What is Insect Plant Interaction?
- Insect-plant interactions encompass a diverse array of relationships between insects and plants, which can be classified into three primary categories: antagonism, commensalism, and mutualism. These interactions are fundamental to ecological dynamics and have significant implications for agriculture, horticulture, and forestry.
- Antagonism represents a category where insects adversely affect plants. This harm typically manifests through feeding behaviors that damage plant tissues, leading to compromised growth, reproductive failures, or disease susceptibility. For instance, herbivorous insects such as caterpillars and beetles consume leaves, stems, or roots, directly influencing the plant’s overall health and viability. This interaction not only affects individual plants but can also disrupt local ecosystems, as the loss of plant biomass impacts herbivores and higher trophic levels.
- Commensalism, on the other hand, involves a relationship where one organism benefits without significantly affecting the other. Certain insects may inhabit plants, deriving shelter or sustenance without inflicting harm. An example includes aphids residing on a plant’s surface; while they may extract sap, the overall effect on the plant can be negligible if their populations remain low. This dynamic highlights the complexity of interactions where organisms coexist with minimal impact on one another.
- Mutualism exemplifies a symbiotic relationship where both insects and plants derive benefits. A prominent example of this interaction is seen in pollinators like bees. As these insects collect nectar from flowers, they inadvertently facilitate the transfer of pollen, promoting fertilization and seed production in plants. This reciprocal relationship enhances plant reproductive success while providing insects with essential nutrients. Such interactions underscore the interdependence of species within ecosystems.
- These interactions manifest across various biological levels, from individual plants to entire communities, reflecting intricate biochemical and ecological processes. The co-evolution of insects and plants has led to remarkable adaptations. For example, plants may produce secondary metabolites—chemical compounds that serve dual purposes: deterring herbivorous insects and attracting beneficial species such as pollinators or predators of herbivores.
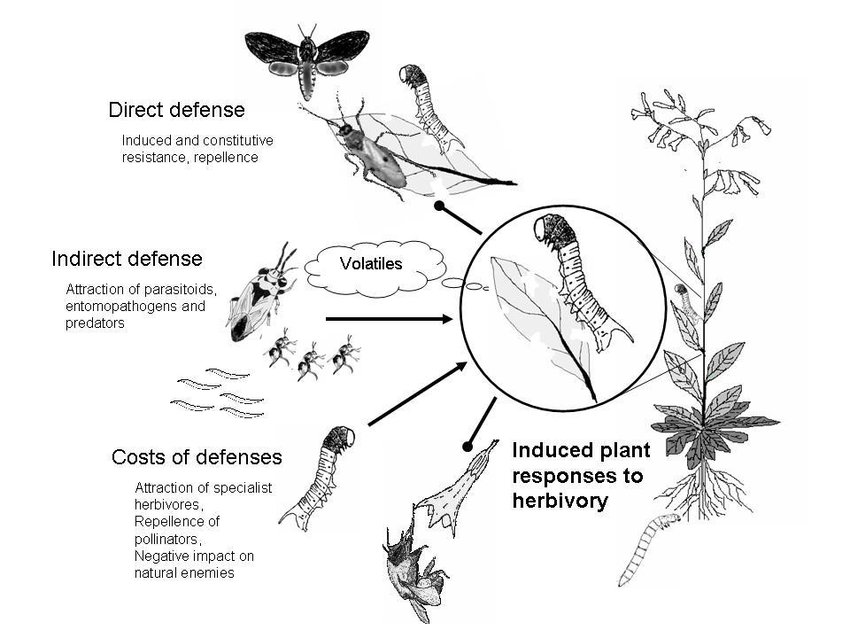
What are the main types of interactions between insects and plants?
Insect-plant interactions are essential components of ecological dynamics, influencing biodiversity, ecosystem functioning, and agricultural productivity. These interactions can be categorized into three primary types: antagonism, commensalism, and mutualism. Each type encompasses specific relationships characterized by distinct functional roles and consequences for both insects and plants.
- Antagonism: This category involves negative impacts on plants due to insect activity. Here are some key points about antagonistic interactions:
- Feeding Damage: Herbivorous insects, such as caterpillars and beetles, consume various plant parts—leaves, stems, and roots. This feeding can lead to significant physical damage, inhibiting growth and development.
- Reproductive Impairment: Damage to floral structures can reduce the plant’s ability to produce seeds and fruits, thereby limiting reproductive success.
- Disease Transmission: Insects may serve as vectors for plant pathogens, introducing diseases that can devastate crops and natural flora. For example, aphids are known to transmit viruses that severely impact plant health.
- Economic Impact: The economic ramifications of antagonistic interactions can be substantial, leading to increased costs in pest management and losses in agricultural yield.
- Commensalism: In this type of interaction, one organism benefits without affecting the other. Important aspects include:
- Habitat Utilization: Some insects utilize plants for shelter, using leaves or stems as a protective environment without causing significant harm.
- Minimal Impact: The presence of these insects does not notably influence the plant’s growth or reproductive capabilities, allowing both organisms to coexist without detriment.
- Example Species: Certain scale insects may live on a plant’s surface, extracting minimal resources while leaving the plant relatively unscathed.
- Mutualism: This interaction is characterized by reciprocal benefits for both organisms involved. Key features include:
- Pollination: Insects such as bees and butterflies play crucial roles in the pollination process. As they collect nectar for sustenance, they inadvertently transfer pollen, aiding in the fertilization of flowers.
- Nutrient Exchange: In return for pollination services, plants provide food resources in the form of nectar or pollen, creating a symbiotic relationship that enhances reproductive success for both parties.
- Seed Dispersal: Some insects contribute to seed dispersal, assisting plants in colonizing new areas and promoting genetic diversity.
Theory of co-evolution
The theory of co-evolution posits that reciprocal evolutionary changes occur between interacting species, meaning that the evolutionary trajectory of one species influences and is influenced by another. This concept, initially described in the context of the relationships between insects and flowering plants, has since been expanded to encompass a wide range of biological interactions. The implications of co-evolution are profound, affecting various biological and ecological phenomena.
- Foundation of Co-evolution:
- Co-evolution suggests that the evolutionary adaptations of one species can lead to corresponding adaptations in another. This dynamic interplay is critical for understanding the complexities of biodiversity and ecosystem stability. The theory illustrates how species are not isolated entities but are interconnected through their evolutionary histories.
- Examples of Co-evolution:
- Predator-Prey Relationships: The interaction between predators and their prey exemplifies co-evolution. As predators evolve traits that enhance their hunting efficiency—such as increased speed or improved camouflage—their prey concurrently develop defenses to evade capture. These defenses may manifest as warning coloration, mimicry, or physical adaptations like spines or toxins. This ongoing “arms race” drives diversification within both groups and contributes to the evolutionary pressure on each species.
- Herbivore-Plant Interactions: In the context of herbivores and plants, plants have developed a wide array of physical and chemical defenses to deter herbivory. These defenses include structural features such as thorns and spines, as well as chemical compounds like phenolics and terpenoids. In response, herbivores have evolved specialized adaptations, such as robust digestive systems or the ability to detoxify harmful chemicals, allowing them to exploit plant resources effectively.
- Mutualistic Relationships: Co-evolution can also lead to mutualistic interactions, where both species benefit from their relationship. A notable example is the relationship between acacia ants and acacia trees. The trees provide food and shelter for the ants, while the ants offer protection against herbivores. Such mutualism exemplifies how co-evolution can enhance the survival and reproductive success of both partners, fostering a symbiotic relationship that is essential for their coexistence.
- Flowering Plants and Pollinators: The relationship between flowering plants and their pollinators is another prominent example of co-evolution. Many flowers have evolved striking colors and enticing scents to attract various pollinators, including bees, butterflies, and birds. In turn, these pollinators have developed specialized features to efficiently extract nectar and pollen. This co-adaptation is crucial for the reproductive success of many plant species, highlighting the interconnectedness of organisms in ecosystems.
- Role of Allelochemicals:
- Allelochemicals, which are secondary metabolites produced by plants, algae, bacteria, and fungi, also play a significant role in co-evolutionary processes. These compounds can influence the growth and development of other organisms, often serving as a means of chemical defense against competition and herbivory. For example:
- Reduction of Chlorophyll Production: Certain allelochemicals can inhibit chlorophyll synthesis, thereby reducing a plant’s ability to photosynthesize. This suppression can lead to diminished growth or even death in affected plants.
- Disruption of Plant Growth Regulators: Allelochemicals can alter levels of essential plant growth regulators, resulting in imbalances in phytohormones. Such disruptions may inhibit normal growth and development, impacting overall plant fitness.
- Allelochemicals, which are secondary metabolites produced by plants, algae, bacteria, and fungi, also play a significant role in co-evolutionary processes. These compounds can influence the growth and development of other organisms, often serving as a means of chemical defense against competition and herbivory. For example:
- Ecological Implications:
- The theory of co-evolution underscores the interconnectedness of species within ecosystems. Each evolutionary change in one species can create cascading effects that alter the dynamics of entire communities. This web of interdependencies contributes to the richness and complexity of biodiversity. Moreover, understanding co-evolution can inform conservation efforts, as it emphasizes the need to consider species interactions when managing ecosystems.
Phytophagous insect and their host finding mechanism
Phytophagous insects, commonly known as plant-eating insects, encompass a vast array of species that feed on various parts of plants, including roots, leaves, stems, and even whole plants. These insects are crucial components of ecosystems, playing significant roles in nutrient cycling, pollination, and even as prey for other organisms. Their host-finding mechanisms are complex and involve several sensory modalities and behavioral strategies.
- Diversity and Classification:
- Phytophagous insects represent approximately 25% of all insect species, with around 500,000 known species. They can be classified into two main categories: monophagous species, which feed on a single plant species, and polyphagous species, which can feed on multiple plant species. This diversity allows them to adapt to various ecological niches.
- Feeding Mechanisms:
- The feeding behavior of phytophagous insects varies significantly across different developmental stages. Their mouthparts are specialized for different feeding strategies, impacting their food choice and host selection. External feeders consume plant material directly, while internal fluid feeders extract sap or other internal fluids from plants.
- Host Finding Mechanism:
- The process of locating suitable host plants involves several critical steps: searching, landing, and contact evaluation. Each step relies on a combination of sensory inputs.
- Sensory Modalities:
- Phytophagous insects utilize various sense organs, including:
- Visual: Insects often rely on visual cues such as color and shape to locate potential host plants.
- Olfactory: Chemical cues emitted by plants play a crucial role. Insects detect volatile organic compounds (VOCs) that indicate the presence of suitable food sources.
- Tactile: Once in proximity, insects may use tactile cues to assess the plant surface.
- Gustatory: Taste receptors allow insects to evaluate the chemical composition of plants upon contact.
- Phytophagous insects utilize various sense organs, including:
- Factors Influencing Host Selection:
- The availability and abundance of plant species significantly influence the host selection of phytophagous insects. If a previously used host plant is scarce, insects may shift to new hosts. Additionally, there is a tendency for insects to prefer plants where their mothers laid eggs, suggesting a learned preference for specific plant characteristics.
- Specific Examples of Host Selection:
- Orthoptera: Grasshoppers tend to favor grassy areas like lawns and fallow lands, choosing plants rich in sugars, amino acids, and vitamins. For instance, locusts primarily consume specific monocots, avoiding dicotyledons altogether.
- Diptera: Fruit flies are strongly influenced by color and odor when selecting fruits. The cabbage root fly, for example, uses wind direction to locate odors emanating from host plants.
- Lepidoptera: Caterpillars are drawn to the volatile compounds released by certain plants, such as cabbage. Female butterflies often choose oviposition sites based on their larvae’s feeding preferences, utilizing taste receptors on their legs to identify suitable plants.
- Coleoptera: Beetles from the Chrysomelidae family specifically seek out crucifers that are high in glucosinolates. Visual and olfactory cues are crucial for many Cerambycidae species in locating hosts.
- Hemiptera: Aphids demonstrate specific hovering behaviors that help them accumulate in favorable feeding locations. Additionally, certain species have a narrow host range but may exhibit a strong preference for plants within specific families, such as the Solanaceae.
- Chemical Interactions:
- The interaction between phytophagous insects and plants often involves a chemical dialogue. Plants produce various compounds that can influence insect behavior:
- Attractants: Volatile compounds can lure insects to plants for oviposition.
- Repellents: Alkaloids, terpenoids, and phenolics can deter herbivorous insects.
- Feeding or Oviposition Stimulants: Specific chemicals, like Aristolochic acid, can stimulate feeding or egg-laying behaviors.
- Deterrents: Certain compounds can cause insects to cease feeding altogether.
- The interaction between phytophagous insects and plants often involves a chemical dialogue. Plants produce various compounds that can influence insect behavior:
How does co-evolution affect the relationship between plants and other organisms?
Co-evolution is a dynamic process characterized by reciprocal evolutionary changes between interacting species, particularly plants and other organisms. This intricate relationship has led to the development of complex adaptations and interactions that enhance survival and reproductive success. The following points illustrate how co-evolution shapes these relationships:
- Defensive Adaptations in Plants:
- Plants have evolved a variety of physical and chemical defenses to deter herbivores. These defenses include structures such as thorns, spines, and stinging hairs, along with chemical compounds like phenolics and terpenoids. These adaptations serve to reduce herbivory by making plants less palatable or more toxic. In turn, herbivores have developed counter-adaptations, such as specialized mouthparts or detoxification mechanisms, allowing them to exploit these plants despite their defenses.
- Mutualistic Relationships:
- An exemplary case of co-evolution is observed in the relationship between acacia trees and acacia ants. The trees provide shelter and food sources, such as nectar and protein-rich Beltian bodies, for the ants. In return, the ants defend the trees from herbivorous insects and other threats. This mutualistic interaction enhances the survival of both species, illustrating how co-evolution can lead to interdependence.
- Pollinator Interactions:
- The co-evolution of flowering plants and their pollinators is another critical aspect of plant-animal interactions. Flowering plants have developed specific traits, such as vibrant colors, alluring scents, and nectar rewards, to attract pollinators. Meanwhile, pollinators have evolved specialized anatomical structures, such as elongated proboscises or unique mouthparts, enabling them to efficiently extract nectar and pollen. This co-adaptation benefits both groups, facilitating plant reproduction and ensuring food sources for pollinators.
- Allelochemical Influence:
- Allelochemicals are secondary metabolites produced by plants that can significantly influence the growth and development of other organisms. Certain plants, through allelopathy, release these compounds into the environment, which can inhibit the growth of competing plants by altering chlorophyll production and photosynthesis. Additionally, allelochemicals can modify plant growth regulators and induce hormonal imbalances, thereby affecting overall plant health and development. This chemical warfare exemplifies the evolutionary pressures exerted by plants on their competitors and herbivores.
- Evolutionary Arms Race:
- The ongoing interactions between plants and their herbivores can be described as an evolutionary arms race. As plants evolve new defenses, herbivores respond with counter-adaptations, creating a cycle of reciprocal adaptations. This dynamic promotes biodiversity and drives the evolution of specialized traits in both plants and herbivores.
- Environmental Context:
- Co-evolution is also influenced by environmental factors, such as climate, soil type, and the presence of other organisms. These factors can shape the specific adaptations that evolve within a given ecosystem. For instance, plants in nutrient-poor soils may develop more potent allelochemicals to outcompete neighbors, while those in resource-rich environments may focus on attracting pollinators.
What are the different types of coevolutionary relationships and their implications for ecosystems?
The following points outline these relationships and their ecological significance:
- Predator-Prey Relationships:
- This dynamic involves one organism, the predator, hunting and consuming another, the prey. The evolutionary interactions between these two groups often lead to an “arms race.” For example, predators may evolve enhanced sensory abilities, speed, or stealth, while prey species may develop effective defenses, such as camouflage, warning coloration, or physical adaptations like spines. This constant adaptation enhances biodiversity and contributes to the stability of ecosystems by promoting diverse predator and prey populations.
- Herbivore-Plant Interactions:
- Plants have developed a wide range of defensive mechanisms to deter herbivores from feeding on them. These defenses include physical barriers such as thorns, spines, and tough leaves, as well as chemical deterrents like toxins and allelochemicals. In response, herbivores have evolved specialized adaptations, such as powerful jaws, enzymes to detoxify plant compounds, or the ability to consume toxic plants. This interplay not only influences the evolutionary trajectories of both groups but also shapes plant community dynamics and affects nutrient cycling within ecosystems.
- Mutualistic Relationships:
- Coevolution also fosters mutualistic interactions, where both species benefit. A prominent example is the relationship between acacia trees and acacia ants. The trees provide food and shelter to the ants, while the ants protect the trees from herbivores and other threats. Such mutualistic relationships enhance ecosystem resilience, as they can stabilize populations and facilitate resource sharing among species.
- Pollinator-Flower Interactions:
- The co-evolution of flowering plants and their pollinators is crucial for plant reproduction and biodiversity. Flowers have adapted to attract specific pollinators through traits like vibrant colors, enticing scents, and nectar rewards. In turn, pollinators have evolved specialized features for accessing nectar and pollen. This relationship underscores the interconnectedness of species in ecosystems, as successful pollination is essential for the reproduction of many plant species, influencing food webs and habitat structure.
- Implications for Ecosystems:
- The ongoing interactions and adaptations between co-evolving species have profound implications for ecosystem health and stability. Predator-prey dynamics contribute to population control and species diversity, while herbivore-plant interactions can shape community composition and nutrient availability. Mutualistic relationships enhance resource efficiency and facilitate ecosystem resilience in the face of environmental changes. Furthermore, the interconnectedness established through coevolution can lead to greater ecosystem productivity and stability, as the success of one species often depends on the health and survival of others.
- Diversity and Ecosystem Function:
- Coevolutionary relationships contribute to biodiversity, which is a key component of healthy ecosystems. Diverse species interactions promote resilience against environmental disturbances, such as climate change and habitat loss. Therefore, understanding coevolutionary processes is essential for conservation efforts aimed at preserving biodiversity and maintaining functional ecosystems.
How do allelochemicals affect plant growth?
Allelochemicals are secondary metabolites synthesized by various organisms, including plants, algae, bacteria, and fungi. These compounds play a significant role in influencing the growth and development of other plants, often affecting competition, succession, and overall ecosystem dynamics. The following points illustrate the mechanisms by which allelochemicals can impact plant growth:
- Reduction of Chlorophyll Production:
- Certain allelochemicals can interfere with the synthesis of chlorophyll in target plants. A decrease in chlorophyll levels directly impairs the photosynthetic capacity of plants, leading to reduced energy production. This inhibition can slow growth rates or even lead to plant death, especially in species that are sensitive to these compounds. Therefore, the overall vigor of plant populations may be compromised in environments rich in allelochemicals.
- Disruption of Plant Growth Regulators:
- Allelochemicals can affect the levels of plant growth regulators, which are critical for various developmental processes. These substances can cause imbalances in phytohormones such as auxins, gibberellins, and cytokinins. For instance, alterations in auxin levels can disrupt cell elongation and division, adversely impacting root and shoot growth. This disruption can lead to stunted growth or abnormal development patterns in affected plants.
- Allelopathic Effects:
- Many plants produce allelochemicals as a strategy to compete with neighboring vegetation. These allelochemicals can inhibit seed germination, root development, and overall growth of competing plant species. For example, certain tree species release compounds that suppress the growth of understory plants, allowing them to monopolize resources like sunlight, water, and nutrients. This competitive advantage can alter community structure and species diversity in ecosystems.
- Induction of Physiological Stress:
- Exposure to allelochemicals can induce physiological stress in sensitive plants, leading to altered metabolic pathways. This stress response may result in reduced nutrient uptake, impaired water regulation, and enhanced susceptibility to pathogens. Consequently, plants affected by allelochemicals may exhibit signs of stress, such as wilting, leaf chlorosis, and reduced reproductive success.
- Impact on Root Development:
- Some allelochemicals can negatively influence root morphology and function, which are essential for nutrient and water absorption. Inhibition of root growth can lead to decreased plant resilience against environmental stressors, such as drought or nutrient deficiency. Furthermore, altered root architecture can limit a plant’s ability to compete effectively for resources.
- Ecological Implications:
- The effects of allelochemicals extend beyond individual plant health to influence entire ecosystems. By shaping plant community dynamics, allelochemicals can affect biodiversity, nutrient cycling, and habitat stability. For instance, allelopathic species may dominate certain environments, leading to decreased species richness and altered food web interactions.
How do endosymbionts contribute to insect survival and adaptation?
The following points outline how endosymbionts facilitate insect resilience and adaptability in various ecological contexts.
- Nutritional Support: Endosymbionts are instrumental in providing essential nutrients that may be lacking in the insect’s diet. For instance, certain endosymbiotic bacteria synthesize crucial amino acids, vitamins, and other micronutrients that are vital for the growth and development of their hosts. This relationship is especially beneficial for insects, such as sap-sucking or leaf-chewing species, that often consume nutrient-poor diets.
- Detoxification of Plant Defenses: Many plants produce secondary metabolites—chemical compounds that can be toxic to herbivores. Endosymbionts enable insects to detoxify these harmful substances, allowing them to exploit plant species that would otherwise be detrimental to their health. This detoxification capability significantly broadens the range of host plants that insects can utilize, thus enhancing their dietary options and ecological niche.
- Enhanced Digestion: Endosymbionts improve the digestive efficiency of insects by aiding in the breakdown of complex plant materials that the insects themselves cannot digest. For example, certain bacteria can assist in the fermentation of cellulose, a primary component of plant cell walls. This symbiotic relationship allows insects to extract additional nutrients from fibrous food sources, promoting better growth and reproductive success.
- Adaptation to Host Plants: Specific endosymbionts can facilitate an insect’s adaptation to particular host plants, especially those with complex chemical defenses. This adaptability fosters co-evolution, where both the insect and the plant evolve traits that enhance their mutual survival. Such evolutionary dynamics can lead to specialization, where insects develop unique adaptations to effectively exploit their host plants.
- Influence on Insect Behavior: Endosymbionts can also modify the behavior of their insect hosts, influencing feeding preferences, reproductive strategies, and interactions with other organisms. For example, certain endosymbionts may manipulate the host’s attraction to specific plant compounds, thus shaping its ecological interactions and niche. These behavioral changes can have significant ramifications for the insect’s survival and reproductive success in its environment.
What role do secondary metabolites play in insect-plant interactions?
Secondary metabolites are organic compounds synthesized by plants that play crucial roles beyond basic growth, development, and reproduction. These compounds significantly influence insect-plant interactions, impacting both plant defense mechanisms and insect behavior. The following points delineate the various roles that secondary metabolites fulfill in these complex ecological relationships.
- Defense Against Herbivory: Many secondary metabolites act as chemical deterrents to herbivorous insects. These compounds, which include alkaloids, tannins, and phenolics, can be toxic or repellent. They may also reduce the digestibility of plant tissues, making them less palatable to herbivores. Consequently, these metabolites protect plants from damage and help maintain their health and reproductive capacity.
- Attraction of Natural Enemies: In response to insect herbivory, some plants emit volatile secondary metabolites that serve to attract natural enemies of the herbivores, such as predators and parasitoids. This response, termed “induced plant defense,” enhances the plant’s indirect defenses by enlisting external agents to combat herbivore populations. Therefore, these compounds not only protect the plant directly but also foster a broader ecological network of interactions.
- Insect Behavior Modification: Secondary metabolites can significantly influence insect behavior, acting either as feeding stimulants or deterrents. For instance, certain compounds may lure insects to the plant for feeding or oviposition, while others may repel them. This dual functionality allows plants to manage their interactions with various insect species, thus influencing the overall dynamics of the ecosystem.
- Sequestration for Defense: Some herbivorous insects have evolved the ability to sequester plant secondary metabolites for their own defensive purposes. By incorporating these toxic compounds into their bodies, insects can deter predators and parasitoids. This strategy enables them to exploit plants that produce harmful substances while simultaneously using those toxins for their protection, illustrating a sophisticated adaptation to ecological pressures.
- Communication: Secondary metabolites also facilitate chemical communication between plants and insects. Specific compounds can signal to insects that a plant is a suitable host for feeding or egg-laying. Conversely, certain metabolites may indicate that the plant is under stress or has been damaged. This signaling is crucial for both plants and insects, as it informs behavioral decisions and interactions.
- Impact on Plant Quality: The presence of secondary metabolites can alter the nutritional quality of plants, which directly affects the fitness of herbivorous insects. For example, high concentrations of certain metabolites may make a plant less nutritious, leading to decreased insect growth and reproductive success. Furthermore, beneficial microbes associated with plants can influence the production of these metabolites, thereby impacting insect behavior and overall fitness.
How do plants defend themselves against insect herbivores?
Plants have developed an array of defense mechanisms to safeguard themselves from insect herbivores, employing a combination of physical, chemical, and behavioral strategies. These adaptations are crucial for enhancing plant survival and maintaining ecosystem balance. The following points outline the primary defense mechanisms utilized by plants:
- Physical Defenses:
- Morphological Traits: Many plants exhibit structural features that hinder herbivore feeding. For instance, thorns and spines act as physical barriers, making it difficult for insects to access the plant tissues. Additionally, trichomes, or hair-like structures on leaves, can obstruct feeding and may contain irritating substances that deter herbivores.
- Phenological Escape: Some plants strategically time their growth and reproductive cycles to coincide with periods of low herbivore activity. By synchronizing their life stages with environmental cues, they minimize exposure to potential insect attacks, effectively evading herbivory.
- Chemical Defenses:
- Secondary Metabolites: Plants produce a diverse array of secondary metabolites that play a vital role in deterring herbivory. These compounds, which include alkaloids, tannins, phenolics, and terpenoids, can be toxic or repellent to herbivores. For example, alkaloids can interfere with the nervous systems of insects, while tannins bind to proteins, reducing their digestibility and nutritional value.
- Induced Defenses: In response to herbivore damage, many plants can enhance the production of defensive compounds through a process known as induced defense. This dynamic response allows plants to adaptively increase their resistance when faced with herbivore threats, thereby improving their chances of survival.
- Attraction of Natural Enemies:
- Volatile Organic Compounds (VOCs): When attacked, some plants release specific volatile compounds that attract natural enemies of herbivores, such as predators and parasitoids. This indirect defense mechanism facilitates the reduction of herbivore populations by inviting organisms that prey on them, thus creating a biological control system.
- Nutritional Quality Alteration:
- Plants can adjust their nutritional profile in response to herbivory, rendering their tissues less appealing or nutritious to insects. This alteration may involve modifications in essential nutrient levels or the production of compounds that impede digestion, further deterring herbivore feeding.
- Mutualistic Relationships:
- Some plants form mutualistic partnerships with other organisms, such as ants, which provide protection against herbivores in exchange for food resources like nectar or extrafloral nectaries. These mutualistic relationships enhance the plant’s defensive capabilities and contribute to its overall fitness.
- Chemical Communication:
- Plants can communicate with each other through root exudates or airborne signals, warning neighboring plants of herbivore presence. This signaling can trigger defensive responses in nearby plants, increasing their resilience to potential attacks and fostering a community-wide defensive strategy.
What are tri-trophic interactions, and how do they influence insect-plant dynamics?
Tri-trophic interactions represent a fundamental ecological framework involving three distinct trophic levels: plants (producers), herbivores (primary consumers), and their natural enemies, such as predators and parasitoids (secondary consumers). These interactions play a crucial role in shaping the dynamics of insect-plant relationships and can significantly influence broader ecological outcomes. The following points elucidate the mechanisms of tri-trophic interactions and their effects on insect-plant dynamics:
- Components of Tri-Trophic Interactions:
- Plants: Function as primary producers, offering food and habitat for herbivores. They often develop a range of defense mechanisms—both physical (e.g., thorns, tough leaves) and chemical (e.g., secondary metabolites)—to deter herbivory.
- Herbivores: As primary consumers, these organisms feed on plants. Their feeding activities can induce physiological and chemical changes in plants, often prompting the production of defensive compounds that further influence plant-herbivore dynamics.
- Natural Enemies: Comprising predators and parasitoids, these organisms prey on herbivores. They play a vital role in regulating herbivore populations, thereby affecting plant health and productivity.
- Influence on Plant Defense Mechanisms:
- The presence of herbivores can stimulate plants to bolster their defenses. This includes increasing the production of secondary metabolites and volatile organic compounds (VOCs) that attract natural enemies. Such induced defenses create a feedback loop, where the plant’s response to herbivory not only affects herbivore populations but also enhances the effectiveness of their natural enemies.
- Impact on Herbivore Behavior and Fitness:
- Tri-trophic interactions can significantly alter the behavior and fitness of herbivores. For instance, the presence of natural enemies may prompt herbivores to change their feeding habits, opting for less palatable plants or altering their foraging strategies to avoid predation. These behavioral changes can have direct implications on herbivore growth, reproduction, and overall survival rates.
- Ecological Balance:
- The dynamics of tri-trophic interactions contribute to ecosystem stability and balance. By regulating herbivore populations, natural enemies prevent overgrazing, which in turn promotes plant diversity. This balance is critical for sustaining healthy ecosystems and can influence agricultural practices, underscoring the interconnectedness of these interactions.
- Evolutionary Implications:
- Tri-trophic interactions are also pivotal in driving co-evolutionary processes among plants, herbivores, and their natural enemies. For example, as plants evolve new defensive strategies, herbivores may develop counter-adaptations, resulting in an ongoing evolutionary arms race. Similarly, natural enemies may evolve more effective strategies to overcome plant defenses or to enhance their ability to locate herbivores.
- Influence of Environmental Factors:
- Environmental conditions, such as nutrient availability, climate variables, and habitat diversity, can significantly influence tri-trophic interactions. For example, increased nitrogen levels in the soil can enhance plant secondary metabolite production, subsequently affecting herbivore feeding behaviors and the effectiveness of natural enemies in controlling herbivore populations.
How do environmental factors affect insect-plant interactions?
Environmental factors significantly influence insect-plant interactions, affecting the behavior, physiology, and ecology of both groups. These interactions are complex and are shaped by various environmental conditions. Below are the key ways in which environmental factors impact these interactions:
- Nutrient Availability:
- Nutrient levels, particularly nitrogen and phosphorus, play a crucial role in determining plant growth and the synthesis of secondary metabolites. Increased nitrogen availability can lead to a decrease in carbon-based secondary metabolites in plants, potentially making them more palatable to herbivores. This change can subsequently influence herbivore feeding behavior and population dynamics, resulting in shifts in herbivore abundance and diversity.
- Climate Conditions:
- Temperature and humidity are essential environmental parameters that impact the physiological processes of plants and insects. Elevated temperatures can accelerate insect development and reproduction rates, potentially resulting in increased herbivory. Conversely, extreme temperatures can induce stress in plants, making them more vulnerable to insect attacks. Climate change can also affect the phenology of both plants and insects, leading to mismatches in the timing of flowering and herbivore activity, which disrupts established ecological interactions.
- Water Availability:
- Drought conditions can impose significant stress on plants, leading to alterations in their chemical defenses. Stressed plants may enhance the production of certain secondary metabolites as a defensive response, which can deter herbivores. However, prolonged drought can diminish plant vigor, increasing susceptibility to herbivory. Thus, the balance between water availability and plant health is critical in determining the dynamics of insect-plant interactions.
- Light Intensity:
- Light availability directly affects plant growth and photosynthesis, which in turn influences the nutritional quality of plant tissues. Plants growing under low light conditions may exhibit reduced levels of secondary metabolites, potentially increasing their attractiveness to herbivores. Additionally, varying light conditions can influence the visibility of plants to herbivores and their natural enemies, thereby affecting feeding patterns and predator-prey dynamics.
- Soil Composition and Structure:
- The physical and chemical properties of soil significantly influence plant health and growth, impacting their interactions with herbivores. For instance, soil compaction can restrict root growth and limit nutrient uptake, leading to weakened plants that are more susceptible to insect attacks. Healthy soil conditions promote robust plant growth, enhancing the plants’ ability to defend against herbivory.
- Biotic Interactions:
- The presence of other organisms, including competing plants, beneficial microbes, and predators, can substantially influence insect-plant dynamics. For example, plants competing for resources may invest more energy in growth rather than defense, making them more vulnerable to herbivores. Conversely, beneficial microbes can bolster plant health and enhance resistance to herbivory, creating a complex network of interactions that shapes community dynamics.
- Pollution and Chemical Exposure:
- Environmental pollutants, such as pesticides and heavy metals, can detrimentally impact both plant health and insect behavior. Pesticides may adversely affect beneficial insect populations or disrupt their natural enemies, potentially leading to increased herbivore populations. Furthermore, chemical exposure can alter the production of secondary metabolites in plants, affecting their palatability and defense mechanisms against herbivores.
- Habitat Structure:
- The physical structure of the habitat, encompassing plant density and diversity, influences insect movement and feeding behavior. Dense vegetation can provide refuge for herbivores from their natural enemies, allowing for increased herbivory. In contrast, diverse plant communities can support a broader range of insect species and interactions, promoting ecological resilience and stability.
What are the implications of insect-plant interactions for agricultural practices?
Here are the key implications of insect-plant interactions for agriculture:
- Pest Management Strategies:
- Knowledge of insect-plant dynamics aids in developing effective pest management practices. By recognizing the role of natural enemies, such as predators and parasitoids, farmers can implement integrated pest management (IPM) approaches. These strategies enhance beneficial organisms, reducing reliance on chemical pesticides and promoting ecological balance.
- Crop Resistance Breeding:
- Insights into plant defenses against herbivores can inform breeding programs aimed at developing pest-resistant crop varieties. Breeders can select for traits that enhance plant resilience, such as increased production of secondary metabolites or physical defenses (e.g., trichomes). These resistant crops can diminish susceptibility to insect damage, thereby improving yield and minimizing the need for chemical interventions.
- Use of Trap Crops:
- The implementation of trap cropping involves planting specific crops designed to attract pests away from economically valuable crops. By understanding herbivore preferences for certain plants, farmers can effectively reduce pest populations and minimize damage to primary crops, optimizing overall agricultural output.
- Enhancing Biodiversity:
- Promoting biodiversity within agricultural systems can lead to improved insect-plant interactions. Diverse cropping systems support a wider array of beneficial insects, which contribute to natural pest control. Additionally, maintaining habitat diversity around fields provides refuge for natural enemies and pollinators, further enhancing ecosystem services.
- Soil Health and Nutrient Management:
- The link between soil health and plant vigor is critical for managing insect-plant interactions. Practices that improve soil health, such as crop rotation, cover cropping, and the use of organic amendments, can enhance plant resilience to insect herbivory. Healthy plants are typically better equipped to produce defensive compounds that deter pests, thus reducing damage.
- Impact of Climate Change:
- Climate change presents challenges that alter insect-plant interactions, affecting pest dynamics and crop yields. Farmers must adapt their practices to accommodate shifts in pest populations and plant responses to environmental stressors. This adaptation may include adjusting planting dates, selecting more resilient crop varieties, and employing adaptive management strategies.
- Chemical Use and Resistance Management:
- The application of chemical pesticides can disrupt insect-plant interactions by harming beneficial insects and fostering pest resistance. A deep understanding of ecological dynamics allows farmers to make informed decisions regarding pesticide use, promoting practices that minimize negative impacts on beneficial organisms and maintaining ecological integrity.
- Pollination Services:
- Insect-plant interactions are crucial for effective pollination, which is essential for many crop species. Understanding relationships between plants and their pollinators enables farmers to enhance pollination services. Practices such as planting pollinator-friendly crops and providing suitable habitats for pollinators can significantly improve crop yields.
- Economic Implications:
- Effective management of insect-plant interactions can lead to increased crop yields and reduced losses from pest damage, enhancing the economic viability of agricultural operations. By investing in sustainable practices that foster beneficial interactions, farmers can achieve long-term productivity and profitability, ensuring the sustainability of their farming practices.
How do insects use plant secondary metabolites for their own defense mechanisms?
The following points outline the primary methods by which insects utilize these plant-derived chemicals:
- Sequestration:
- Herbivorous insects often sequester plant secondary metabolites within their bodies during feeding. This process allows insects to store toxic or unpalatable compounds, which they can later use as chemical defenses against their own predators. For instance, certain caterpillars can accumulate alkaloids from their host plants, rendering them distasteful or harmful to potential predators.
- Chemical Mimicry:
- Insects may mimic the chemical profiles of their host plants by incorporating secondary metabolites into their own biochemistry. By resembling the chemical signatures of unpalatable or toxic plants, they can avoid detection by predators that are conditioned to avoid such compounds. This form of chemical camouflage provides an effective means of protection.
- Induction of Plant Defenses:
- Some insects have the ability to induce the production of secondary metabolites in plants as a defensive response to their feeding activities. This induction can enhance the plant’s defenses, making it less palatable or more toxic to other herbivores. Consequently, the insect benefits from a reduced competition and predation risk, as the plant’s heightened defenses deter additional herbivores.
- Use of Plant Compounds in Communication:
- Insects can utilize plant secondary metabolites for chemical communication. Certain herbivorous insects produce pheromones derived from plant compounds, which can serve to attract mates or signal alarm to conspecifics. This chemical communication facilitates reproductive success and enhances survival by alerting others to potential threats.
- Defense Against Parasitoids:
- Insects that sequester toxic compounds from plants can employ these metabolites to defend against parasitoids. By integrating these toxins into their bodies, they can deter parasitoids from laying eggs or feeding on them. This adaptation significantly increases their chances of survival, as it creates a hostile environment for their natural enemies.
- Enhancing Fitness:
- The ability to utilize plant secondary metabolites can substantially enhance the fitness of herbivorous insects. Insects that can effectively sequester and employ these defensive compounds often experience reduced predation rates, leading to improved survival and reproductive outcomes. This adaptability is crucial in the dynamic environments where these insects thrive.
- Symbiotic Relationships:
- Some insects form symbiotic relationships with microbes that assist them in detoxifying or utilizing plant secondary metabolites. These symbiotic microbes can augment the insect’s capacity to feed on toxic plants, while also contributing to the insect’s chemical defenses. Such relationships exemplify the intricate interactions between insects, plants, and microorganisms, further highlighting the complexity of ecological relationships.
How do insects select their host plants, and what factors influence this choice?
Below are the primary factors that shape host plant selection by insects:
- Chemical Cues:
- Insects are highly attuned to the chemical signals emitted by plants, including volatile organic compounds (VOCs) and various secondary metabolites. These chemical cues serve as indicators of the plant’s nutritional quality, palatability, and defense mechanisms. For instance, certain herbivores may be drawn to plants that release specific VOCs when damaged, signaling their suitability for feeding.
- Visual Cues:
- The visual attributes of plants, such as color, shape, and size, significantly influence host plant selection. Insects may utilize these visual signals to identify potential hosts, particularly in environments where chemical cues are less perceptible. Brightly colored flowers often attract pollinators, while distinctive leaf shapes may indicate appropriate foliage for herbivory.
- Nutritional Quality:
- The nutritional composition of potential host plants is critical for insect herbivores. Factors such as carbohydrate, protein, and essential amino acid levels determine the attractiveness of a plant. Insects tend to favor plants rich in nitrogen, which is vital for their growth and reproductive success.
- Plant Secondary Metabolites:
- While many secondary metabolites serve as deterrents to herbivory due to their toxic or unpalatable nature, others function as phagostimulants, promoting feeding. Insects may preferentially select plants containing specific secondary metabolites that enhance their own defensive capabilities or overall fitness.
- Experience and Learning:
- Insects possess the capacity to learn from their past interactions with host plants. Positive experiences, such as successful feeding or reproduction on a particular plant, increase the likelihood of revisiting that plant. Conversely, negative experiences, such as encountering predators or experiencing toxicity, can lead to a marked avoidance of certain species.
- Microclimatic Conditions:
- The microclimate surrounding a plant plays a significant role in host plant selection. Factors such as light availability, humidity, and temperature can influence insect preferences. For example, shaded environments may be more appealing to certain herbivores during warm weather, offering relief from heat.
- Presence of Natural Enemies:
- The risk posed by predators or parasitoids can affect the selection of host plants. Insects may opt to avoid plants that harbor high populations of natural enemies, seeking instead those that provide a safer environment for feeding and reproduction.
- Plant Phenology:
- The developmental stage of a plant—whether it is flowering, fruiting, or producing leaves—can significantly affect its attractiveness to insects. Many herbivores may prefer young, tender leaves rich in nutrients, while others may target flowers or fruits for feeding or oviposition.
- Genetic Factors:
- Genetic diversity within insect populations can lead to variations in host plant preferences. Some individuals may exhibit innate preferences for specific plant species or varieties, which can influence the dynamics of insect-plant interactions at a broader ecological scale.
What are the challenges faced by phytophagous insects in detoxifying plant defenses?
The following points outline the major hurdles these insects face in their quest for survival and adaptation:
- Chemical Diversity:
- Plants produce an extensive array of secondary metabolites, including alkaloids, terpenoids, phenolics, and glucosinolates. Each of these compounds exhibits distinct chemical structures and mechanisms of action. Consequently, the vast diversity presents a formidable challenge for insects, necessitating the evolution or acquisition of specific detoxification mechanisms tailored to each compound.
- Metabolic Costs:
- The detoxification processes required to neutralize these toxic compounds are energetically demanding. The metabolic pathways involved in modifying or breaking down these toxins consume considerable resources, diverting energy from essential functions such as growth and reproduction. This trade-off can adversely affect the overall fitness of the insect.
- Evolutionary Lag:
- An evolutionary lag may exist between the emergence of new plant defenses and the corresponding adaptations in insect populations. As plants evolve novel chemical defenses, insects may struggle to adapt in a timely manner, resulting in heightened mortality rates or decreased reproductive success among those populations unable to efficiently detoxify these compounds.
- Symbiotic Relationships:
- Some phytophagous insects rely on symbiotic microorganisms to assist in detoxifying plant defenses. However, the effectiveness of these symbionts can vary considerably based on environmental conditions, host plant quality, and the insect’s own physiological state. If these beneficial microbes are ineffective, the insects may face greater toxicity from the plant defenses.
- Resistance Development:
- While some insects can develop resistance to specific plant defenses over time, this process can be slow and inconsistent across populations. Moreover, the mechanisms underlying resistance may incur trade-offs, such as reduced fitness when feeding on non-toxic plants or increased vulnerability to alternative stressors.
- Environmental Variability:
- Fluctuations in environmental conditions—such as temperature, humidity, and the availability of host plants—can significantly influence the effectiveness of detoxification mechanisms. Stressful conditions may impair an insect’s ability to metabolize toxins efficiently, resulting in heightened susceptibility to the defensive compounds of their host plants.
- Behavioral Constraints:
- Insects must often balance their feeding strategies with the necessity of avoiding toxic compounds. This constraint can limit their ability to exploit certain host plants, particularly if their detoxification capabilities are insufficient. While behavioral adaptations, such as targeting less toxic plant parts or feeding during specific times, may mitigate some risks, these strategies may not always be feasible.
- Impact of Coevolution:
- The coevolutionary dynamics between plants and herbivores result in increasingly sophisticated plant defenses. As plants evolve more potent toxins, the corresponding need for insects to refine their detoxification strategies intensifies, presenting a substantial challenge—especially for specialized feeders that depend on a limited range of host plants.
- Genetic Constraints:
- The genetic foundation of detoxification mechanisms can be intricate, and not all insect populations possess the requisite genetic variability to adapt to novel or intensified plant defenses. Limited genetic diversity within populations can impede their capacity to evolve effective detoxification strategies.
How do insects communicate with plants, and what role do volatile compounds play?
The following points elucidate how this communication transpires and the critical role of volatile compounds:
- Induced Volatile Production:
- Upon being attacked by herbivores, plants often initiate a response characterized by the production and release of specific VOCs. This phenomenon, known as “induced volatile production,” serves multiple functions, including attracting natural enemies of the herbivores and signaling neighboring plants about the impending threat.
- Attraction of Natural Enemies:
- The VOCs emitted by damaged plants play a vital role in attracting natural enemies such as predatory insects and parasitoids. For instance, when a plant experiences infestation by caterpillars, it may release particular blends of VOCs that signal the herbivore’s presence, thereby prompting predators to locate and target the caterpillars. This attraction is a crucial component of the plant’s defensive strategy.
- Insect Responses to Plant Volatiles:
- Insects possess specialized sensory structures that enable them to detect and respond to plant volatiles effectively. These sensory cues guide insects toward suitable host plants for feeding and oviposition (egg-laying). The ability to perceive specific VOCs allows insects to select plants that are more nutritious or less defended, thereby optimizing their foraging efficiency.
- Chemical Communication:
- The interplay between plants and insects exemplifies a form of chemical communication. In response to plant signals, herbivorous insects may produce their own volatile compounds, which can further modulate plant responses. For example, certain herbivorous insects emit specific VOCs that can alter plant defenses or even stimulate the production of additional defensive compounds within the plant.
- Role in Plant Defense:
- The production of VOCs constitutes an integral part of a plant’s defense mechanism. Through the emission of these compounds, plants not only attract beneficial insects but also deter further herbivore attacks. Some VOCs act as repellents or signal neighboring plants to prepare their defenses against potential herbivory.
- Inter-plant Communication:
- VOCs facilitate communication between neighboring plants as well. When one plant is under attack, the emitted volatiles can be detected by nearby plants, prompting them to activate their own defense mechanisms in anticipation of herbivore attacks. This phenomenon, known as “priming,” enhances the resilience of plant communities against herbivore pressure.
- Environmental Influences:
- The production and release of VOCs can be significantly influenced by various environmental factors, including light, temperature, and humidity. These factors can affect both the quantity and quality of the volatiles produced, ultimately influencing the effectiveness of communication between plants and insects.
What are the primary allelochemicals involved in plant-insect interactions?
The primary allelochemicals involved in these interactions can be categorized as follows:
- Terpenoids:
- Terpenoids, also known as isoprenoids, play a pivotal role in plant defense mechanisms. These compounds can deter herbivores through their toxicity or unpleasant taste. Additionally, terpenoids may attract natural enemies of herbivores, such as predatory insects and parasitoids. This dual function underscores their importance in both direct and indirect plant defense strategies.
- Phenolics:
- Phenolic compounds are another class of allelochemicals that contribute to plant defense. They can reduce herbivory by rendering plants less palatable, often through astringent properties. Some phenolics possess toxic effects on insects, disrupting their feeding and potentially hindering their development. The presence of these compounds often correlates with increased resistance to herbivore attack.
- Alkaloids:
- Alkaloids are well-known for their potent toxicity and serve as effective deterrents against a variety of herbivores. These nitrogen-containing compounds can interfere with the nervous system of insects, thus affecting their feeding behavior and developmental processes. Many plants produce alkaloids as a primary line of defense, showcasing a remarkable evolutionary adaptation to herbivory.
- Glucosinolates:
- Glucosinolates are sulfur-containing compounds found predominantly in cruciferous plants, such as cabbage and broccoli. These metabolites can deter herbivores due to their bitter taste and potential toxicity. Moreover, glucosinolates may attract parasitoids that specifically target the herbivores feeding on the host plant, facilitating an indirect defensive strategy for the plant.
- Fatty Acid Derivatives:
- Fatty acid derivatives, including jasmonic acid and other oxylipins, can function as both feeding stimulants and deterrents. Depending on the context and concentration, these compounds can influence insect behavior, prompting feeding or deterring herbivory. This variability highlights the dynamic nature of plant-insect interactions.
- Volatile Organic Compounds (VOCs):
- VOCs are volatile substances emitted by plants in response to herbivore damage. These compounds play a crucial role in indirect plant defense by attracting natural enemies of herbivores, such as parasitoids and predatory insects. The release of specific VOCs can enhance the recruitment of these beneficial organisms, effectively mitigating herbivore pressure on the plant.
How do specific phytochemicals influence insect behavior, such as feeding and oviposition?
The following points outline how these interactions unfold:
- Feeding Deterrents:
- Certain phytochemicals, such as alkaloids and phenolics, function as feeding deterrents. These compounds make the plant less palatable or even toxic. For instance, tannins can bind to proteins in the digestive systems of herbivores, reducing the digestibility of plant material and subsequently making the plant less attractive for feeding. This mechanism can effectively limit herbivory and enhance plant survival.
- Feeding Stimulants:
- Conversely, specific phytochemicals can act as feeding stimulants, enticing insects to feed on certain plants. Compounds like particular sugars or amino acids may be present in plant tissues and can attract herbivores. The presence of these attractive compounds can increase the likelihood of herbivorous insects choosing to feed on the plants containing them, thereby affecting their foraging behavior.
- Oviposition Cues:
- Phytochemicals also serve as critical cues for oviposition, guiding female insects in selecting suitable host plants for laying eggs. Chemical signals, such as certain flavonoids or other secondary metabolites, can indicate to female moths that a plant is an appropriate host for their larvae. This chemical recognition is vital for ensuring the survival of the next generation by selecting optimal environments for offspring development.
- Chemical Signaling:
- The presence of specific phytochemicals can provide information about the nutritional quality of a plant. Insects have evolved mechanisms to detect these chemical cues, which include both attractants and deterrents. By recognizing these signals, insects can make informed decisions regarding their feeding and oviposition behaviors, optimizing their chances for survival and reproductive success.
- Induced Responses:
- When plants experience damage from herbivores, they may emit herbivore-induced plant volatiles (HIPVs). These volatile compounds can attract natural enemies of the herbivores, such as predators and parasitoids. This indirect defense mechanism not only influences the behavior of herbivores but also impacts the foraging behavior of their predators, creating a complex interaction network within the ecosystem.
What role do volatile organic compounds play in attracting or repelling insects?
Volatile organic compounds (VOCs) serve as critical chemical signals that influence insect behavior, particularly in terms of attraction and repulsion. These compounds play multifaceted roles in ecological interactions, shaping the dynamics between plants and insects. The following points elucidate the functions of VOCs in these contexts:
- Attraction to Pollinators:
- Many flowering plants emit specific VOCs that signal the presence of nectar and pollen, thereby attracting pollinators such as bees, butterflies, and moths. Common compounds include terpenoids and benzenoids, known for their floral scents. For example, linalool and benzaldehyde are recognized for their effectiveness in drawing various pollinators, which is essential for plant reproduction and ecological balance.
- Host Plant Recognition:
- Herbivorous insects rely on VOCs to locate appropriate host plants for feeding and oviposition. The unique blend of VOCs emitted by a plant can indicate its nutritional quality and suitability as a food source. Insects have adapted to identify these chemical cues, which may include both attractive and deterrent signals, guiding their foraging behavior and reproductive choices.
- Repellents:
- In contrast to attractants, some VOCs function as repellents, deterring insects from approaching or feeding on certain plants. For instance, specific plants may release VOCs that signal to herbivores their unpalatability or potential toxicity. This defense mechanism reduces the likelihood of herbivory, thereby enhancing plant survival and fitness.
- Induced Plant Responses:
- When herbivores damage plants, these plants may respond by emitting herbivore-induced plant volatiles (HIPVs). These compounds can attract natural enemies of the herbivores, such as parasitoids and predators. This indirect defense strategy not only protects the plant by increasing predation on the herbivores but also plays a crucial role in shaping the interactions within the food web.
- Chemical Communication:
- VOCs facilitate intricate chemical communication between plants and insects, allowing for a range of interactions. The similarity in volatile chemistry between flowers and certain insects suggests an evolutionary adaptation where flowers may produce insect semiochemicals to manipulate insect behavior. This relationship enhances the likelihood of successful pollination, thereby benefiting both plants and pollinators.
How do environmental factors influence the production of allelochemicals in plants?
Environmental factors play a crucial role in influencing the production of allelochemicals in plants, which are secondary metabolites that can affect the interactions between plants and their herbivores or neighboring plants. The following points outline how various environmental conditions impact the synthesis and release of these important compounds:
- Light Availability:
- The quantity and quality of light directly influence photosynthesis, which in turn affects the synthesis of secondary metabolites, including allelochemicals. For instance, plants exposed to higher light intensities are likely to produce increased levels of flavonoids and terpenoids. These compounds serve multiple functions, such as deterring herbivores or attracting pollinators, thereby enhancing the plant’s reproductive success.
- Soil Nutrients:
- The availability of essential nutrients, such as nitrogen and phosphorus, significantly affects the production of allelochemicals. Nutrient-rich conditions can promote plant growth and enhance their capacity to produce secondary metabolites. Conversely, nutrient-poor environments may trigger an increase in allelochemical production as a defensive strategy against herbivory, allowing plants to allocate resources toward chemical defenses.
- Water Availability:
- Water stress is a significant factor influencing allelochemical production. Drought conditions often stimulate the synthesis of specific secondary metabolites, which can help plants cope with water scarcity and deter herbivores. For example, phenolic compounds may be produced in greater quantities under water-limited conditions, providing a defensive mechanism against feeding.
- Temperature:
- Temperature fluctuations can impact metabolic rates in plants, subsequently influencing the synthesis of allelochemicals. Elevated temperatures may enhance the production of certain volatile compounds, while extreme temperatures can induce stress responses that alter chemical defenses. The balance of temperature is crucial for optimal metabolic functioning and allelochemical production.
- Herbivory Pressure:
- The presence of herbivores acts as a significant stimulus for the production of allelochemicals. When plants experience damage from feeding, they may respond by releasing specific compounds designed to deter further herbivory or to attract natural enemies of the herbivores. This inducible response is a critical aspect of plant defense mechanisms.
- Microbial Interactions:
- Interactions with soil microorganisms and mycorrhizal fungi can also influence allelochemical production. Beneficial microbes enhance nutrient uptake and improve plant health, which can lead to increased production of secondary metabolites. This relationship underscores the interconnectedness of plant health, microbial communities, and allelochemical dynamics.
- Environmental Stressors:
- Additional stressors, such as pollution, salinity, and inter-plant competition, can significantly affect allelochemical production. Under stress, plants may increase their synthesis of allelochemicals to better compete for resources or defend against herbivores. These responses are crucial for maintaining plant fitness in challenging environmental conditions.
- Al Tawaha, Abdel Rahman & Sharma, Anamika & Banerjee, Dhriti & Sengupta, Jayita. (2021). Insect–Plant Interactions. 10.1201/9781003108931-11-11.
- https://www.scielo.br/j/bjpp/a/sKjpnLPZp7Qc8ypvrmyMDtm/?format=pdf&lang=en
- https://entnemdept.ufl.edu/baldwin/webbugs/3005_5006/lecture%20units/Unit%2010%20Module/notes10.pdf
- https://www.slideshare.net/slideshow/phytophagous-insect-and-their-host-finding-mechanism/214117057
- https://palivec.entu.cas.cz/~cizek/EkologieLesaPrednaska/InsectPlantInteractions/InsectPlantBiology.pdf
- https://chatham.ces.ncsu.edu/wp-content/uploads/2020/10/Plant-Insect-Interactions-Part-2.pdf?fwd=no
- https://www.ugr.es/~jmgreyes/PRESENTACIONES_PPT/13Coevolucion.pdf
- https://www.slideshare.net/slideshow/theory-of-coevolutionpptx/255350194
- https://www.slideshare.net/matufrank/plant-insects-interactions
- https://evolution.unibas.ch/teaching/evol_fort/pdf/Coevolution.pdf
- https://entnemdept.ufl.edu/baldwin/webbugs/3005_5006/Docs/notes/notes10.pdf
- https://cales.arizona.edu/classes/ento415/LECTURES/ENTO415_PlantInteractions.pdf