What is Root knot nematode?
- Root-knot nematodes (RKN), specifically from the genus Meloidogyne, are microscopic, parasitic worms that attack the roots of a wide range of plants. These nematodes thrive in warm climates or regions with short winters, making them a significant threat in tropical and subtropical areas. They infect over 2,000 plant species globally, leading to substantial crop losses—up to 5% of worldwide yield. Among the different species, Meloidogyne incognita is particularly notorious, causing around $100 billion in crop damage annually.
- RKNs are particularly harmful because they infect the plant’s root system, causing root-knot galls—swellings that disrupt the normal nutrient and water flow within the plant. These galls drain essential nutrients, stunting the plant’s growth and reducing its resistance to environmental stresses like drought, as well as to other diseases. Infected young plants may die, while mature plants suffer from reduced growth and significantly lower yields.
- The damage caused by root-knot nematodes does not result in dramatic outbreaks like many other plant diseases. Instead, the impact is gradual, with crop production declining over several seasons. Symptoms can be hard to diagnose, as above-ground signs such as wilting, yellowing, and stunted growth may resemble nutrient deficiencies or water stress. This makes it difficult for farmers to detect RKN infections until they have caused substantial damage.
- One of the most challenging aspects of controlling root-knot nematodes is their sedentary endoparasitic lifestyle, meaning they live and feed inside the plant’s roots without moving, making them difficult to eliminate. Various methods have been developed for managing RKNs, including the use of resistant plant varieties, soil treatments such as solarization (heating the soil using plastic covers), chemical nematicides, and biological controls. Chemical treatments, while effective, are expensive and often lead to harmful environmental consequences. Therefore, modern agricultural practices increasingly emphasize eco-friendly solutions, such as the use of resistant germplasm and biological control agents.
- In recent years, researchers have made significant progress in understanding the biology of RKNs, particularly their life cycle and interactions with host plants. These advances are crucial for developing effective management strategies. Current research focuses on sustainable and environmentally friendly approaches, like utilizing bio-control agents—organisms that naturally attack or suppress nematodes—as well as enhancing resistant crop varieties to reduce dependency on chemical interventions.
Life cycle of Meloidogyne incognita
The life cycle of Meloidogyne incognita, a root-knot nematode, follows a highly specialized sequence of stages that allows it to effectively parasitize plant roots. The process begins with the egg and progresses through four juvenile stages (J1, J2, J3, J4) before reaching adulthood. Understanding these stages is crucial for effective management of this pest, as each phase plays a specific role in the nematode’s survival and reproduction.
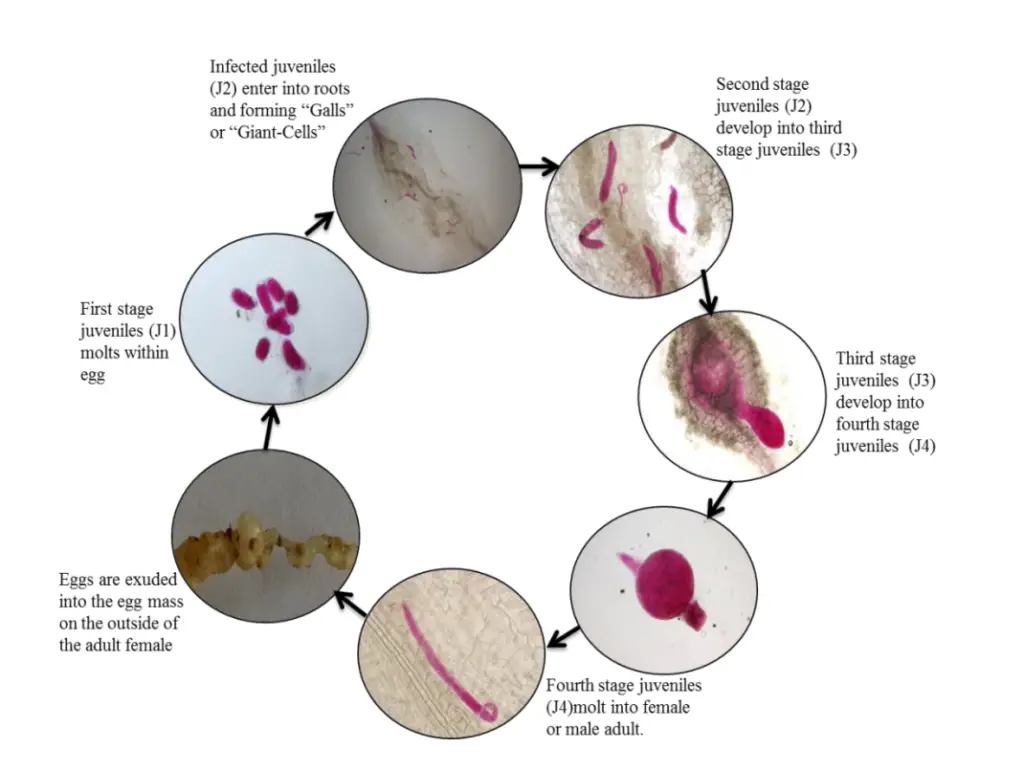
- Egg Stage: The life cycle starts when a mature female nematode lays eggs in a gelatinous matrix, which is secreted from her rectal glands. This glycoprotein matrix not only helps in egg deposition but also protects the eggs from environmental stresses and predators. These eggs are often found within root galls or on the surface of these galls. As the gelatinous mass matures, it changes from soft and transparent to firm and dark brown. Importantly, egg hatching is temperature-dependent and does not require any stimulus from the host plant.
- Juvenile Stage (J1 and J2): The first juvenile stage (J1) develops within the egg and molts into the second juvenile stage (J2) while still inside the egg. The J2 juvenile is the infective stage and is the only stage capable of penetrating plant roots. J2s are highly sensitive to their environment and can remain dormant if a suitable host is not available. When conditions become favorable, particularly when the presence of a host plant is detected, the J2 hatches and migrates toward the plant roots. These J2 nematodes are attracted to the plant’s root exudates and actively seek entry points near the root tip, often moving through intercellular spaces to reach the vascular tissues.
- Infection and Sedentary Development: Once inside the plant’s root, the J2 juvenile becomes sedentary. It establishes a feeding site by transforming nearby root cells into giant cells (GC), which serve as a nutrient source. These giant cells are induced by the nematode and are crucial for its growth and development, as they provide a continuous supply of water and nutrients. During this stage, the nematode undergoes two more molts (J3 and J4), each molt involving the shedding of its cuticle.
- Juvenile Stages (J3 and J4): In the third juvenile stage (J3), the nematode remains stationary, relying on the giant cells for nourishment. By the time it molts into the fourth juvenile stage (J4), it prepares to differentiate into an adult. The nematode’s sex is determined by environmental conditions. In nutrient-scarce or stressful environments, more males are produced, which are motile and leave the host in search of mates. However, in favorable conditions, the nematode will usually develop into a female, which remains sedentary.
- Adult Stage: The adult stage marks the sexual maturity of the nematode. Females, which remain within the plant roots, reproduce predominantly through mitotic parthenogenesis, meaning that they can produce offspring without fertilization. After mating, the female lays eggs within the plant tissue or on the root surface, encased in the protective gelatinous mass, thereby completing the cycle.
- Environmental Factors and Sex Determination: The sex ratio of M. incognita is influenced by external factors, with adverse conditions promoting the development of males. Males are motile, meaning they leave the plant to seek mating opportunities, while females stay sedentary within the roots, continuously producing egg masses to propagate the next generation.
Symptoms produced by Meloidogyne incognita
Meloidogyne incognita, commonly known as root-knot nematodes, are a significant agricultural pest that infects a wide range of plants, leading to a variety of symptoms. These symptoms not only impair plant growth but also make it difficult for farmers to distinguish between nematode infection and nutrient deficiencies. Understanding these symptoms is critical for early detection and effective management.
- Nutritional Deficiency Symptoms: Plants infected by M. incognita often show signs of nutrient deficiency. These include yellowing of leaves, known as chlorosis, stunted growth, wilting, and, in severe cases, plant death. Since these symptoms closely resemble those caused by inadequate nutrients or water, farmers may misinterpret nematode damage as a simple deficiency, delaying proper intervention. Chlorosis occurs because the nematodes interfere with the plant’s ability to absorb essential nutrients and water, weakening its overall health.
- Root Galls and Structural Damage: One of the most distinctive symptoms of M. incognita infection is the formation of galls or swellings on the roots. These galls result from the nematodes establishing feeding sites within the plant roots, causing abnormal cell division and expansion. As a result, the infected roots become bushier and shorter compared to healthy roots, which hinders the plant’s ability to take up water and nutrients efficiently. These galls can disrupt the plant’s vascular system, leading to reduced overall growth.
- Wide Host Range: M. incognita is known to infect over 3,000 different plant species, making it one of the most versatile and challenging pests in agriculture. Because of this broad host range, it is difficult to identify common crops that are not affected by root-knot nematodes. The nematodes’ adaptability allows them to infest a variety of plants, from vegetables and fruits to ornamental species, making them a widespread issue in farming.
- Lesions and Secondary Infections: In addition to the direct damage caused by feeding, M. incognita can create small lesions or openings in plant tissues. These lesions serve as entry points for secondary pathogens, such as bacteria, fungi, and viruses. Once these pathogens gain access through the damaged tissue, they can cause further infections, compounding the harm to the plant. This secondary infection process exacerbates the impact of nematode infestations, further reducing plant health and yield.
- Stunted Root Development: Beyond the formation of galls, infected plants exhibit abnormal root systems. The roots become shorter and more fibrous, losing their natural length and density. This stunted root development diminishes the plant’s ability to anchor itself in the soil and draw water from deeper layers, making it more susceptible to drought and other environmental stresses.
- Difficulty in Diagnosis: One of the most problematic aspects of M. incognita infections is the difficulty in diagnosing the damage based on above-ground symptoms. The visible signs, such as yellowing leaves and stunted growth, can easily be mistaken for nutrient or water deficiencies, leading to mismanagement of the problem. Therefore, careful examination of the root system, where the galls and structural damage are more evident, is essential for accurate diagnosis.
Identification of Meloidogyne incognita
Identifying Meloidogyne incognita, a significant root-knot nematode species, involves a combination of morphological, molecular, and isoenzymatic techniques. Each method provides specific insights into the organism’s characteristics, allowing for more accurate and reliable diagnosis. Given the nematode’s economic impact on agriculture, precise identification is essential for the development of effective management strategies.
- Morphological Identification: Traditionally, the morphology of female nematodes has been a primary method of identification, particularly the study of the perineal pattern. This pattern, located at the rear of female nematodes, exhibits distinct features such as striations and shapes that vary between species. However, in some cases, the morphological characteristics of M. incognita may overlap with those of other nematode species, complicating accurate identification based on appearance alone. Therefore, morphological analysis often serves as a preliminary step rather than a conclusive diagnosis.
- Molecular Identification: To overcome the limitations of morphological analysis, molecular techniques are employed for more precise identification. Several molecular markers and DNA-based methods have been developed, targeting specific regions of the nematode’s genome. For instance, the ribosomal Large Subunit (LSU) D2/D3 expansion segments and Small Subunits (SSU) are commonly used markers. These regions contain sequences that are conserved among nematodes, allowing researchers to differentiate between closely related species. Additionally, mitochondrial DNA has been analyzed to provide further resolution, as these markers are highly variable and species-specific. Other methods like Random Amplified Polymorphic DNA (RAPD) and internal transcribed spacers (ITS) also help in the differentiation of nematode species.
- Isoenzyme Phenotyping: Another method of identification is isoenzyme phenotyping, which involves analyzing the enzymes extracted from mature females of M. incognita. By using gel electrophoresis, researchers can observe the relative mobility of specific enzymes, such as esterases and malate dehydrogenases. The isoenzyme patterns differ between species, making this method a useful biochemical tool for confirming identification, especially when combined with molecular data.
- Combined Approach: While each identification method offers valuable information, no single technique is flawless. Therefore, combining morphological, molecular, and biochemical data provides a more comprehensive and reliable diagnosis. By cross-referencing results from various approaches, researchers can ensure greater accuracy in identifying Meloidogyne incognita, minimizing the potential for misidentification.
Management strategies of Meloidogyne incognita
Managing Meloidogyne incognita, a destructive root-knot nematode, is vital for protecting crops and maintaining high agricultural yields. Multiple strategies, including chemical, biological, cultural controls, and the use of resistant cultivars, have been employed to mitigate its impact. Each method offers unique advantages and limitations, often requiring a combined approach to achieve effective control.
- Chemical Control:
Chemical nematicides have traditionally been one of the most effective tools for managing Meloidogyne spp., particularly in large-scale agriculture. Nematicides are grouped into fumigant and non-fumigant types, depending on their volatility in soil. Fumigants, such as chloropicrin, metam sodium, and 1,3-dichloropropene, are highly effective but also highly toxic. Non-fumigants, including oxamyl and fluensulfone, are less volatile but still carry environmental risks. Although nematicides can significantly reduce nematode populations, they cannot fully eliminate them and often accumulate in plant tissues, posing risks to the ecosystem and human health. Moreover, many nematicides contain banned or restricted compounds, such as methyl bromide, due to their harmful environmental impact. - Biological Control:
Biological control is increasingly favored for its environmental safety. Various fungi, such as Trichoderma, Acremonium, and Chaetomium, have demonstrated antagonistic effects against plant-parasitic nematodes. These fungi colonize plant roots, thereby reducing nematode penetration and feeding. For example, Trichoderma species can suppress M. incognita populations before they establish feeding sites in the host plant. Similarly, saprophytic fungi have been shown to reduce nematode penetration rates in crops like tomatoes. Additionally, symbiotic relationships with arbuscular mycorrhizal (AM) fungi and endophytes like Fusarium oxysporum can alter nematode behavior and enhance plant resistance. These biological agents often induce systemic resistance in plants, making them a promising option for sustainable nematode control. - Cultural Control:
Cultural practices are essential in integrated nematode management. Techniques such as crop rotation, intercropping, and the use of clean planting materials can significantly reduce nematode populations. Crop rotation with non-host species disrupts the nematode’s life cycle, while intercropping can minimize nematode spread. Additionally, soil solarization—a method of heating the soil using clear plastic to trap solar radiation—has been effective in reducing M. incognita egg viability. Solarizing the soil for 3-4 weeks before planting can drastically reduce nematode populations. Cleaning farm implements and using certified disease-free seeds also help limit the spread of nematodes between fields. - Resistant Cultivars:
Developing and planting resistant cultivars is one of the most sustainable approaches to nematode management. Several resistance genes have been identified in various crops. For instance, the Mi-1.2 gene in tomatoes confers strong resistance against high nematode pressures, while the Rmc-1 gene in wild potatoes provides resistance against multiple Meloidogyne species. Similarly, the Me and N genes in pepper plants offer significant protection. However, the emergence of resistance-breaking nematode strains poses a challenge, necessitating ongoing research to develop new resistant varieties. - Integrated Approach:
No single method offers a complete solution for managing M. incognita. Therefore, an integrated approach combining chemical, biological, cultural, and genetic strategies is often the most effective. For example, the use of resistant cultivars in conjunction with soil solarization and biological control agents can reduce reliance on chemical nematicides, thereby promoting environmental sustainability.
Morphological identification of root knot nematodes (Meloidogyne Spp.)
The following provides a detailed overview of the perineal patterns observed in four economically important Meloidogyne species.
- Meloidogyne incognita:
- The perineal pattern is characterized by smooth and wavy striae, sometimes exhibiting a zigzag arrangement.
- Notably, lateral lines are absent in this species.
- A distinct squarish high dorsal arch is present, encircling the tail terminus with a prominent whorl, which aids in its identification.
- Meloidogyne javanica:
- This species displays smooth, somewhat wavy striae.
- A distinctive feature is the presence of lateral lines or ridges that traverse the pattern, fading toward the tail terminus.
- The dorsal arch can be low and rounded or occasionally high and squarish, frequently adorned with a whorl in the tail terminus region.
- Meloidogyne arenaria:
- The striae in this species are smooth and slightly wavy, often extending laterally to form wing-like projections on one or both sides of the pattern.
- Unlike the previous species, M. arenaria does not have distinct lateral ridges; instead, its pattern features forked and irregular lateral fields.
- The dorsal arch is typically low and exhibits indented regions near the lateral fields, creating rounded shoulders.
- Meloidogyne hapla:
- The perineal pattern consists of close, smooth, and wavy striae, with some patterns developing wings on one or both lateral sides.
- The area between the anus and tail terminus is marked by stippled subcuticular punctuations, providing a unique identification characteristic.
- Lateral ridges are absent; however, irregularities in the striae can be observed in the lateral fields.
- The dorsal arch usually appears low and rounded but may also present as high and squarish in some instances.
Molecular diagnostics of root knot nematodes (Meloidogyne Spp.)
Molecular diagnostics of root knot nematodes (Meloidogyne spp.) have become essential for accurate species identification and effective management of these economically significant agricultural pests. Traditional morphological methods, while useful, can be time-consuming and require specialized training. Molecular techniques, particularly those involving DNA amplification and sequencing, offer rapid, specific, and sensitive alternatives. The following narrative outlines the processes and methodologies involved in the molecular diagnostics of Meloidogyne species, particularly focusing on DNA isolation and polymerase chain reaction (PCR) amplification techniques.
- DNA Isolation:
- The initial step in molecular diagnostics is the extraction of DNA from nematodes. Newly hatched second-stage infective juveniles (J2) and male Meloidogyne nematodes are meticulously selected using a small needle and placed in 15 μL of Worm Lysis Buffer (WLB) on a glass slide. The nematodes are then cut into two pieces with a stainless steel blade under a stereomicroscope.
- For female nematodes, tweezers are employed to handle them, and they are crushed using a pipette tip to release their internal contents.
- The cut nematode fragments are transferred into a 0.5 mL centrifuge tube containing an additional 10 μL of WLB. This mixture is then centrifuged at 13,500 rpm for 2 minutes.
- Subsequently, the tubes are placed at −80 °C for 15 minutes to promote cell lysis. Afterward, 7 μL of mineral oil is added to each tube, followed by incubation at 60 °C for 1 hour and then at 90 °C for 10 minutes.
- The mineral oil is removed using a pipette, and the aqueous sample is stored at −20 °C until further analysis.
- Composition of Worm Lysis Buffer (WLB):
- The effectiveness of the DNA extraction process is heavily reliant on the composition of the WLB, which typically includes:
- Potassium chloride (50 mM)
- Tris buffer (10 mM, pH 8.2)
- Magnesium chloride (2.5 mM)
- Proteinase K (60 μg/mL)
- NP40 (0.45%)
- Tween 20 (0.45%)
- Gelatin (0.01%)
- The effectiveness of the DNA extraction process is heavily reliant on the composition of the WLB, which typically includes:
- PCR Amplification:
- Polymerase chain reaction (PCR) is employed to amplify specific DNA sequences from the extracted nematode DNA. Various specific SCAR (Sequence Characterized Amplified Region) primers have been developed for the identification of different Meloidogyne species.
- The general PCR reaction mixture consists of:
- 0.5 μL of extracted DNA
- 0.5 μL of each 10-μM primer
- 2.5 μL of 10 × buffer
- 1.5 μL of 50 mM MgCl₂
- 2.5 μL of 200 mM each dNTP
- 2 units of DNA polymerase
- Amplification Process:
- The PCR amplification typically follows these steps:
- Initial Denaturation: 94 °C for 2 minutes
- Denaturation: 94 °C for 30 seconds
- Annealing: Variable temperatures depending on the specific primers (e.g., 50 °C for some primers, 54 °C for others)
- Extension: 72 °C for 1 to 90 seconds, depending on the primer set
- Final Extension: 72 °C for 7 minutes
- The process is repeated for 45 cycles to ensure sufficient amplification.
- The PCR amplification typically follows these steps:
- Specific Primers and Amplification Sizes:
- The following specific SCAR primers can be used for identifying various Meloidogyne species, along with their corresponding amplification sizes:
- Meloidogyne incognita: 720 bp (Blok et al., 1997)
- Meloidogyne javanica: 720 bp (Zijlstra et al., 2000)
- Meloidogyne arenaria: 420 bp (Zijlstra et al., 2000)
- Meloidogyne hapla: 440 bp (Wishart et al., 2002)
- Other species-specific primers can yield different sizes, such as Meloidogyne mayaguensis (780 bp) and Meloidogyne fallax (670 bp).
- The following specific SCAR primers can be used for identifying various Meloidogyne species, along with their corresponding amplification sizes:
Mode of reduction of Meloidogyne incognita
Reducing the impact of Meloidogyne incognita—a major agricultural pest responsible for root-knot disease—requires a comprehensive understanding of various biological, chemical, and environmental factors. Several key modes of action can be employed to limit the population and pathogenicity of this nematode. These include inhibition of nematode development, fostering antagonistic microbiota, enhancing plant growth and morphology, and inducing plant resistance. Each method contributes to a holistic strategy that mitigates nematode damage while promoting sustainable agricultural practices.
- Inhibition of Nematode Activity:
The direct inhibition of M. incognita involves strategies that disrupt its life cycle or prevent its ability to infect plant roots. This can be achieved through the use of nematicides or biological agents that either kill the nematodes or prevent them from establishing feeding sites within plant tissues. In many cases, chemical inhibitors target the nematode’s ability to penetrate the root zone, while biological agents like fungi and bacteria create barriers to nematode movement and reproduction. - Development of Antagonistic Microbiota:
Certain microorganisms, particularly fungi and bacteria, play a significant role in reducing M. incognita populations by acting as natural antagonists. These microorganisms either parasitize the nematodes or produce compounds that deter nematode feeding and reproduction. For instance, fungi such as Trichoderma harzianum and Fusarium oxysporum have been shown to alter root exudates and create an inhospitable environment for nematode larvae, thus preventing them from invading host plants. By colonizing the rhizosphere, these antagonistic microbes outcompete nematodes for resources and physically block their access to the roots. - Enhanced Plant Growth and Morphology:
Improving plant health and growth can naturally reduce the susceptibility of crops to nematode infections. Plants that grow more robustly due to optimal nutrition and environmental conditions tend to exhibit fewer symptoms of nematode attack. Additionally, certain microorganisms can promote plant growth while simultaneously inhibiting nematode activity. These plant growth-promoting rhizobacteria (PGPR) enhance root development and overall plant vigor, making it more difficult for nematodes to establish themselves. Enhanced plant growth often correlates with an increased ability to tolerate or resist nematode-induced damage. - Induced Resistance:
One of the most promising methods for controlling M. incognita is through induced resistance, where plants develop enhanced defensive capabilities after exposure to specific inducers. This process can be local or systemic, with the latter involving a plant-wide response to nematode invasion. Two main pathways—Induced Systemic Resistance (ISR) and Systemic Acquired Resistance (SAR)—are involved in this process. ISR is typically activated by microorganisms such as Trichoderma or PGPR and relies on the jasmonic acid (JA) and ethylene (Et) signaling pathways. In contrast, SAR is triggered by pathogens and involves the salicylic acid (SA) pathway. Both pathways lead to the production of defense-related proteins and compounds that help plants fend off nematode infections. - Use of Inducers:
Plants can also be primed for resistance through the application of biotic and abiotic inducers. Biotic inducers include beneficial microorganisms such as fungi and rhizobacteria, which activate defense mechanisms through JA, SA, and Et pathways. Abiotic inducers, such as certain chemicals, can also trigger plant defenses, although their use is less common due to concerns about environmental impact and potential negative effects on plant health at high concentrations. These inducers enhance the plant’s immune response, making it less susceptible to nematode infection. - Seed Coating:
Seed coating with resistance-inducing agents offers an additional strategy for protecting young plants from nematode invasion. Coating seeds with beneficial fungi, bacteria, or elicitors of plant defenses can improve germination rates, enhance seedling vigor, and provide early-stage protection against nematodes. For example, fermentation liquids from fungi like Penicillium chrysogenum have been shown to induce resistance in crops such as tomatoes and cucumbers, reducing the incidence of root-knot disease.
Effective management techniques of RKN
Root-knot nematodes (RKN), particularly Meloidogyne species, represent a significant threat to various crops, causing substantial yield losses. Effective management of RKN requires a combination of control techniques, as complete eradication is not possible. Instead, various management strategies can be employed to mitigate the impact of RKN on crops. These methods include chemical, biological, and cultural approaches, as well as the use of resistant cultivars. By integrating these strategies, growers can effectively manage RKN populations and reduce damage to crops.
- Chemical Control: The use of synthetic nematicides can suppress RKN populations by inhibiting nematode reproduction and movement. Cadusafos, an organophosphate-based nematicide, is particularly effective in controlling RKN. Studies show that it causes 100% mortality of juveniles and inhibits egg hatching. Emamectin benzoate, another effective nematicide derived from Streptomyces avermitilis, has been shown to significantly reduce gall formation and improve plant growth in infested crops. Although synthetic chemicals like methyl bromide have been banned due to environmental concerns, alternatives such as 1,3-dichloropropene and chloropicrin have proven effective in controlling nematodes in field trials.
- Botanical Nematicides: Certain plant-derived compounds, such as those from Melia azedarach (chinaberry), exhibit nematicidal properties. Aqueous extracts from chinaberry fruits have been shown to reduce RKN populations in soil and roots, while also activating host plant defenses. The use of botanicals provides a more environmentally friendly option compared to synthetic chemicals, offering broad-spectrum activity against pests with fewer negative effects on non-target organisms.
- Biological Control: Nematophagous bacteria such as Pseudomonas fluorescens play an important role in managing RKN. These bacteria produce antibiotics, such as 2,4-diacetylphloroglucinol (DAPG), which suppress nematode populations and improve plant resistance. Studies have shown that seed treatment with P. fluorescens can lower nematode populations in several crops, including corn, cotton, and tomato. In combination with other beneficial microorganisms like Trichoderma viride, these bacteria can enhance plant resistance and promote growth.
- Nematophagous Fungi: Several fungi, such as Trichoderma longibrachiatum, have been identified as effective biological control agents against RKN. These fungi parasitize and kill nematode juveniles by penetrating their integument and releasing lethal metabolites. Studies show that T. longibrachiatum can inhibit over 88% of nematode juveniles, reducing gall formation in plants. Other nematophagous fungi, such as Paecilomyces lilacinus and Syncephalastrum racemosum, also show strong ovicidal and larvicidal effects against RKN.
- Essential Oils: Essential oils derived from plants such as Mentha and Eucalyptus have shown nematicidal properties due to their volatility and environmental safety. Recent studies have demonstrated that essential oils can reduce nematode populations and gall formation in crops like tomato. Oils from Eucalyptus globules and Pelargonium asperum have proven particularly effective when applied to soil at appropriate concentrations, making them a viable alternative to synthetic fumigants.
- Resistant Cultivars: Using crop varieties that are resistant to RKN is one of the most effective and sustainable management techniques. Resistant cultivars, such as those in okra and pepper, contain genetic traits that prevent RKN reproduction and reduce root damage. Breeding programs aim to develop new resistant cultivars by incorporating resistance genes like the N and Me genes. These genes, however, may be less effective at high temperatures, making it crucial to select cultivars based on environmental conditions.
Importance of Root knot nematode
- Economic Impact:
- RKNs are responsible for substantial yield losses in a wide range of crops, including vegetables, fruits, and ornamental plants. It is estimated that they can cause global crop losses exceeding $100 billion annually.
- The presence of root knot nematodes can lead to increased production costs due to the need for additional pest management measures, such as nematicides and soil amendments.
- Host Range:
- Meloidogyne species have a broad host range, infesting over 3,000 different plant species. This wide adaptability allows them to thrive in various agricultural settings, increasing their potential impact on food security.
- Important crops affected include tomatoes, potatoes, soybeans, cotton, and many other economically vital plants.
- Damage Mechanism:
- RKNs infect plant roots, forming galls or “knots” that disrupt normal root function. This leads to impaired water and nutrient uptake, reducing overall plant vigor and yield.
- Infected plants may exhibit symptoms such as stunted growth, yellowing of leaves, wilting, and poor fruit development, making them more susceptible to other pests and diseases.
- Soil Health and Ecosystem Impact:
- Root knot nematodes can alter soil health by affecting microbial communities in the rhizosphere. The disruption of beneficial microbial populations can lead to imbalances in nutrient cycling and soil fertility.
- They can also influence the dynamics of plant communities, potentially leading to reduced biodiversity and altered ecosystem functions.
- Resistance and Management Challenges:
- The management of root knot nematodes is complicated by their ability to develop resistance to nematicides and other control measures. This necessitates integrated pest management (IPM) strategies that combine biological, chemical, and cultural practices.
- The development and deployment of resistant plant varieties are crucial in managing RKN populations. However, the continuous evolution of nematode populations can overcome resistance traits, making ongoing research essential.
- Research and Knowledge Development:
- The study of root knot nematodes is vital for understanding plant-nematode interactions, nematode biology, and the development of effective management strategies. Research in molecular diagnostics, host resistance, and biological control offers promising avenues for future advancements in nematology.
- Subedi, Sudeep & Thapa, Bihani & Shrestha, Jiban. (2020). Root-knot nematode (Meloidogyne incognita) and its management: a review. 3. 21-31. 10.3126/janr.v3i2.32298.
- https://aloki.hu/pdf/1801_16791690.pdf
- https://iivr.icar.gov.in/sites/default/files/Technical%20Bulletins/Final%20Bulletin_76.pdf
- https://en.wikipedia.org/wiki/Root-knot_nematode
- https://agritech.tnau.ac.in/crop_protection/nematology/crop_prot_nematode_veg_root_nemo.html
- https://www.biologydiscussion.com/plants/plant-diseases/root-knot-disease-of-rice-caused-by-meloidogyne-graminicola-plant-diseases/43279
- https://plantpathology.ca.uky.edu/files/ppfs-vg-28.pdf
- https://ipm.uga.edu/files/2022/02/Root-Knot-Nematode.pdf