What is Inbreeding depression?
- Inbreeding depression refers to the decline in biological fitness and health that occurs due to the mating of individuals who are closely related by descent. This phenomenon primarily arises from the increase in homozygosity in the progeny, which results from the mating of genetically similar individuals. Inbreeding elevates the probability that offspring will inherit deleterious recessive alleles from both parents, leading to a higher incidence of genetic disorders and reduced overall fitness.
- The process of inbreeding increases homozygosity, which means that the genetic variation within the population decreases. For instance, self-fertilization (selfing), a form of extreme inbreeding, reduces heterozygosity progressively in each generation. Consequently, as homozygosity increases, the negative effects on vitality and reproductive success become more pronounced.
- Inbreeding depression manifests as reduced vigor and fertility among the progeny. This decline in fitness can be observed in various forms, such as decreased growth rates, higher susceptibility to diseases, and lower reproductive success. Therefore, the primary consequence of inbreeding is the erosion of genetic diversity, which undermines the adaptive potential of the population and can lead to long-term evolutionary disadvantages.
Definition of Inbreeding depression
Inbreeding depression is the reduced biological fitness and health of offspring resulting from the mating of closely related individuals, which increases homozygosity and exposes deleterious recessive alleles.
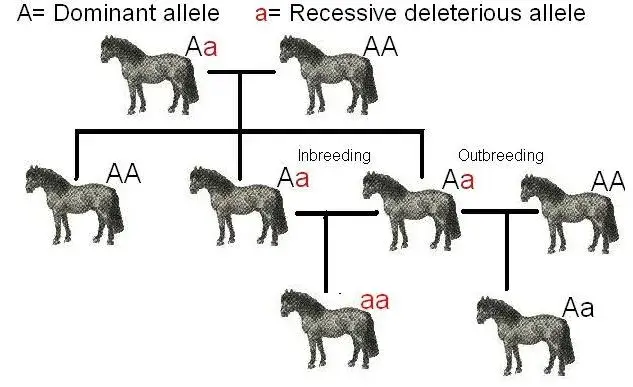
History of Inbreeding depression
History of Inbreeding Depression
- Early Recognition: Inbreeding depression has long been acknowledged due to its detrimental effects. Many cultures historically prohibited marriages between closely related individuals to avoid these negative outcomes. This suggests an early awareness of the issues associated with inbreeding, even before scientific understanding was fully developed.
- Systematic Observations: The systematic study of inbreeding began around the early 18th century. Inbreeding practices became common in cattle breeding by this time. While some improvements in production were noted, there was a marked decline in fertility, which led to the necessity of outcrossing inbred lines to restore vigor.
- Darwin’s Contribution: In 1876, Charles Darwin published “Cross and Self Fertilization in the Vegetable Kingdom,” where he noted that plants resulting from self-fertilization tended to be weaker compared to those from cross-fertilization. This work provided some of the earliest documented evidence of inbreeding depression.
- Maize Studies: The phenomenon of inbreeding depression was further elucidated through experiments on maize. In the early 20th century, researchers like East (1908) and Shull (1909) published detailed accounts on the effects of inbreeding in maize, highlighting its negative impact on plant performance.
- Broader Impact: Subsequent studies extended these findings to other crop plants and species. It became evident that inbreeding adversely affects cross-pollinated and asexually propagated species, often with severe consequences for their overall health and productivity.
Effects of Inbreeding
Inbreeding, or the mating of closely related individuals, has several significant effects on biological populations. These effects primarily manifest as reductions in vigor, reproductive capacity, and overall fitness. The following outlines the key consequences of inbreeding:
- Appearance of Lethal and Sublethal Alleles: Inbreeding often leads to the expression of lethal and sublethal alleles, which adversely affect survival and reproduction. These alleles may cause defects such as chlorophyll deficiencies (e.g., albinism, chlorina), rootless seedlings, and abnormalities in flower structure. Plants exhibiting these traits generally do not survive or reproduce effectively, resulting in their elimination from the population.
- Reduction in Vigor: Inbreeding typically results in reduced vigor among the progeny. This is evident in shorter and weaker plants, alongside a general decrease in the size of various plant parts. The overall robustness of the population diminishes, impacting its growth and resilience.
- Reduction in Reproductive Ability: The reproductive capacity of inbred populations often declines significantly. Many inbred lines exhibit poor fertility, making them difficult to maintain. Consequently, only a few inbred lines retain sufficient reproductive ability to be useful in breeding programs.
- Separation into Distinct Lines: Inbreeding leads to the population separating into phenotypically distinct lines due to increased homozygosity. This results from the random fixation of alleles in different lines, which increases the variance within the population. Each line becomes genetically distinct and exhibits unique phenotypic characteristics.
- Increase in Homozygosity: With continued inbreeding, each line becomes more homozygous, reducing genetic variation within the line. After several generations, lines approach nearly complete homozygosity (>99 percent). These inbred lines, which are maintained through strict inbreeding practices such as self-fertilization, exhibit reduced variability within the line.
- Reduction in Yield: Inbreeding generally leads to a decrease in yield. Inbred lines often produce significantly lower yields compared to their open-pollinated counterparts. For example, in maize, the best inbred lines yield about half as much as the original open-pollinated varieties. This reduction varies across species, with some, like alfalfa and carrots, experiencing a more substantial yield decrease compared to others like onions and certain cucurbits, where the reduction is minimal.
Degrees of Inbreeding Depression
Inbreeding depression varies significantly among plant species, with effects ranging from severe to minimal or even absent. These variations can be categorized into four broad categories:
- High Inbreeding Depression:
- Characteristics: Species exhibiting high inbreeding depression, such as alfalfa (Medicago sativa), carrot (Daucus carota), and hayfield tarweed, experience severe detrimental effects. A significant proportion of plants produced by self-fertilization display lethal traits and fail to survive.
- Consequences: The loss of vigor and fertility is profound, resulting in very few lines being maintained after 3 or 4 generations of inbreeding. The surviving lines typically exhibit yields reduced to less than 25 percent of those from open-pollinated varieties.
- Moderate Inbreeding Depression:
- Characteristics: Crop species like maize (Zea mays), jowar (Sorghum bicolor), and bajra (Pennisetum americanum) show moderate levels of inbreeding depression. These species produce both lethal and sublethal types in selfed progeny, but a substantial portion of the population can be sustained through self-pollination.
- Consequences: While there is a noticeable reduction in fertility and some lines reproduce poorly, the production and maintenance of inbred lines are relatively easier compared to species with high inbreeding depression.
- Low Inbreeding Depression:
- Characteristics: Several crop plants, including onion (Allium cepa), various cucurbits, rye (Secale cereale), sunflower (Helianthus annuus), hemp (Cannabis sativa), and timothy grass, exhibit low inbreeding depression. These species show only a small proportion of plants with lethal or subvital characteristics.
- Consequences: The loss of vigor and fertility is minimal, and maintenance of inbred lines is generally feasible. Yield reduction due to inbreeding is often small or absent, with some inbred lines producing yields comparable to or even exceeding those of open-pollinated varieties.
- Absence of Inbreeding Depression:
- Characteristics: Self-pollinated species often do not exhibit inbreeding depression. This absence of depression is attributed to their reproductive strategy, which leads to homozygous balance within the population.
- Consequences: Although these species do not show inbreeding depression, they may display heterosis (hybrid vigor). This is in contrast to cross-pollinated species, which maintain a heterozygous balance and are more prone to inbreeding depression.
What is heterosis?
- Heterosis, first introduced by Shull in 1914, refers to the phenomenon where an F1 hybrid exhibits superior traits compared to both of its parent lines. This superiority is often seen in increased vigor, size, growth rate, or yield. Heterosis can also manifest as cases where the hybrid shows reduced performance compared to the stronger parent, though this is less commonly discussed.
- The effectiveness of heterosis is typically measured in relation to the average performance of the two parent lines, known as mid-parent heterosis. However, in practical breeding contexts, the more relevant measure is heterobeltiosis, which compares the hybrid’s performance to the superior parent. This form of heterosis is often emphasized because it provides a clearer indication of the hybrid’s potential advantages over its better-performing parent.
- For commercial and practical applications, the most important measure is economic or useful heterosis. This estimate compares the hybrid to the best available commercial variety of the crop. If the hybrid does not surpass the best commercial variety, it may have limited practical value despite its advantages over the parent lines.
- In 1944, Powers suggested that heterosis should be specifically used to describe hybrids that are either superior or inferior to both parent lines, with other outcomes being classified as instances of dominance. This approach aims to clarify the concept and reduce confusion stemming from various terminologies used to describe hybrid performance.
- Heterosis is sometimes used interchangeably with hybrid vigor, although the latter term specifically describes the superiority of hybrids over their parents. The term luxuriance refers to the increased vigor and size of interspecific hybrids, but unlike heterosis, luxuriance often involves hybrids that are less fertile or sterile.
- Overall, heterosis represents a critical concept in plant breeding and hybrid development, reflecting the potential for hybrids to exceed the performance of their parent lines in various desirable traits.
Definition of heterosis
Heterosis: The phenomenon where hybrid offspring exhibit superior traits or enhanced biological functions compared to their parent strains, often due to increased genetic diversity from mating genetically distinct individuals.
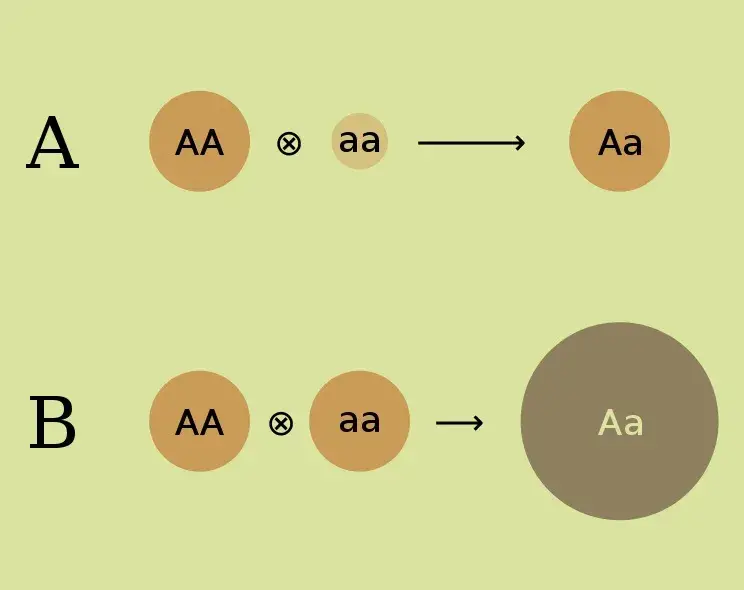
History of heterosis
- Early Observations:
- Koelreuter’s Discovery (1673): Hybrid vigor, or heterosis, was first observed in artificial tobacco (Nicotiana sp.) hybrids by Koelreuter. His work marked the initial recognition of enhanced vigor in hybrid plants.
- Subsequent Reports: Following Koelreuter, various researchers documented hybrid vigor in numerous plant species. These hybrids arose from both interspecific and intraspecific crosses.
- Darwin’s Contributions (1876):
- Darwin’s Conclusion: Charles Darwin concluded that hybrids produced from unrelated plant types exhibited high vigor. His observations laid foundational knowledge for understanding heterosis in hybrid plants.
- Development in Maize Research:
- Beal’s Studies (1877-1882): Beal’s research focused on intervarietal hybrids in maize (Zea mays). He reported that some F1 hybrids yielded up to 40 percent more than their parental varieties, highlighting significant hybrid vigor.
- Differential Heterosis: It became evident that heterosis varied among hybrids. Crosses between genetically diverse varieties exhibited greater heterosis compared to crosses involving closely related varieties.
- Advancements in Maize Hybridization:
- Early 20th Century Work: The development of hybrid maize, crucial for modern agriculture, was significantly advanced by the work of East and Shull in the early 1900s. They demonstrated that crossing inbred lines produced superior hybrids.
- Inbred Lines vs. Open-Pollinated Varieties: Hybrids derived from inbred lines, particularly those from unrelated inbreds, consistently showed greater heterosis than those from open-pollinated varieties. Inbred lines from the same variety or closely related varieties yielded hybrids with only minimal heterosis.
- Commercial Hybrid Production:
- Techniques and Applications: Commercial maize hybrids are now produced by crossing inbred lines rather than open-pollinated varieties. This method, refined through extensive research, has also been applied successfully to other cross-pollinated species like bajra and jowar.
- Variation in Hybrid Performance: Certain inbred lines produce consistently better hybrids than others, and specific cross combinations outperform others. This has led to refined hybridization techniques and improved crop varieties.
Heterosis in Cross-Pollinated and Self-Pollinated Species
- Heterosis in Cross-Pollinated Species:
- General Observations: Cross-pollinated species typically exhibit significant heterosis, particularly when hybridization involves inbred lines. This phenomenon is evident in many commercially exploited crops.
- Commercial Examples: Crops such as onion (Allium cepa) and alfalfa (Medicago sativa) have demonstrated substantial heterosis. This has led to the successful commercial use of hybrids in these species.
- Heterosis in Self-Pollinated Species:
- Magnitude of Heterosis: Self-pollinated species generally show a smaller degree of heterosis compared to cross-pollinated species. However, in some self-pollinated crops, the heterosis observed is sufficient to produce hybrid varieties with commercial value.
- Examples in Vegetables: Certain self-pollinated vegetables, such as tomato (Lycopersicon esculentum), benefit from hybridization. In these cases, a single fruit can yield a large number of seeds, making the production of hybrid varieties feasible.
- Challenges in Self-Pollinated Crops:
- Production Difficulties: Despite the presence of heterosis, the primary challenge in self-pollinated crops is not the lack of heterosis but the difficulty in producing large quantities of hybrid seeds. This issue impacts the commercial viability of hybrids in some self-pollinated species.
Manifestations of Heterosis
Heterosis, or hybrid vigor, refers to the superiority of hybrid offspring over their parental lines. This phenomenon can be observed in several distinct ways, reflecting improvements in various physiological and developmental traits.
- Increased Yield:
- Description: A primary manifestation of heterosis is an increase in yield. This enhancement can be measured in different parts of the plant, including grains, fruits, seeds, leaves, tubers, or the whole plant.
- Importance: Yield enhancement is crucial in plant breeding, as higher yields are often the main objective of crop improvement programs.
- Increased Reproductive Ability:
- Description: Hybrids exhibiting heterosis often show improved reproductive capabilities. This is evident in the higher production of seeds, fruits, or other propagules, such as tubers in potatoes (Solanum tuberosum) or stems in sugarcane (Saccharum officinarum).
- Significance: Enhanced reproductive ability contributes to greater productivity and efficiency in crop production.
- Increased Size and General Vigour:
- Description: Hybrids generally display greater size and vigour compared to their parents. This increase in size typically results from a higher number and larger size of cells in various plant tissues.
- Impact: Enhanced vigour is associated with faster growth rates and overall plant health.
- Improved Quality:
- Description: Many hybrids show superior quality traits, which may not always correlate with higher yields. For instance, hybrid onions may have better keeping quality compared to open-pollinated varieties, despite similar or lower yields.
- Relevance: Quality improvements can be crucial for specific market requirements and product standards.
- Earlier Flowering and Maturity:
- Description: Hybrids often flower and mature earlier than their parent plants. This trait is particularly advantageous in vegetable crops, where earlier harvesting can be beneficial.
- Consideration: Although earlier maturity may sometimes result in a lower total plant weight, the benefit of faster harvest can outweigh this drawback.
- Greater Resistance to Diseases and Pests:
- Description: Some hybrids exhibit increased resistance to diseases and pests compared to their parents. This enhanced resistance can lead to reduced need for chemical treatments and lower crop losses.
- Advantage: Improved disease and pest resistance contributes to more sustainable agricultural practices.
- Greater Adaptability:
- Description: Hybrids typically show better adaptability to environmental changes than inbred lines. This greater adaptability is often reflected in reduced variance in performance across different environments.
- Explanation: This trait may be attributed to the physiological advantages conferred by heterosis, which improves the plant’s resilience to environmental stress.
- Faster Growth Rate:
- Description: In some cases, hybrids demonstrate a faster growth rate than their parents, though the total plant size may not necessarily be larger.
- Impact: A faster growth rate can lead to quicker crop cycles and more efficient use of growing conditions.
- Increased Number of Plant Parts:
- Description: Certain hybrids show an increase in the number of nodes, leaves, or other plant parts without a corresponding increase in total plant size. This trait has been observed in crops like beans (Phaseolus vulgaris).
- Significance: Such increases in plant parts can influence crop management and harvest strategies.
Dominance Hypothesis
The Dominance Hypothesis, initially proposed by Davenport in 1908 and subsequently expanded by Bruce, Keeble, and Pelew in 1910, offers an explanation for the observed phenomenon of heterosis, or hybrid vigor. This hypothesis focuses on the roles of dominant and recessive alleles in determining hybrid performance.
- Basic Concept:
- Dominant and Recessive Alleles: According to the Dominance Hypothesis, at each genetic locus, the dominant allele typically exerts a favorable effect, while the recessive allele tends to have an unfavorable impact. In a heterozygous state, the presence of a dominant allele can mask the detrimental effects of a recessive allele.
- Impact on Hybrid Vigor: The hypothesis suggests that heterosis arises not simply from the heterozygosity of hybrids but from the masking of harmful recessive alleles. Therefore, hybrids display enhanced traits because the recessive alleles with deleterious effects are suppressed by the dominant alleles from the other parent.
- Inbreeding Depression:
- Mechanism: Inbreeding leads to an increase in homozygosity, which brings recessive alleles into the homozygous state. Many of these recessive alleles are harmful, contributing to reduced vigor and fertility in inbred lines.
- Contrast with Heterosis: While inbreeding results in the expression of harmful recessive alleles, leading to inbreeding depression, heterosis arises from the prevention of these harmful effects in hybrids.
- Open-Pollinated Populations:
- Heterozygosity: In open-pollinated populations, plants maintain high levels of heterozygosity, which helps in masking the effects of deleterious recessive alleles. Therefore, these populations generally do not exhibit the harmful effects of recessive alleles that become homozygous in inbred lines.
- Inbreeding Effects: When inbreeding occurs, the proportion of homozygous recessive alleles increases, leading to reduced plant vigor and fertility due to the expression of harmful recessive traits.
- Hybrid Performance:
- Influence of Parental Genotypes: The effectiveness of heterosis in F1 hybrids depends significantly on the genetic composition of the parent plants. Hybrids resulting from parents with differing recessive and dominant alleles are more likely to exhibit heterosis.
- Diverse Origins: Typically, hybrids produced from parents of diverse genetic backgrounds show more pronounced heterosis compared to those from parents with similar genetic backgrounds.
- Practical Implications:
- Inbred Lines: The hypothesis posits that isolating inbred lines with all dominant alleles present would result in vigor comparable to or greater than that of open-pollinated varieties. However, practical challenges have prevented the successful isolation of such inbred lines to date.
- Hybrid Breeding: The Dominance Hypothesis informs breeding strategies, emphasizing the importance of parental genetic diversity to maximize hybrid vigor.
Objections to the Dominance Hypothesis
The Dominance Hypothesis, despite its contributions to understanding heterosis, faces two significant objections based on empirical observations and theoretical considerations:
- Failure to Isolate Vigorous Inbreds:
- Expectation: The Dominance Hypothesis posits that inbreds containing all dominant alleles should exhibit the same level of vigor as F1 hybrids. According to the hypothesis, if all dominant alleles are present, these inbreds should be as vigorous as hybrids due to the masking of harmful recessive alleles.
- Observation: In practice, no such inbreds with complete dominance have been successfully isolated. The anticipated vigor of these inbreds has not materialized, suggesting a gap in the hypothesis.
- Illustrative Example: The hypothesis predicts that inbreds with all dominant alleles (e.g., AABBCCDD) should be more vigorous than those with a mix of dominant and recessive alleles. However, inbred lines have not consistently shown this expected vigor when compared to hybrids, leading to doubts about the hypothesis.
- Symmetrical Distribution in F2 Generations:
- Expectation: According to the Dominance Hypothesis, the segregation of dominant and recessive traits in the F2 generation should result in a distribution ratio that reflects the number of genes involved. For quantitative characters, this would lead to a non-symmetrical distribution due to the complex interactions between multiple genes.
- Observation: Empirical evidence shows that F2 generations often display a symmetrical distribution of quantitative traits, contrary to the prediction of the hypothesis.
- Theoretical Issue: The hypothesis suggests that if the number of genes controlling a trait is large, the distribution should deviate from symmetry. Instead, symmetrical distributions are commonly observed.
Explanations for the Objections:
- Dominance of Linked Genes Hypothesis:
- Jones’s Proposal (1917): To address the issue of failing to isolate vigorous inbreds, Jones suggested that the linkage of dominant and recessive genes might explain these discrepancies. In this view, quantitative characters controlled by many linked genes would still show symmetrical distributions in the F2 generation. This hypothesis posits that even if dominant alleles were linked with recessive ones, the overall distribution could appear symmetrical due to the effects of linkage.
- Implications: If dominant and recessive alleles are linked, the patterns observed in quantitative traits might still align with the Dominance Hypothesis despite not isolating perfect inbreds.
- Symmetrical Distribution in F2:
- Collins’s Findings (1921): Collins demonstrated that a large number of genes controlling quantitative traits could still produce a symmetrical distribution, even in the absence of linkage. This finding implies that the expected non-symmetrical distribution predicted by the Dominance Hypothesis does not hold in practical scenarios.
- Environmental Effects: The symmetry in F2 distributions might also result from environmental factors and the inherent variability of traits, which can overshadow theoretical expectations based on genetic dominance alone.
Overdominance Hypothesis
The Overdominance Hypothesis, independently introduced by East and Shull in 1908, provides a theoretical framework for understanding heterosis, or hybrid vigor. This hypothesis, also known as single-gene heterosis, super-dominance, or the cumulative action of divergent alleles, posits that heterozygotes exhibit superior traits compared to either homozygote due to the advantageous interactions between divergent alleles.
Key Points of the Overdominance Hypothesis:
- Concept of Superiority in Heterozygotes:
- Definition: According to this hypothesis, the heterozygous state (e.g., Aa) is superior to both homozygous states (e.g., AA and aa). This enhanced performance of heterozygotes is attributed to the beneficial effects arising from the presence of two different alleles at a locus.
- Implication: This superiority is thought to stem from the combined functional capabilities of divergent alleles, which allows the heterozygote to outperform both homozygous forms.
- Divergence of Alleles:
- Allelic Diversity: East (1936) proposed that at each genetic locus, multiple alleles (e.g., a1, a2, a3, etc.) exist, each with distinct and progressively different functions. Heterozygotes possessing more divergent alleles are theorized to show greater heterosis.
- Functional Contribution: The hypothesis assumes that each allele contributes differently to the phenotype, and the combination of these divergent functions in heterozygotes enhances the overall performance of the hybrid.
- Performance of Hybrids:
- Enhanced Functionality: The hybrid, being heterozygous for highly divergent alleles, can perform the functions of both alleles, which is not possible in homozygotes. This functional complementarity in heterozygotes results in greater vigor and superior performance.
- Example: For instance, if alleles a1 and a2 perform different functions beneficial to plant growth, a heterozygote (a1a2) would outperform homozygotes (a1a1 or a2a2) due to the combined functional contributions of both alleles.
- Implications for Inbreeding and Hybrid Vigour:
- Inbreeding Depression: The hypothesis implies that inbreeding, which leads to increased homozygosity, would result in a decrease in vigor and performance due to the loss of advantageous allele interactions. In contrast, hybrids, which maintain heterozygosity, benefit from the functional advantages of divergent alleles.
- Inability to Isolate Vigorous Inbreds: According to the Overdominance Hypothesis, it would be impossible to isolate inbred lines that are as vigorous as F1 hybrids. The loss of heterozygosity and the associated beneficial interactions in inbred lines prevent them from achieving the same level of performance as hybrids.
Evidence for Overdominance
The Overdominance Hypothesis asserts that heterozygotes exhibit superior traits compared to either homozygote due to the interaction of divergent alleles. Although clear-cut examples of heterozygote superiority are limited, evidence supporting this hypothesis can be observed in several biological contexts. Below are key examples and considerations related to the evidence for overdominance:
Examples Demonstrating Overdominance:
- Maize (Zea mays):
- Gene Ma: In maize, the gene Ma influences maturity. The heterozygote Ma ma demonstrates greater vigor and later maturity compared to the homozygotes Ma Ma and ma ma. This difference in performance suggests that the heterozygous genotype benefits from the combined effects of divergent alleles.
- Barley (Hordeum vulgare):
- Chlorophyll Mutants: Gustafsson reported that certain chlorophyll mutants in barley, when heterozygous, produce a larger number of seeds and exhibit increased seed size compared to the homozygous normal varieties. This enhanced productivity supports the overdominance hypothesis.
- Hooded Gene: Heterozygotes for the hooded gene in barley show increased photosynthetic rates relative to homozygotes. The enhanced photosynthetic ability indicates that the heterozygous state provides functional advantages not present in the homozygous conditions.
- Humans (Homo sapiens):
- Sickle Cell Anemia: The sickle cell trait, caused by a recessive gene that is lethal in the homozygous state, demonstrates a form of overdominance. In regions where malaria is prevalent, individuals heterozygous for the sickle cell gene (Ss) possess increased resistance to malaria compared to individuals with normal hemoglobin (SS). This provides a selective advantage and illustrates the benefit of heterozygosity.
- Neurospora crassa (Bread Mold):
- Gene pab: In Neurospora crassa, the pab gene, involved in the synthesis of p-aminobenzoic acid, shows that heterozygotes (pab pab) exhibit faster growth rates and greater vigor compared to both homozygous conditions (pab pab and pab* pab*). This growth advantage aligns with the predictions of the overdominance hypothesis.
Limitations and Considerations:
- Limited Number of Clear-Cut Cases:
- The evidence for overdominance is not universally applicable. The number of genes where heterozygote superiority is well-established is relatively small. Many examples involve complex genetic interactions that do not exclusively support overdominance.
- Alternative Explanations:
- Linkage and Epistasis: In some cases, the observed superiority of heterozygotes may result from genetic linkages in the repulsion phase or epistatic interactions rather than overdominance. Epistasis involves interactions between non-allelic genes, while overdominance is specifically about interactions between alleles at the same locus.
- Chromosome Segments and Complex Loci: Evidence also exists for heterozygote superiority involving chromosome segments or complex loci. However, these instances may not directly demonstrate overdominance but rather other forms of genetic interactions.
Comparison Between Dominance and Overdominance Hypotheses
Aspect | Dominance Hypothesis | Overdominance Hypothesis |
---|---|---|
Core Idea | Heterozygotes are as good as or only slightly better than dominant homozygotes due to masking of harmful recessive alleles. | Heterozygotes are superior to both homozygotes due to the combined functional effects of divergent alleles. |
Mechanism | Results from the masking of deleterious recessive alleles by dominant alleles. | Results from the superior functionality of heterozygotes due to the cumulative or synergistic effects of divergent alleles. |
Homozygous Performance | Dominant homozygotes are often as effective as heterozygotes, but recessive homozygotes have reduced performance due to harmful traits. | Heterozygotes outperform both homozygotes because they benefit from a broader range of functional alleles. |
Inbreeding Effects | Inbreeding increases homozygosity, exposing recessive deleterious alleles and causing inbreeding depression. | Inbreeding leads to a loss of heterozygosity, which results in decreased vigor and fertility, as heterozygosity is essential for superior performance. |
Genotypic Influence | The level of heterosis is influenced by the genotypes of the parents, with greater genetic diversity generally leading to higher heterosis. | The level of heterosis depends on the genetic divergence between parents, with more divergent parents showing higher heterosis. |
Examples of Superiority | Not many clear-cut cases where heterozygotes are superior to both homozygotes; some examples include maize maturity and certain chlorophyll mutants. | Examples include heterozygotes for sickle cell anemia showing increased malaria resistance, and Neurospora crassa showing faster growth rates. |
Current Understanding | Dominance is generally accepted as a major factor in heterosis, with overdominance and epistasis also contributing. | Overdominance is suggested to be less prevalent; many cases attributed to overdominance may actually be due to epistasis or linkage. |
The physiological basis of heterosis
The physiological basis of heterosis, commonly referred to as hybrid vigor, involves a range of mechanisms that enhance the performance of hybrids compared to their parent inbred lines. This concept has been explored through various physiological and biochemical studies, revealing several potential mechanisms that contribute to the increased vigor observed in hybrids. The following are the primary mechanisms identified:
Mechanisms Leading to Heterosis
- Increased Embryo and Endosperm Size
- Description: Earlier studies suggested that the larger embryo and endosperm size in hybrids compared to inbreds could result in increased growth rates during the seedling stage.
- Observation: While this mechanism was evident in some cases, it was not consistently demonstrated across all hybrid varieties.
- Enhanced Metabolic Activities
- Description: Whaley (1952) proposed that the primary heterotic effects involve growth regulators and enzymes. He suggested that hybrids possess a more efficient enzyme system, allowing them to mobilize stored food materials more effectively than inbreds.
- Implication: This efficiency contributes to superior growth in the early stages of development.
- Reduced Amount of a Single Gene Product
- Description: In some hybrids, the intermediate levels of gene products can enhance vigor. For example, in Neurospora crassa, the gene pab is involved in producing p-aminobenzoic acid. The heterozygote pab+/pab* produces intermediate amounts of this compound, resulting in faster growth compared to the homozygotes.
- Mechanism: The intermediate gene product levels can be optimal for certain physiological processes, thus enhancing growth and development.
- Separate Gene Products
- Description: The two alleles in a heterozygote may produce proteins with distinct functions. Heterozygotes benefit from the combined functions of both proteins, whereas homozygotes only have one type of protein.
- Example: In human sickle cell anemia, heterozygotes (Ss) produce both normal and defective hemoglobin. This combination provides resistance to malaria, whereas homozygotes for the defective allele (ss) do not survive due to compromised hemoglobin function.
- Combined Gene Products
- Description: Many enzymes are dimers or polymers, composed of subunits from different alleles. Hybrids can produce enzyme molecules with distinct properties, such as altered heat sensitivity.
- Example: In the case of octanyl dehydrogenase-I, the heterozygote produces an enzyme that is less heat-sensitive than those produced by homozygotes.
- Effects in Different Tissues
- Description: Heterozygotes may exhibit intermediate enzyme levels in different tissues, resulting from the combined effects of alleles producing high enzyme levels in different tissues.
- Example: In maize, the enzyme Adh shows different activities in various tissues. The hybrid exhibits intermediate activities in both tissues, contributing to overall vigor.
Commercial Applications of Heterosis
Heterosis, commonly known as hybrid vigor, is a phenomenon where hybrids exhibit superior qualities compared to their parent strains. This phenomenon is extensively utilized in agriculture and animal breeding due to its significant advantages in productivity and efficiency. The commercial applications of heterosis are diverse, spanning across various crop species, vegetables, fruit trees, and animals.
Applications in Crop Species
- Cross-Pollinated Crops
- Maize (Zea mays): One of the most prominent examples of heterosis exploitation, maize hybrids demonstrate increased yield, disease resistance, and adaptability. Hybrid maize varieties are extensively used due to their superior performance compared to inbred lines.
- Sorghum (Sorghum bicolor) and Pearl Millet (Pennisetum glaucum): These crops also benefit from heterosis, with hybrids showing enhanced growth and yield potential.
- Sugar Beets (Beta vulgaris): Hybrid varieties exhibit improved root size and sugar content.
- Sunflower (Helianthus annuus): Hybrid sunflowers show higher oil content and better resistance to diseases.
- Asexually Propagated Crops
- Forage Grasses and Forage Legumes: Hybrid varieties can provide superior biomass production and nutritional quality.
- Cotton (Gossypium spp.): Hybrid cotton plants are cultivated for higher fiber yield and better pest resistance.
- Self-Pollinated Crops
- Rice (Oryza sativa): In China, hybrid rice varieties have been developed to achieve higher yields and better resistance to environmental stresses. Despite challenges, hybrid rice has shown substantial improvements in productivity.
Applications in Vegetable Crops
- Tomato (Solanum lycopersicum): Hybrid tomatoes exhibit enhanced fruit size, uniformity, and resistance to diseases.
- Brinjal (Solanum melongena): Hybrid varieties show improved yield and better resistance to pests.
- Onion (Allium cepa) and Cucurbits (e.g., Cucumbers, Squash): Hybrids offer increased fruit quality and yield.
- Brussels Sprouts (Brassica oleracea var. gemmifera): Hybrids provide improved growth and higher yield.
Applications in Fruit Trees
- Fruit Trees: Heterosis is utilized in various fruit tree species to enhance fruit size, flavor, and overall productivity. Hybrid varieties often show better resistance to diseases and environmental conditions.
Applications in Animal Breeding
- Silkworms (Bombyx mori): Hybrid silkworms are bred for increased silk production and better resistance to diseases.
- Poultry: Hybrid chickens and other poultry show improved growth rates, egg production, and disease resistance.
- Cattle and Swine: Hybrid animals often exhibit better growth rates, reproductive performance, and disease resistance compared to purebred counterparts.
Challenges and Limitations
- Seed Production: One of the primary challenges in the commercial application of heterosis is the production of large quantities of hybrid seed. This challenge is particularly pronounced in self-pollinated species with low seed production per fruit.
- Wheat and Barley: Although there have been efforts to exploit heterosis in these self-pollinated species, success has been limited due to the difficulty in producing hybrid seeds and maintaining desired traits.
Difference Between Heterosis and Inbreeding Depression
Aspect | Heterosis | Inbreeding Depression |
---|---|---|
Definition | Enhanced biological functions and traits in hybrid offspring due to genetic diversity. | Reduced biological fitness and performance in offspring from mating closely related individuals. |
Parental Genome | Results from mating genetically distinct individuals. | Results from mating closely related individuals, leading to reduced genetic diversity. |
Genetic Variation of Genomes | High genetic variability between parents contributes to superior traits in hybrids. | Low genetic variation and increased similarity lead to expression of harmful recessive alleles. |
Adaptation to Environment | Offspring are well-adapted to various environmental conditions due to genetic diversity. | Offspring struggle with environmental changes due to reduced genetic variability. |
Characteristics of Offspring | Superior traits like improved growth rates, yield, and disease resistance. | Inferior traits such as reduced growth, lower fertility, and increased disease susceptibility. |
Genetic Variation in Parents | Occurs with genetically distant parents providing diverse genetic material. | Arises from genetically related parents, leading to reduced genetic diversity. |
Homozygosity/Heterozygosity | Increased heterozygosity contributes to enhanced performance. | Increased homozygosity reveals deleterious recessive traits and reduces fitness. |
Appearance of Genetic Variation | Genetic variation noticeable in F1 hybrids even if parents are genetically uniform. | Requires pre-existing genetic variation that becomes detrimental due to increased homozygosity. |
Occurrence | Result of outbreeding, enhancing offspring traits. | Result of inbreeding, negatively impacting offspring traits. |
Effect on Phenotypic Traits | Leads to beneficial increases in traits, improving overall performance. | Results in detrimental reductions in traits, decreasing overall fitness. |
Effect of Genetic Drift in Small Populations | More pronounced in small populations due to the impact of outbreeding. | Less pronounced in small populations; genetic drift can reduce negative effects of inbreeding. |
Likelihood of Outbreeding Depression | May be reduced by outbreeding depression, affecting heterosis magnitude. | Generally unaffected by outbreeding depression unless specific conditions like isolation are present. |
FAQ
Physiological Bases of Heterosis
Heterosis, or hybrid vigor, refers to the phenomenon where hybrid offspring exhibit superior traits compared to their parent lines. The physiological bases of heterosis are complex and involve several mechanisms. Here’s a detailed discussion of the primary physiological bases:- Increased Cell Size and Number
- Embryo and Seed Size: One of the early hypotheses suggested that heterosis resulted from larger embryo and seed sizes in hybrids compared to inbred parents. Larger embryos and seeds often lead to enhanced early growth and development.
- Growth Rate: Hybrids tend to show faster growth rates during the seedling stage due to increased cell size and number. This increase in growth can be attributed to more robust and efficient cellular machinery.
- Improved Metabolic Efficiency
- Enzyme Systems: Whaley (1952) proposed that hybrid embryos have more efficient enzyme systems that mobilize stored food materials more effectively. This metabolic efficiency can result in faster growth and greater vigor during early development.
- Growth Regulators: Hybrids often exhibit enhanced production of growth regulators, which can positively influence various physiological processes, including cell division and elongation.
- Genetic Interactions
- Reduced Amount of a Single Gene Product: In some hybrids, an intermediate level of a gene product may result in increased vigor. For example, in Neurospora crassa, the heterozygous pab+ pab produces intermediate amounts of p-aminobenzoic acid, which promotes faster growth compared to the homozygotes.
- Separate Gene Products: Different alleles may produce proteins with distinct functions. The presence of both protein types in hybrids can enhance adaptability to environmental changes. For instance, in humans, the heterozygous state for sickle cell anemia provides resistance to malaria due to the combined effects of normal and defective hemoglobin.
- Hybrid Enzyme Products
- Combined Gene Products: Many enzymes are composed of multiple subunits from different alleles. Hybrids may produce hybrid enzyme molecules with unique properties compared to those produced by homozygotes. For example, some hybrid enzymes may be less sensitive to heat or other environmental stresses.
- Electrophoretic Patterns: Hybrids often exhibit novel enzyme bands on electrophoretic gels, indicating the presence of new enzyme forms with potentially advantageous properties.
- Effects in Different Tissues
- Enzyme Activity in Multiple Tissues: Some hybrids show superior performance due to the expression of enzymes in multiple tissues at intermediate levels. For example, an enzyme that shows different levels of activity in pollen and other tissues may result in enhanced overall performance in hybrids.
Short Notes
(i) Sickle Cell Anemia- Definition: Sickle cell anemia is a genetic disorder caused by a mutation in the hemoglobin gene, leading to abnormal hemoglobin S (HbS).
- Cause: The mutation involves a single nucleotide change (A to T) in the β-globin gene, resulting in an amino acid substitution (valine for glutamic acid).
- Symptoms: It leads to the production of sickle-shaped red blood cells, which cause blockages in blood vessels, leading to pain, anemia, and organ damage.
- Heterozygote Advantage: Individuals with one sickle cell allele (heterozygotes) are more resistant to malaria, providing a selective advantage in malaria-endemic regions.
- Definition: The pab locus in Neurospora crassa is responsible for the synthesis of p-aminobenzoic acid (PABA), a vital component for growth.
- Gene Interaction: Heterozygotes for the pab locus produce intermediate levels of PABA, resulting in faster growth compared to homozygous mutants that produce either too little or none.
- Significance: This locus provides an example of how heterozygosity can enhance growth through intermediate gene product levels.
- Definition: The Ma gene in maize (Zea mays) influences the maturity and growth of the plant.
- Gene Effect: The heterozygous Ma ma plants exhibit greater vigor and later maturity compared to the homozygous Ma Ma or ma ma plants.
- Application: This gene demonstrates how heterozygotes can show superior traits such as enhanced growth rates and longer periods of anthesis.
- Definition: The Adh (alcohol dehydrogenase) gene in maize has multiple alleles that code for different forms of the enzyme alcohol dehydrogenase.
- Enzyme Function: Different alleles produce enzymes with varying heat sensitivities.
- Heterozygote Advantage: Heterozygotes exhibit an enzyme with improved heat stability compared to the homozygotes, which contributes to better stress resistance.
- Definition: Inbreeding depression refers to the reduced biological fitness in a population resulting from mating between closely related individuals.
- Effects: It leads to an increase in homozygosity for deleterious alleles, resulting in decreased vigor, growth, fertility, and overall fitness.
- Example: In crops, inbreeding depression can reduce yields and increase susceptibility to diseases.
- Definition: Hybrid vigour, or heterosis, is the enhanced growth, yield, or overall performance of hybrids compared to their parent lines.
- Cause: It results from the combination of different genetic backgrounds from the parent lines, often leading to improved traits such as higher yield, greater size, and better disease resistance.
- Application: It is widely utilized in agriculture to produce high-yielding hybrid crops and livestock. Examples include hybrid maize and hybrid vegetables, which often outperform their parent varieties in terms of productivity and resilience.
Dominance and Overdominance Hypotheses of Heterosis
1. Dominance Hypothesis
Main Features:- Basic Idea: The dominance hypothesis posits that heterosis results from the masking of deleterious recessive alleles by dominant alleles in the hybrid. Essentially, the hybrid benefits from the dominance of beneficial traits and the suppression of harmful traits present in the homozygous parent lines.
- Mechanism: In this hypothesis, heterozygotes benefit from having one or more dominant alleles that mask the effects of recessive alleles which might be deleterious. This leads to improved performance in hybrid offspring compared to homozygous parent lines.
- Incomplete Explanation: The dominance hypothesis does not account for the fact that heterosis can be observed in traits not related to simple dominance-recessive interactions.
- Limited Cases: It does not explain heterosis in cases where hybrids do not exhibit straightforward dominance relationships.
- Complex Traits: Advances in genetics have shown that while simple dominance may play a role, other genetic mechanisms such as epistasis and gene interactions also contribute to heterosis.
- Broader Application: Evidence has demonstrated that while dominance may explain some instances of heterosis, the hypothesis is part of a broader set of mechanisms that contribute to hybrid vigor.
2. Overdominance Hypothesis
Main Features:- Basic Idea: The overdominance hypothesis suggests that heterosis results from the hybrid having an advantage due to the interaction between two different alleles at a single locus. This interaction creates a phenotype that is superior to both homozygous parent lines.
- Mechanism: According to this hypothesis, the hybrid exhibits superior traits because the heterozygote has a more optimal expression of the gene product than either of the homozygotes. This optimal expression can lead to enhanced growth, yield, or other beneficial traits.
- Limited Evidence: There are limited cases where overdominance has been conclusively demonstrated, and it is often challenging to separate it from other phenomena such as epistasis.
- Complex Interactions: The overdominance hypothesis may oversimplify the complex interactions that can occur between different genes and alleles.
- Empirical Evidence: Studies in specific cases, such as maize and human sickle cell anemia, have provided evidence supporting overdominance. Advances in molecular genetics continue to uncover more examples.
- Genetic Complexity: Modern genetic research recognizes that overdominance is one of several mechanisms contributing to heterosis, and it works in conjunction with other factors such as epistasis and linkage.
Acceptance of the Hypotheses
More Widely Accepted Hypothesis:- Dominance Hypothesis: The dominance hypothesis is generally more widely accepted, primarily because it can explain a broader range of heterotic effects observed in many crop species and animals. Its simplicity and applicability to a variety of traits make it a practical framework for understanding hybrid vigor.
- Practical Evidence: Dominance effects are easily observable in many breeding programs and can be practically applied to improve traits in crops and livestock.
- Broad Applicability: The dominance hypothesis provides a framework that aligns well with observed phenomena in a wide range of species, including those where detailed genetic mechanisms are not fully understood.
- Integration with Other Mechanisms: While dominance alone may not explain all cases of heterosis, it is often integrated with other mechanisms, such as epistasis and overdominance, to provide a comprehensive understanding of hybrid vigor.
Definition of Heterosis
Heterosis, also known as hybrid vigor, refers to the phenomenon where the hybrid offspring exhibit superior qualities or increased performance compared to their parent lines. This enhanced performance can be observed in terms of growth rate, yield, resistance to diseases, and other physiological traits.Characteristic Features of Heterosis
- Increased Vigor: Hybrids often show greater growth rates and overall robustness compared to their parents.
- Improved Yield: Hybrid crops usually produce higher yields than either of the parent lines, due to better growth efficiency and resource utilization.
- Enhanced Disease Resistance: Hybrids frequently exhibit better resistance to diseases and pests than their parent lines.
- Greater Stress Tolerance: Hybrids can demonstrate superior tolerance to environmental stresses such as drought, salinity, and temperature extremes.
- Improved Quality Traits: Hybrids may also show enhanced quality traits, such as better nutritional content, flavor, or texture.
- Increased Fertility: Some hybrids may exhibit increased reproductive success and higher seed production.
Applications of Heterosis
- Agricultural Crops: Heterosis is widely utilized in the production of hybrid crops, such as maize, cotton, and various vegetables. These hybrids are engineered to maximize yield, disease resistance, and other desirable traits.
- Animal Breeding: In livestock, heterosis is applied to improve productivity and health in animals, including cattle, poultry, and swine. Hybrid vigor can result in better growth rates, milk production, and overall performance.
- Horticulture: Hybrid vigor is used to enhance the quality and yield of fruit trees and ornamental plants. For example, hybrid varieties of tomatoes and peppers are bred for better fruit quality and higher production.
- Forage Crops: Heterosis is applied in breeding forage crops to improve their nutritional value, growth rate, and yield for animal feed.
Achievements in Crop Improvement Using Heterosis
- Maize (Zea mays): The development of hybrid maize varieties has led to significant increases in yield and resistance to diseases and pests. Hybrid maize now dominates global maize production due to its superior performance.
- Cotton (Gossypium spp.): Hybrid cotton varieties have achieved higher yields and improved fiber quality, contributing to increased productivity in cotton farming.
- Vegetable Crops: Hybrid vegetables, such as hybrid tomatoes, cucumbers, and peppers, have demonstrated enhanced yields, better disease resistance, and improved fruit quality.
- Rice (Oryza sativa): In some regions, hybrid rice varieties have been developed that offer higher yields and better adaptability to varying environmental conditions.
- Sunflower (Helianthus annuus): Hybrid sunflowers have been bred to achieve improved oil content and resistance to diseases, enhancing their commercial value.
Main Features of Inbreeding Depression
Inbreeding depression refers to the reduced biological fitness of offspring resulting from the mating of closely related individuals. The key features of inbreeding depression include:- Reduced Vigour: Offspring often show decreased growth rates, reduced biomass, and overall less vigor compared to outbred individuals.
- Decreased Fertility: There is often a reduction in reproductive success, including lower seed set, reduced seed viability, and smaller seed size.
- Increased Susceptibility to Disease: Inbred individuals are typically more susceptible to diseases and pests, as genetic diversity that provides resistance may be diminished.
- Reduced Longevity: Inbred plants and animals may exhibit shortened lifespans or increased susceptibility to environmental stresses.
- Increased Expression of Recessive Defects: Recessive deleterious alleles become more likely to be expressed when both parents contribute similar genetic defects, leading to an increased occurrence of genetic disorders.
- Lower Adaptability: Inbred populations generally have lower adaptability to changing environmental conditions due to a reduced genetic pool.
Degrees of Inbreeding Depression in Crop Species
Inbreeding depression varies among crop species depending on their breeding systems and genetic diversity. The degrees of inbreeding depression encountered in crop species can be summarized as follows:- High Inbreeding Depression:
- Self-Pollinated Crops: Crops that primarily self-pollinate, such as wheat and barley, often show significant inbreeding depression because self-pollination increases homozygosity. However, these species have developed mechanisms to manage or minimize inbreeding effects, such as maintaining genetic diversity through mutation or selection.
- Example: In wheat, inbreeding can lead to poor seedling growth and reduced yields.
- Moderate Inbreeding Depression:
- Cross-Pollinated Crops: These crops, such as maize and sunflower, experience less inbreeding depression compared to self-pollinated crops because they generally maintain higher levels of genetic diversity. Nonetheless, inbreeding in cross-pollinated crops can still result in reduced vigor and productivity if the genetic base becomes narrow.
- Example: In maize, inbred lines used for hybrid production might show inbreeding depression, but hybrid vigor can counteract some of these effects.
- Low Inbreeding Depression:
- Asexually Propagated Crops: Crops that reproduce asexually, such as potatoes and bananas, do not undergo traditional inbreeding depression because they do not involve sexual reproduction. However, these crops can still suffer from reduced vigor due to clonal propagation, which can lead to the accumulation of deleterious mutations.
- Example: In potatoes, while true inbreeding depression is not applicable, clonal crops may still exhibit reduced vigor due to the accumulation of genetic defects.
Here is a detailed differentiation between the terms:
(i) Homozygous vs. Homogeneous Lines
- Homozygous Lines:
- Definition: Refers to individuals or lines where both alleles at a particular gene locus are identical, whether dominant or recessive (e.g., AA or aa).
- Characteristics: Homozygous lines are uniform for specific genetic traits because they have consistent allele pairs across generations.
- Use: Essential for producing predictable and uniform offspring in breeding programs.
- Homogeneous Lines:
- Definition: Refers to a population or line that exhibits uniformity in traits or characteristics, but this uniformity can result from either genetic (homozygosity) or environmental factors.
- Characteristics: The term does not necessarily imply genetic uniformity but indicates that the observed traits are consistent within the line.
- Use: Applied in various contexts, including both genetic and phenotypic uniformity.
(ii) Homozygous vs. Heterozygous Balance
- Homozygous Balance:
- Definition: Refers to a state where the genetic makeup of an individual or line is uniformly homozygous for specific loci, contributing to stability in expression of traits.
- Characteristics: There is no variation at the specific loci due to the presence of identical alleles. This often results in predictable phenotypic outcomes.
- Heterozygous Balance:
- Definition: Refers to a state where an individual has two different alleles at a particular gene locus (e.g., Aa), leading to a balance in the expression of traits influenced by both alleles.
- Characteristics: Heterozygosity can lead to intermediate phenotypic effects or dominance effects depending on the nature of the alleles involved.
(iii) Genetic Code vs. Gene Pool
- Genetic Code:
- Definition: The set of rules by which the information encoded in genetic material (DNA or RNA sequences) is translated into proteins. It includes codons that specify amino acids.
- Characteristics: Universal among most organisms, translating nucleotide sequences into amino acids and proteins.
- Use: Fundamental for understanding molecular biology and genetics, influencing protein synthesis.
- Gene Pool:
- Definition: The total collection of genes and their variants (alleles) in a population.
- Characteristics: Represents the genetic diversity available within a breeding population and influences evolutionary processes and genetic variation.
- Use: Important for studying genetic diversity, population genetics, and evolutionary biology.
(iv) Heterosis vs. Luxuriance
- Heterosis (Hybrid Vigor):
- Definition: The phenomenon where hybrid offspring exhibit greater vigor, growth, yield, or other advantageous traits compared to their parent lines.
- Characteristics: Results from the genetic combination of different parental lines, leading to improved performance.
- Use: Exploited in agriculture to produce high-yielding and resilient crop varieties.
- Luxuriance:
- Definition: Refers to the exuberant growth or abundance of vegetative parts in plants.
- Characteristics: Often seen as a result of favorable growing conditions rather than genetic improvements.
- Use: Observed in plant growth studies but not specifically linked to genetic improvement.
(v) Heterosis vs. Hybrid Vigor
- Heterosis:
- Definition: A broader term encompassing the general phenomenon where hybrid offspring exhibit superior traits compared to their parents.
- Characteristics: Includes various forms of improvement such as increased growth, yield, or resistance due to genetic diversity.
- Use: Used interchangeably with hybrid vigor in many contexts.
- Hybrid Vigor:
- Definition: Specifically refers to the enhanced performance and robustness observed in hybrid offspring.
- Characteristics: Focuses on the increased physical vigor, growth, and productivity seen in hybrids.
- Use: Often used in agricultural and horticultural contexts to describe improvements in hybrid plants and animals.
(vi) Dominance vs. Overdominance
- Dominance:
- Definition: A genetic phenomenon where one allele masks the expression of another allele at the same locus.
- Characteristics: In a heterozygous state, the dominant allele's trait is expressed while the recessive allele's effect is hidden.
- Example: In pea plants, the allele for tall height (T) is dominant over the allele for short height (t).
- Overdominance:
- Definition: A situation where the heterozygote exhibits superior traits compared to both homozygotes for the same gene.
- Characteristics: The heterozygote shows enhanced performance or fitness compared to both homozygous dominant and recessive individuals.
- Example: Sickle cell trait in humans, where heterozygotes (AS) show resistance to malaria compared to homozygotes (AA or SS).
Definitions
- Inbreeding Depression: The reduction in biological fitness and vigor observed in offspring resulting from mating between closely related individuals. This decline in fitness often manifests as decreased fertility, lower survival rates, and overall reduced performance due to the increased expression of deleterious recessive alleles and reduced genetic diversity.
- Hybrid Vigor (Heterosis): The increased vigor, growth, or yield observed in hybrid offspring compared to their parent strains. This enhancement results from the combination of diverse genetic backgrounds, which can lead to improved overall performance, such as better disease resistance, higher yield, and greater adaptability.
Effects on Self-Pollinated and Cross-Pollinated Species
1. Self-Pollinated Species- Inbreeding Depression: In self-pollinated species, inbreeding depression is more pronounced due to the continuous self-fertilization leading to homozygosity. Over generations, self-pollination can increase the likelihood of expressing harmful recessive traits, reducing the fitness of the plants. For instance, in self-pollinated crops like wheat and barley, persistent self-pollination can result in reduced grain size, lower yields, and increased susceptibility to diseases due to the accumulation of deleterious alleles.
- Hybrid Vigor: The application of hybrid vigor in self-pollinated species is more challenging. While hybrid varieties are developed, maintaining genetic diversity and producing hybrid seeds in large quantities can be difficult. However, some successful examples include hybrid rice, which has shown significant yield improvements compared to its inbred counterparts. The production of hybrid varieties in such crops involves creating hybrids through controlled cross-pollination before self-pollination resumes.
- Inbreeding Depression: Cross-pollinated species generally experience less inbreeding depression because they maintain genetic diversity through mating with unrelated individuals. However, if these species are subjected to inbreeding or self-pollination, such as in breeding programs or isolated populations, they can still suffer from reduced fitness. For example, inbreeding in maize (a cross-pollinated species) can lead to poorer growth and lower yields.
- Hybrid Vigor: Hybrid vigor is often prominently exploited in cross-pollinated species. Cross-pollination naturally introduces genetic diversity, and hybrid varieties of such species frequently exhibit enhanced growth, yield, and disease resistance. Maize is a classic example, where hybrid varieties have significantly outperformed inbred lines in terms of yield and adaptability. Similarly, hybrid varieties of sunflower and cotton show improved performance over their inbred counterparts.
Examples
- Self-Pollinated Species: In wheat, continuous self-pollination can result in inbreeding depression, which manifests as reduced seed size and lower yield. Hybrid varieties of rice, produced through controlled cross-pollination, demonstrate hybrid vigor with increased yield and resistance to stress.
- Cross-Pollinated Species: In maize, hybrid varieties show remarkable hybrid vigor, with increased growth and yield compared to inbred varieties. This phenomenon is utilized extensively in commercial maize production to maximize crop performance.
- https://course.cutm.ac.in/wp-content/uploads/2020/06/Heterosis-inbreeding-depression-various-theories-of-Heterosis.pdf
- http://www.eagri.org/eagri50/GBPR211/lec21.pdf
- https://pbea.agron.iastate.edu/files/2021/11/Inbreeding-and-Heterosis.pdf
- https://www.ramauniversity.ac.in/online-study-material/agriculture/bsc/iiisemester/fundamentalsofplantbreeding/lec%2010.pdf
- https://www.researchtrend.net/bfij/pdf/97%20Heterosis,%20Residual%20Heterosis%20and%20Inbreeding%20Depression%20Study%20in%20Tomato%20[Solanum%20lycopersicum%20L.]%20Hiteksha%20I.%20Damor.pdf
- https://www.differencebetween.com/difference-between-heterosis-and-vs-inbreeding-depression/
- https://pediaa.com/what-is-the-difference-between-heterosis-and-inbreeding-depression/#google_vignette
- https://course.cutm.ac.in/wp-content/uploads/2020/06/Heterosis-inbreeding-depression-various-theories-of-Heterosis.pdf
- https://www.researchgate.net/figure/differences-between-inbreeding-depression-and-heterosis_tbl1_38015729