteristics and is influenced by specific physical and chemical factors.
- Simple Diffusion:
- This process allows non-polar solutes to passively cross the lipid bilayer of the membrane. Molecules diffuse down their concentration gradient, driven by their kinetic energy.
- The rate of diffusion is influenced by the size of the molecule and its solubility in lipids. For example, small non-polar molecules like oxygen (O₂) and nitrogen (N₂) diffuse rapidly, while larger molecules like glucose (180 Da) encounter resistance. Interestingly, polar molecules, despite their size, can also diffuse rapidly through specialized channels known as aquaporins, which facilitate the movement of water molecules (18 Da).
- Charged ions such as Na⁺ and K⁺ have a much lower permeability through lipid bilayers compared to water, as their charge and associated hydration shells hinder entry into the hydrophobic core of the membrane.
- Facilitated Diffusion:
- Transport proteins embedded in the membrane assist in the passage of ions and polar molecules that cannot diffuse freely through the lipid bilayer.
- These proteins can be categorized into three types: channels, carriers, and pumps.
- Channels: Channel proteins form water-filled pores that allow specific ions to pass through based on size and charge. Some channels are gated, opening and closing in response to signals such as changes in membrane potential or binding of ligands. For instance, K⁺ channels are crucial for the function of guard cells in stomatal opening.
- Carriers: Carrier proteins exhibit high specificity for particular ions or organic molecules. The binding of the solute induces conformational changes in the carrier, facilitating transport across the membrane. The rate of transport via carriers is slower than through channels, typically allowing for the movement of 100-1000 molecules per second compared to millions through channels.
- Simple Diffusion:
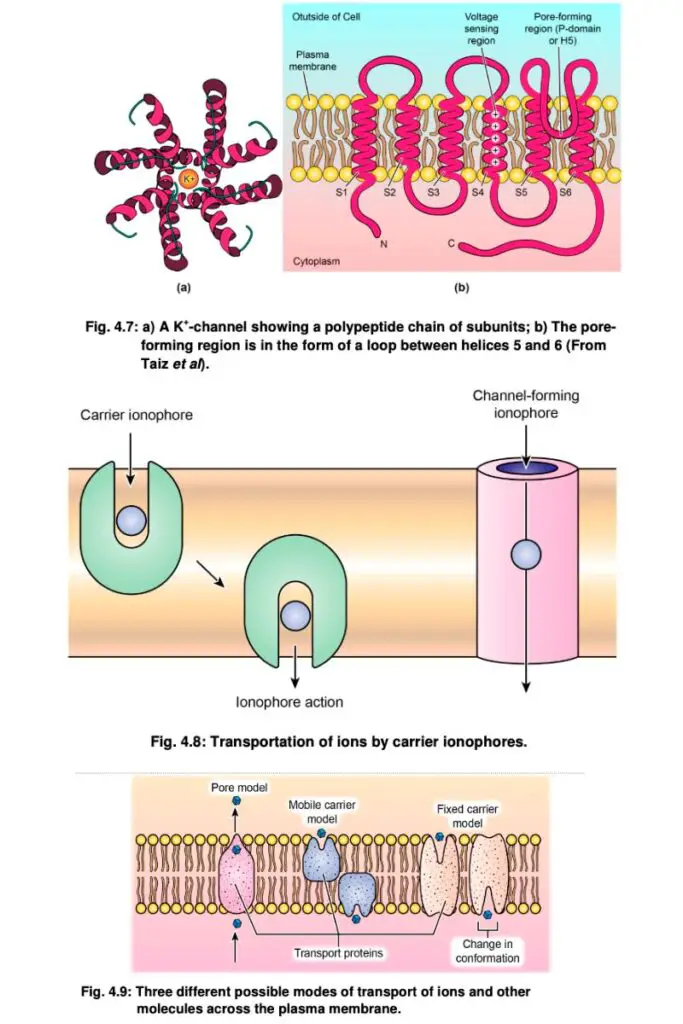
Simple Diffusion
Simple diffusion is a fundamental process by which ions and molecules traverse cell membranes, specifically the lipid bilayer. This process is crucial for various biological functions in organisms, including plants. The following points provide an overview of the mechanisms, characteristics, and implications of simple diffusion.
- Definition and Overview: Simple diffusion refers to the passive transport of non-polar solute molecules across cell membranes. This movement occurs down a concentration gradient, meaning molecules move from areas of higher concentration to areas of lower concentration, relying on their inherent kinetic energy.
- Lipid Bilayer Structure: An artificial or synthetic lipid membrane allows for differential permeability. Non-polar solute molecules are able to pass through the membrane more easily than polar molecules. The lipid bilayer consists of phospholipids arranged in a double layer, creating a hydrophobic interior that serves as a barrier to most polar substances.
- Factors Influencing Diffusion Rate:
- Molecular Size: The size of a molecule significantly impacts its ability to diffuse across the lipid bilayer. Smaller molecules tend to diffuse more readily.
- Lipid Solubility: Molecules that are hydrophobic or non-polar, such as oxygen (O2) and nitrogen (N2), dissolve in the lipid bilayer and diffuse rapidly. Conversely, larger or polar molecules, like glucose (180 Da), face greater challenges in penetrating the membrane.
- Examples of Diffusing Molecules:
- Small Non-Polar Molecules: O2 (32 Da) and N2 (28 Da) diffuse rapidly due to their low molecular weight and hydrophobic nature.
- Other Small Molecules: Ethanol (46 Da), carbon dioxide (CO2, 44 Da), and urea (60 Da) also cross the bilayer efficiently.
- Water Molecules: Despite being polar and less soluble in lipids, water (18 Da) diffuses quickly through specialized channels known as aquaporins, highlighting its unique transport mechanism.
- Ionic Molecules: Charged ions, such as sodium (Na+) and potassium (K+), encounter considerable resistance in the lipid bilayer, primarily due to their charge and the large hydration shell surrounding them. The permeability of the bilayer is approximately 10^9 times higher for water than for small ions. Potassium ions (K+) exhibit greater permeability compared to other ions, serving as a reference point for evaluating ion permeability.
- Statistical Pores: The lipid bilayer also contains transient pores, termed “statistical pores,” formed by the thermal movement of acyl phospholipid chains. These pores allow for the selective passage of smaller hydrophilic substances while restricting larger ones.
- Mechanism of Transport: Simple diffusion is characterized as a passive transport mechanism, which means it does not require ATP or any direct energy input. The driving force for diffusion arises from the concentration or electrochemical gradient of the solute. This principle is encapsulated in Fick’s Law, which asserts that the rate of diffusion is directly proportional to the concentration difference between two regions.
- Implications for Solute Transport: Since diffusion operates along a concentration gradient, it does not permit solutes to accumulate against this gradient. This characteristic is vital for maintaining cellular homeostasis, allowing cells to regulate internal concentrations of various substances effectively.
MembraneTransport Proteins
Membrane transport proteins play a critical role in facilitating the movement of various substances across biological membranes, which are inherently selective barriers. Unlike artificial membranes, biological membranes contain specialized proteins that enable the rapid passage of ions, sugars, amino acids, nucleotides, and other metabolites, which cannot traverse lipid bilayers on their own. The following points outline the structure, function, and importance of these transport proteins.
- Definition and Importance: Membrane transport proteins are integral components of biological membranes that mediate the transport of ions and other solutes across the membrane. They allow for the selective passage of substances that would otherwise be restricted by the lipid bilayer’s hydrophobic nature. This mechanism is crucial for maintaining cellular homeostasis and enabling various physiological processes.
- Facilitated Diffusion: Transport proteins facilitate a process known as facilitated diffusion, which overcomes the solubility challenges presented by lipid bilayers. This process is characterized by passive-mediated transport, as it occurs along concentration gradients without the expenditure of ATP. Therefore, the direction of solute movement is determined by either concentration gradients for uncharged solutes or electrochemical gradients for charged solutes.
- Classes of Membrane Transport Proteins: There are three primary classes of membrane transport proteins:
- Channels: These are transmembrane proteins that form water-filled pores, allowing specific ions or molecules to pass through. The selectivity of channel proteins is determined by the size of the pore and the nature of the charged surfaces lining the interior. Channels can be classified into two types:
- Simple Channels: Allow passive transport of water and ions without any gating mechanisms.
- Gated Channels: These channels open and close in response to external signals, such as changes in membrane potential or the presence of signaling molecules. Gated channels can be electrically gated or modulated by hormones and other stimuli.
- Channels: These are transmembrane proteins that form water-filled pores, allowing specific ions or molecules to pass through. The selectivity of channel proteins is determined by the size of the pore and the nature of the charged surfaces lining the interior. Channels can be classified into two types:
- Transport Specificity: Channels are highly selective based on the hydrated size of the ion species they permit. For instance, potassium (K+), chloride (Cl-), and calcium (Ca2+) channels are well characterized, with K+ channels playing a crucial role in processes like stomatal regulation in plants. The permeability of membranes can vary over time depending on the activity of these ion channels.
- Carriers (Transporters): These proteins do not form continuous pores across the membrane but bind specific ions or molecules, undergoing conformational changes to transport them. This process resembles an enzyme-substrate interaction, allowing for a high degree of specificity. Unlike channel-mediated transport, carrier-mediated transport is generally slower, with transport rates ranging from hundreds to thousands of molecules per second, compared to millions via channels.
- Ionophores: Ionophores are organic molecules that enhance the permeability of membranes to specific ions. They facilitate the diffusion of these ions across membranes by forming complexes with them, effectively shielding their charge from the hydrophobic environment. Ionophores are particularly important in transporting ions at rates significantly higher than those achievable by passive diffusion alone.
- Active Transport: Some transport proteins, known as pumps, can actively transport solutes against their concentration gradients using energy derived from ATP. This process is crucial for maintaining ion gradients essential for cellular functions.
- Driving Forces of Protein-Mediated Transport: The driving forces behind protein-mediated transport include concentration gradients for uncharged solutes and both concentration and electrical gradients for charged solutes. The Nernst equation provides a quantitative framework for understanding the relationship between ion concentration gradients and membrane potential. For example, a Nernst potential of 59 mV corresponds to a ten-fold concentration gradient for a univalent cation, indicating the voltage required to balance this concentration difference.
- Transmembrane Potential: All plasma membranes exhibit transmembrane potentials, with the inside of the cell generally more negative than the outside. This potential is generated by the active transport of ions, particularly H+ ions, out of the cell. It facilitates the entry of positively charged ions while opposing the influx of negatively charged ions, further emphasizing the role of transport proteins in regulating ion homeostasis.
Pumps and Active Transport
Pumps and active transport are crucial mechanisms that enable cells to regulate their internal environments by moving ions and metabolites against concentration gradients. This active transport process is fundamental to maintaining homeostasis, as it allows for the accumulation of necessary substances within the cell, even when external concentrations are unfavorable. Active transport occurs through the mediation of specific proteins known as pumps, which facilitate the movement of solutes using energy, primarily derived from ATP hydrolysis.
- Active Transport Definition: Active transport is defined as the unidirectional movement of ions and metabolites into or out of a cell against established concentration gradients. This process does not occur spontaneously; it requires energy input, typically from ATP. The Nernst equation, which relates to the electrochemical potential of ions, helps in understanding this dynamic.
- Types of Active Transport:
- Primary Active Transport: This form of transport directly uses energy derived from ATP hydrolysis or other metabolic processes to drive the movement of ions. The proteins involved in this mechanism are referred to as pumps. Examples include pumps that transport ions such as H⁺ and Ca²⁺, as well as larger organic molecules.
- Secondary Active Transport: In this mechanism, energy is not directly derived from ATP but instead from ion gradients created by primary active transport processes. The proton motive force (PMF), generated primarily by H⁺ transport, serves as the driving force for this type of transport.
- Role of Pumps: Pumps play a central role in active transport. They facilitate the movement of specific ions against their concentration gradients. The most notable pumps include:
- H⁺-ATPase: This proton pump generates a gradient of electrochemical potential across the plasma membrane, essential for many cellular functions, including ATP synthesis in mitochondria and chloroplasts.
- Ca²⁺ Pumps: These actively transport calcium ions out of cells, thereby maintaining low intracellular calcium concentrations, which is critical for various cellular processes.
- Mechanism of Active Transport: Active transport can occur in two directions:
- Into the Cell: For ions that are at lower concentrations outside the cell, such as K⁺, active transport can increase their intracellular concentration. For instance, when external K⁺ concentrations are low, active uptake mechanisms facilitate their entry.
- Out of the Cell: Conversely, ions like Na⁺ may need to be actively expelled to maintain optimal internal concentrations, highlighting the need for precise regulation of ion concentrations within cells.
- Importance of Ion Gradients: The differences in ion concentrations across membranes not only affect transport dynamics but also impact the overall electrical potential of the cell. The Nernst equation helps to determine the expected concentrations based on membrane potential, while the Goldman equation accounts for the contributions of multiple ions to membrane potential.
- Transport Processes:
- Symport: This process involves the simultaneous transport of H⁺ ions and another solute in the same direction across the membrane. For example, sugars can be symported into the cell along with H⁺ ions, utilizing the energy derived from the proton gradient.
- Antiport: In contrast, antiport systems couple the transport of H⁺ ions with another solute moving in the opposite direction. This mechanism allows for the expulsion of ions like Na⁺ while bringing in H⁺ ions or other metabolites.
- Functional Characteristics of Pumps: The H⁺-ATPase is a prominent example, typically featuring ten transmembrane segments that help establish the proton gradient. This pump’s activity can be influenced by the presence of certain ions, such as K⁺, which can alter the pump’s conformation during ATP hydrolysis.
- Regulation of Transport: Transport proteins in cell membranes, including symporters and antiporters, play significant roles in regulating ion concentrations and the uptake of metabolites. Their function can resemble enzyme-substrate interactions, where binding sites become saturated at high substrate concentrations, limiting further transport despite increased external ion availability. Competitive inhibitors can also influence the binding of ions to these carrier proteins.
Radial movement of ions in roots
The radial movement of ions in roots is a crucial process that allows plants to transport essential minerals from the soil into the xylem, facilitating their subsequent distribution throughout the plant. This movement is intricately tied to the anatomical features of the roots, enabling effective ion absorption and transport. The following points elucidate the mechanisms and pathways involved in this process.
- Entry of Mineral Ions:
- Mineral ions first diffuse into the cell wall of root hairs, entering through the apparent free spaces. This initial phase allows for the passive movement of nutrients from the soil.
- The ions then follow two main pathways: the apoplastic pathway and the symplastic pathway.
- Apoplastic Pathway:
- In this route, nutrients move along the cell wall and intercellular spaces of the epidermal and cortical cells.
- The apoplastic pathway facilitates the rapid transport of ions without crossing cellular membranes until reaching the endodermis.
- Symplastic Pathway:
- Some ions are absorbed by epidermal and cortical cells, entering the cytoplasm through plasmodesmata. This pathway allows ions to move cell-to-cell through the cytoplasmic connections, ensuring efficient transport.
- The presence of plasmodesmata suggests active transport mechanisms within cells, although quantifying their role has been challenging.
- Role of Endodermis and Casparian Strips:
- The endodermis acts as a regulatory checkpoint in the radial movement of ions. The casparian strips present in the endodermal cell walls prevent further entry of water and minerals via the apoplastic route.
- This selective barrier necessitates that all ions enter the symplast at the level of the endodermis before they can proceed to the stele, ensuring that only specific ions are absorbed.
- Ion Transport to the Xylem:
- Once ions reach the stele, they are released into the xylem vessels. Contrary to earlier beliefs that this transfer occurred passively, recent studies indicate that it is an energy-dependent process.
- Xylem parenchyma cells play a critical role in actively transporting ions into the xylem vessels against a concentration gradient.
- Energy Requirements:
- The transfer of ions into the xylem is sensitive to uncouplers of ATP formation, such as carbonyl cyanide m-chlorophenylhydrazone (CCCP), as well as inhibitors of protein synthesis like cycloheximide. This observation supports the notion that metabolic energy is required for ion flux from xylem parenchyma to the xylem tracheary elements.
- Carrier proteins, ion channels, and plasma membrane H⁺-ATPases facilitate this process, akin to mechanisms used for loading nutrients into root cells.
- Pathway Preferences:
- The preferential use of apoplastic versus symplastic pathways by different ions remains to be fully understood.
- Experimental studies with radioisotopes, such as 86Rb^{86}Rb86Rb and 36Cl^{36}Cl36Cl, have shown that certain ions preferentially utilize the symplastic pathway. In contrast, 45Ca2+^{45}Ca^{2+}45Ca2+ in barley seedlings is predominantly transported via the apoplastic pathway, indicating that ion transport mechanisms are ion-specific.
- Implications for Plant Physiology:
- The successful radial movement of ions from root hairs to the xylem is essential for maintaining plant health and growth.
- These processes not only facilitate nutrient uptake but also contribute to turgor pressure and overall root development, showcasing the interconnectedness of ion transport and plant physiology.
Long Distance Transport – Xylem Loading And Unloading
Long-distance transport in plants involves the movement of essential mineral elements from the roots to the shoots, primarily through xylem vessels. This process is vital for plant health and function, as it facilitates the distribution of nutrients and supports physiological activities. The mechanisms of xylem loading and unloading are intricately connected to gradients of hydrostatic pressure and water potential. The following points outline the key aspects of this transport mechanism.
- Hydrostatic Pressure and Water Potential:
- Once mineral elements enter the xylem vessels, their transport from the root to the shoot is driven by root pressure, which generates hydrostatic pressure within the xylem.
- Additionally, the gradient of water potential between the roots and the shoots is crucial for this process. During the day, when stomata are open, the water potential gradient is steep, facilitating efficient upward transport. The typical gradient follows this pattern: atmosphere < leaf cells < xylem sap < root cells < external solution (soil).
- Unidirectional Transport:
- Transport in xylem vessels is primarily unidirectional, meaning that water and dissolved minerals move from the roots upward to the leaves.
- An increase in transpiration rate, which is the process of water vapor loss from plant surfaces, enhances both the uptake of minerals from the soil and their translocation through the xylem.
- Lateral Transport via Xylem Transfer Cells:
- The lateral transport of ions from the xylem to the leaves is facilitated by specialized cells known as xylem transfer cells. These cells possess two distinctive features that enhance their function:
- The cell walls facing the xylem are intricately corrugated and invaginated, increasing the surface area available for absorption. This structural adaptation enhances the efficiency of ion uptake.
- Xylem transfer cells are rich in mitochondria, which are strategically located near the convoluted and corrugated walls. This arrangement provides ATP, the energy currency of the cell, necessary for active transport processes across the walls.
- The lateral transport of ions from the xylem to the leaves is facilitated by specialized cells known as xylem transfer cells. These cells possess two distinctive features that enhance their function:
- Role of Transfer Cells:
- Transfer cells are not exclusive to xylem; they are also found in regions where substantial quantities of ions or organic solutes are moved into or out of conducting cells or storage tissues. Their role is essential for ensuring that ions and nutrients are efficiently managed within the plant.
- Phloem Mobility and Retranslocation:
- While xylem primarily transports water and mineral elements, some minerals that are phloem-mobile can be retranslocated from the shoot back to the root through the phloem.
- The main substance transported in the phloem is sucrose, along with other organic compounds. Unlike the unidirectional transport in the xylem, the transport in phloem is bidirectional, allowing the movement of nutrients in response to the nutritional demands of various plant organs or tissues.