What is Glycogen?
- Glycogen is a highly branched polysaccharide composed of glucose units, serving as the primary form of glucose storage in animals, fungi, and bacteria. It functions as a crucial energy reserve, particularly in humans, where it is stored predominantly in the liver and skeletal muscles. In these tissues, glycogen acts as a rapidly mobilized source of glucose when the body requires energy, playing an essential role in maintaining blood glucose levels and providing fuel for cellular activities.
- The structure of glycogen is similar to amylopectin, a form of starch found in plants, but it differs by being more extensively branched, which enhances its solubility and rate of metabolism. Glycogen molecules consist of glucose residues connected via alpha-1,4 and alpha-1,6 glycosidic bonds. This branching structure facilitates rapid breakdown, allowing glucose to be released quickly in response to the body’s energy demands. Glycogen is stored in the form of granules within the cytoplasm of cells, particularly in the liver, where it accounts for about 5-6% of the organ’s fresh weight, and in skeletal muscles, which store a greater total amount by weight, though at a lower concentration.
- In the liver, glycogen serves as a glucose reservoir to maintain blood glucose levels, especially when fasting. The liver’s glycogen stores are mobilized to release glucose into the bloodstream to maintain homeostasis. In contrast, glycogen stored in skeletal muscles is used primarily by the muscle cells themselves to fuel contraction and energy production during physical activity. Glycogen depletion in muscle cells reduces their ability to uptake glucose from the blood, increasing blood glucose levels for other tissues. The brain, for instance, consumes a significant portion of the blood glucose, especially in fasting conditions, underscoring the importance of glycogen in overall metabolic function.
- The storage capacity of glycogen varies depending on factors such as muscle fiber type, physical training, diet, and metabolic rate. Type 1 muscle fibers, associated with endurance activities, tend to store more glycogen compared to type 2 fibers. Regular exercise and dietary habits significantly influence the extent to which glycogen is stored, and these stores are replenished through dietary carbohydrates.
- Regulation of glycogen metabolism is highly controlled by hormones such as insulin and glucagon. Insulin promotes glycogen synthesis by signaling cells to absorb glucose and convert it into glycogen for storage, while glucagon triggers the breakdown of glycogen into glucose when blood sugar levels are low. Any disruption in the enzymes responsible for glycogen synthesis or degradation can lead to metabolic disorders, emphasizing the importance of proper glycogen regulation for overall health.
- In addition to its roles in humans and animals, glycogen also serves as an energy reserve in various microorganisms and some plants, although it is not found in plant tissues. Its rapid mobilization and efficient storage make glycogen an essential component of energy metabolism, vital for maintaining cellular functions and supporting physical activity.
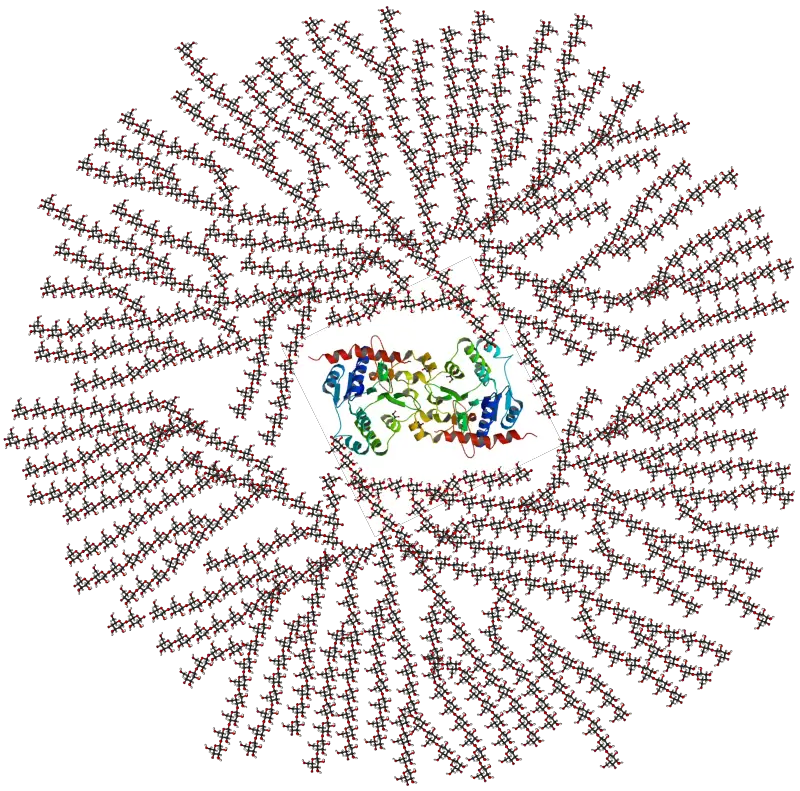
Definition of Glycogen
Glycogen is a branched polysaccharide made of glucose units that serves as the primary form of glucose storage in animals, fungi, and bacteria. It is primarily stored in the liver and muscles and acts as a readily mobilized energy source when the body needs glucose.
Characteristics of Glycogen
Glycogen is a highly branched polysaccharide that serves as an energy reserve in animals. It is composed of glucose molecules and has distinct structural and functional characteristics that make it effective for energy storage and mobilization. Below are key characteristics of glycogen:
- Composition:
Glycogen consists of glucose units linked by alpha-1,4 glycosidic bonds in linear chains, with alpha-1,6 bonds creating branching points. This branching structure makes glycogen more compact and soluble than other polysaccharides, such as starch. - Granular Form:
In cells, glycogen is stored in granules. These granules are primarily found in the liver and skeletal muscles. Each granule has a core protein that aids in glycogen synthesis. The granules also contain water, with a ratio of approximately 3 to 4 parts water per part glycogen, aiding its solubility. - Location:
The largest glycogen stores are found in the liver, where glycogen can be broken down and released into the bloodstream. Skeletal muscles store glycogen as a local energy reserve for muscle contractions during physical activity. Smaller amounts of glycogen are present in other tissues, such as the brain and heart. - Energy Reserve:
Glycogen acts as a readily available source of glucose. During periods of fasting or high-intensity exercise, glycogen can be broken down into glucose, which is then used to maintain blood glucose levels and fuel muscle activity. - Regulation of Blood Sugar:
Liver glycogen plays a crucial role in regulating blood glucose levels. When blood sugar decreases, the hormone glucagon signals the liver to convert stored glycogen back into glucose and release it into the bloodstream. This process is vital for preventing hypoglycemia. - Muscle Fuel:
In skeletal muscles, glycogen is used for energy during exercise. However, muscle cells cannot release glucose into the bloodstream due to the lack of glucose-6-phosphatase, limiting glycogen’s role to local energy supply for muscle contractions. - Protection Against Hypoglycemia:
Glycogen reserves are critical for maintaining stable blood sugar levels, especially during fasting or intense physical activity. By ensuring a continuous supply of glucose, glycogen helps prevent hypoglycemia (low blood sugar). - Glycogenesis:
The synthesis of glycogen from glucose is known as glycogenesis. This process involves several enzymatic steps: glucose is first converted into glucose-6-phosphate, then into glucose-1-phosphate, and finally into UDP-glucose before being polymerized into glycogen. - Glycogenolysis:
The breakdown of glycogen into glucose is referred to as glycogenolysis. The enzyme glycogen phosphorylase plays a key role in cleaving glucose units from glycogen. These glucose units are then converted into glucose-6-phosphate, which can be used for energy production in various tissues.
- Concentration in Tissues:
- Liver cells store significant amounts of glycogen for systemic glucose regulation.
- Skeletal muscles hold the largest quantity of glycogen by weight, though they cannot release it into the bloodstream.
- Plant tissues do not contain glycogen, relying on starch as their primary carbohydrate storage.
- Chemical Structure:
- Glycogen consists of glucose molecules linked by two types of glycosidic bonds: alpha-1,4 bonds form linear chains, while alpha-1,6 bonds create branching points.
- These branching points are essential for glycogen’s function, as they increase solubility and provide more sites for enzymatic breakdown.
- Branching and Solubility:
- Branching of glycogen is crucial for enhancing its water solubility.
- The branches create multiple points of breakdown, ensuring the stored energy can be mobilized quickly when needed.
- Glycogen Metabolism:
- Glycogen breakdown is triggered during low-energy states, whereas its synthesis occurs when energy is abundant.
- This regulation helps maintain glucose homeostasis in the body.
- Hormonal Regulation:
- Insulin signals the body to store glucose as glycogen when energy levels are high.
- Glucagon signals glycogen breakdown in response to low blood glucose, releasing glucose into circulation.
- These hormones work in tandem to balance glycogen metabolism and maintain proper energy supply.
- Limitations of Skeletal Muscle:
- Skeletal muscle lacks glucose-6-phosphatase (G6Pase), an enzyme required for releasing glucose into the bloodstream.
- As a result, muscle glycogen is reserved solely for use within the muscle cells during periods of activity or energy need.
Glycogen’s structure and regulation reflect its essential role in energy storage and release, ensuring glucose levels are maintained during periods of activity and rest.
Structure of Glycogen
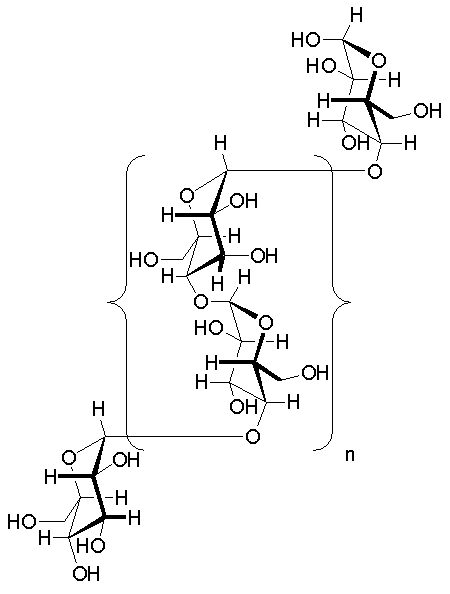
Glycogen is a branched polysaccharide composed of glucose units, serving as a vital energy reserve in animals. Its structure is engineered for efficiency in both storage and mobilization, making it an essential component of metabolic processes.
- Composition:
Glycogen is made up of long chains of glucose units linked by alpha-1,4 glycosidic bonds. The branching occurs at approximately every 8–12 glucose units, connected by alpha-1,6 glycosidic bonds. These bonds create a helical structure that is compact and highly soluble, enabling quick energy release. - Branching Structure:
The glycogen molecule is highly branched, which is more extensive than other polysaccharides like amylopectin. This structure increases the molecule’s solubility and provides a greater number of nonreducing ends for simultaneous enzymatic activity, allowing rapid mobilization of glucose when needed. Branches occur every 8 to 12 glucose units. - Granular Formation:
Glycogen is stored in the cytoplasm of cells as granules, with sizes ranging from 10–40 nm in diameter. These granules contain a core protein called glycogenin, which facilitates glycogen synthesis. The granules are hydrated, with glycogen absorbing three to four parts water per part glycogen. - Molecular Size Variability:
Glycogen molecules can vary widely in size, ranging from 2,000 to over 600,000 glucose units per molecule. This variability allows glycogen to adapt to the metabolic needs of different tissues. - Beta-Particles and Alpha-Particles:
Glycogen molecules form beta-particles, which consist of dimers and trimers linked to a central glycogenin backbone. These beta-particles cluster to form larger structures called alpha-particles, measuring 100–150 nm in diameter. - Protein Association:
Glycogen is closely associated with proteins, with the glycogen granules often bound to enzymes involved in glycogen metabolism. In tissues, glycogen can be adsorbed to proteins, forming complexes known as glycosomes. These glycosomes can exist as lyoglicosomes (acid-labile, free in the cytosol) or desmoglycosomes (acid-resistant, associated with cellular structures like the endoplasmic reticulum). - Hydration and Osmotic Properties:
Due to its structure and extensive branching, glycogen is osmotically inactive. This allows it to be stored in large quantities within cells without disrupting cellular osmotic balance.
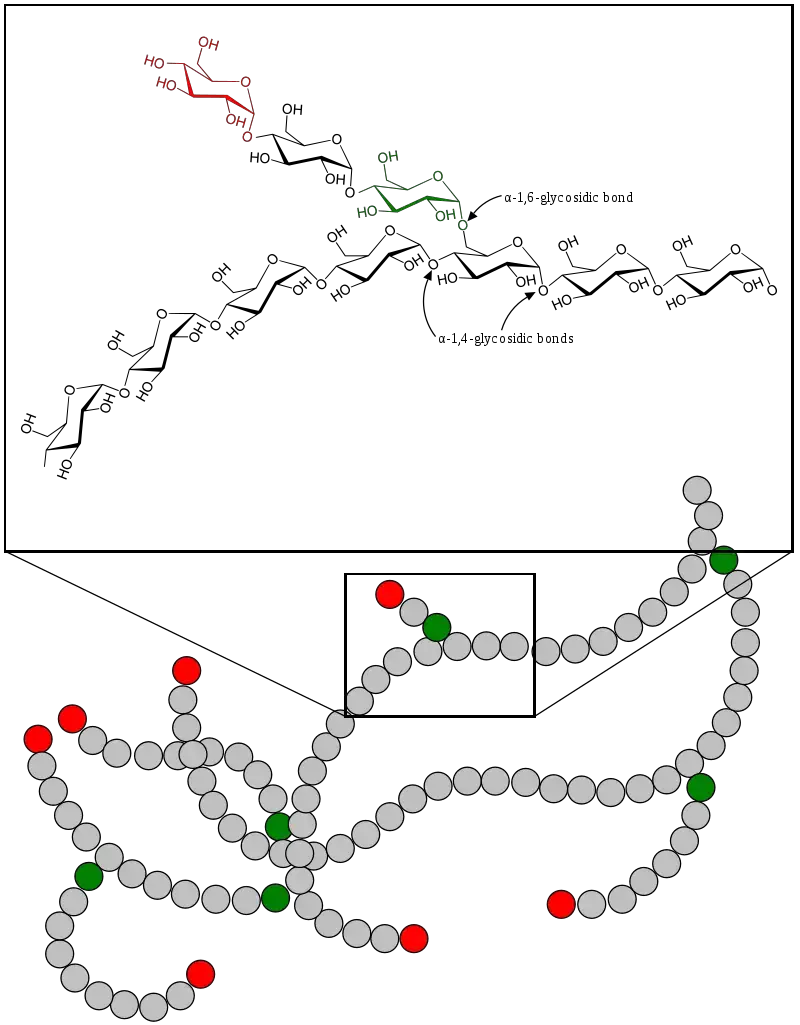
Location of Glycogen
Glycogen is stored in several key locations within the body, with each serving distinct roles to meet the body’s energy demands. Its distribution ensures that energy is readily available for both immediate and long-term needs, depending on the tissue and metabolic state.
- Skeletal Muscles:
- About 80% of the body’s total glycogen is stored in skeletal muscles, roughly 500 grams in an average adult.
- This glycogen is crucial for local energy production during physical activity.
- Unlike liver glycogen, muscle glycogen is not released into the bloodstream. It is used directly by muscle cells to fuel contractions during exercise.
- Liver:
- The liver stores around 100 grams of glycogen, which is approximately 5-6% of its fresh weight.
- Liver glycogen plays a central role in maintaining blood glucose levels.
- During periods of fasting or between meals, the liver breaks down glycogen into glucose and releases it into the bloodstream, providing a steady energy supply for other tissues, especially the brain.
- Other Tissues:
- Brain: The brain contains a much smaller amount of glycogen compared to muscles and the liver, about 100 times less. However, this glycogen is important for supporting neuronal function when blood glucose levels are low.
- Heart: The heart muscle stores glycogen to meet its energy needs during periods of high activity.
- Kidneys and Blood Cells: Minor amounts of glycogen are stored in kidney cells and both red and white blood cells, contributing to their energy requirements.
- Glycogen Pools in Yeast:
- In yeast cells, glycogen is found in two forms.
- One is water-insoluble or acid-extractable, located outside the cellular membrane, which is the major pool of glycogen in yeast.
- The second pool is water-soluble or alkali-extractable, found inside the cellular membrane.
- The roles of these pools in yeast metabolism are still under investigation, and their distinct functions have not yet been fully established.
The amount of glycogen stored in the body can vary, influenced by factors such as diet, exercise, and metabolic conditions. After carbohydrate intake, insulin stimulates glycogenesis (the formation of glycogen), while glycogenolysis (the breakdown of glycogen) occurs when the body requires more glucose for energy.
Glycogen Metabolism
Glycogen metabolism is a crucial process for maintaining energy balance in the body. It involves the synthesis and breakdown of glycogen, which serves as a readily available energy store. The two primary processes in glycogen metabolism are glycogenesis (synthesis of glycogen) and glycogenolysis (breakdown of glycogen).
Glycogenesis (Glycogen Synthesis)
- Glycogenesis requires uridine triphosphate (UTP) to supply energy.
- The first step involves hexokinase or glucokinase phosphorylating glucose, forming glucose-6-phosphate.
- Phosphoglucomutase then converts glucose-6-phosphate to glucose-1-phosphate.
- The enzyme UTP-glucose-1-phosphate uridylyltransferase catalyzes the activation of glucose, forming UDP-glucose from UTP and glucose-1-phosphate.
- In the initial phase of glycogen synthesis, the protein glycogenin catalyzes the attachment of UDP-glucose to itself. It acts as a primer for further glycogen synthesis by adding glucose molecules.
- Glycogen synthase then adds glucose units to the growing chain through α-1,4 glycosidic linkages.
- Branching occurs through the enzyme amylo-(1,4 to 1,6)-transglucosidase (glycogen branching enzyme), which transfers a segment of the glucose chain to form α-1,6 linkages, creating branches in the glycogen molecule.
Glycogenolysis (Glycogen Breakdown)
- Glycogen phosphorylase is the key enzyme that breaks down glycogen by cleaving glucose-1-phosphate from the non-reducing end of the glycogen chain.
- Phosphoglucomutase converts glucose-1-phosphate to glucose-6-phosphate for further metabolic processes.
- Glycogen phosphorylase cannot cleave glucose at the branch points of glycogen; glycogen debranching enzyme (GDE) is required to handle these points. GDE performs two functions:
- Glucotransferase activity: It moves a segment of the branch to another branch, creating more accessible non-reducing ends.
- Glucosidase activity: It removes the final α-1,6-linked glucose at the branch point.
- After debranching, the released glucose is not phosphorylated in muscle, unlike in the liver, where glucose-6-phosphatase is present to convert glucose-6-phosphate into free glucose for release into the bloodstream.
- In muscle tissue, since glucose-6-phosphatase is absent, glucose-6-phosphate enters glycolysis for ATP production and energy. It can also enter the pentose phosphate pathway to produce NADPH and five-carbon sugars.
Key Enzymes Involved in Glycogen Metabolism
- Glycogen Synthase: Responsible for elongating the glycogen chain by adding glucose units via α-1,4 glycosidic bonds.
- Amylo-(1,4 to 1,6)-transglucosidase (Glycogen Branching Enzyme): Responsible for creating branches in the glycogen molecule via α-1,6 linkages.
- Glycogen Phosphorylase: Cleaves glucose-1-phosphate units from glycogen during glycogenolysis.
- Debranching Enzyme (GDE): Handles glycogen branches and removes the final glucose unit at the branch point.
- Phosphoglucomutase: Converts glucose-1-phosphate to glucose-6-phosphate.
Differences in Glycogen Metabolism Between Tissues
- Liver: Acts as a major regulator of blood glucose levels. The liver stores glycogen and releases glucose into the bloodstream when blood glucose levels are low. The enzyme glucose-6-phosphatase enables the liver to convert glucose-6-phosphate into free glucose, which is then released.
- Muscle: Glycogen is stored as an energy reserve for muscle contractions during exercise. However, muscle cells cannot release glucose into the bloodstream because they lack glucose-6-phosphatase. Instead, glycogen breakdown in muscle leads to the production of glucose-6-phosphate, which is used directly for energy production in glycolysis.
Glycogen Synthesis
Glycogen synthesis is a multi-step biochemical process where glucose molecules are converted into glycogen for storage in the liver and muscles. This process is essential for maintaining energy reserves, particularly during periods of fasting or increased energy demand.
- Glucose Uptake:
- Glucose enters cells via glucose transporters (GLUTs).
- In muscle and fat tissues, GLUT4 is activated by insulin to facilitate glucose uptake.
- GLUT1 is responsible for glucose transport in the brain and red blood cells.
- Phosphorylation of Glucose:
- Once inside the cell, hexokinase phosphorylates glucose to form glucose 6-phosphate (G6P).
- This phosphorylation step is critical for trapping glucose inside the cell, preventing it from leaving and preparing it for further processing.
- Isomerization:
- Phosphoglucomutase converts glucose 6-phosphate into glucose 1-phosphate (G1P).
- This conversion is necessary for the subsequent steps in glycogen synthesis.
- Formation of UDP-Glucose:
- UDP-glucose pyrophosphorylase converts glucose 1-phosphate into uridine diphosphate-glucose (UDP-glucose).
- UDP-glucose is the activated form of glucose, serving as the direct donor of glucose units in the formation of glycogen.
- Initiation of Glycogen Synthesis:
- Glycogenin, a primer for glycogen synthesis, catalyzes its own glycosylation by adding 10–20 glucose units from UDP-glucose, forming a short glucan chain.
- This glucan chain acts as the foundation for further glycogen elongation.
- Elongation of Glycogen Chains:
- Glycogen synthase extends the glycogen chain by transferring additional glucose units from UDP-glucose to the growing chain.
- The enzyme forms α-1,4-glycosidic bonds, linking glucose molecules in a linear fashion, crucial for building the glycogen structure.
- Branching of Glycogen:
- The branching enzyme introduces α-1,6-glycosidic bonds at intervals along the glycogen molecule, creating a branched structure.
- This branching increases glycogen’s solubility and provides more ends for rapid mobilization during energy demand.
- Regulation and Coordination:
- The activity of glycogen synthase is tightly regulated by various factors.
- Allosteric effectors like glucose 6-phosphate enhance its activity, while phosphorylation by glycogen synthase kinases and dephosphorylation by protein phosphatases can modify its function, ensuring the process is finely tuned to the cell’s metabolic state.
Glycogen Degradation
Glycogen degradation, or glycogenolysis, is the breakdown of glycogen into glucose, a process crucial for supplying energy in muscle and liver cells. This breakdown is tightly regulated to meet energy demands.
- Activation of Glycogen Phosphorylase:
- The enzyme glycogen phosphorylase is activated through phosphorylation.
- Hormones like glucagon in the liver and epinephrine in muscles trigger this activation, which enables the enzyme to begin the breakdown of glycogen by releasing glucose 1-phosphate (G1P) from the non-reducing ends of glycogen chains.
- Release of Glucose 1-Phosphate:
- During this process, glycogen phosphorylase adds inorganic phosphate (Pi) to the terminal glucose residue of glycogen, generating glucose 1-phosphate.
- This phosphorolysis continues until the enzyme encounters a branch point within the glycogen structure.
- Debranching of Glycogen:
- At branch points, where α-1,6-glycosidic bonds are located, glycogen phosphorylase can no longer continue its cleavage.
- Glycogen debranching enzyme comes into play, with two critical activities:
- Transferase activity: It shifts a block of three glucose residues from one branch to another, making the chain accessible for further cleavage.
- Glucosidase activity: It hydrolyzes the final glucose residue at the branch point, releasing free glucose.
- Conversion of Glucose 1-Phosphate to Glucose 6-Phosphate:
- The enzyme phosphoglucomutase converts glucose 1-phosphate into glucose 6-phosphate (G6P).
- This step is essential because G6P can either enter glycolysis for energy production or be prepared for conversion into free glucose.
- Dephosphorylation of Glucose 6-Phosphate:
- In the liver, glucose 6-phosphatase dephosphorylates G6P to form free glucose, which can be released into the bloodstream, helping to regulate blood sugar during fasting.
- In muscle cells, G6P typically enters glycolysis for immediate energy use, rather than being converted back to free glucose.
Regulation of Glycogen
Glycogen regulation ensures glucose homeostasis in the body by switching between synthesis and breakdown based on energy needs. This process is tightly controlled by hormones like insulin and glucagon, which regulate anabolic and catabolic pathways respectively. Their effects on enzymes and metabolic pathways help balance the storage and release of glucose.
Hormonal Regulation of Glycogen Metabolism
- Insulin and Glucagon:
- Insulin signals a high-energy state, promoting glycogen and lipid synthesis.
- Glucagon signals a low-energy state, driving glycogenolysis (glycogen breakdown) and lipolysis.
- These hormones trigger opposite effects through distinct signaling pathways that alter enzyme activity.
- Insulin’s Effects:
- Activation of Protein Phosphatase 1 (PP1): Insulin activates PP1, which dephosphorylates enzymes involved in glycogen synthesis, promoting the active form of glycogen synthase.
- Activation of Protein Kinase B (PKB): PKB maintains glycogen synthase activity by inhibiting glycogen synthase kinase 3 (GSK3), an enzyme that would otherwise deactivate glycogen synthase.
- Glucagon’s Effects:
- Activation of Protein Kinase A (PKA): Glucagon activates PKA through a cAMP-mediated pathway. PKA phosphorylates and inactivates PP1, preventing the conversion of glycogen synthase b (inactive form) to glycogen synthase a (active form).
- Glycogenolysis Activation: PKA also activates glycogen phosphorylase kinase, which then activates glycogen phosphorylase to break down glycogen.
Enzyme Regulation in Glycogen Synthesis and Breakdown
- Glycogen Synthase:
- Two Forms: Glycogen synthase exists in two forms—glycogen synthase a (active) and glycogen synthase b (inactive). The difference lies in the phosphorylation state, with glycogen synthase b being more phosphorylated.
- Insulin and PP1 Action: When insulin is present, PP1 dephosphorylates glycogen synthase b, converting it to the active glycogen synthase a.
- PKB and GSK3: PKB inactivates GSK3, preventing it from phosphorylating glycogen synthase a back to its inactive form.
- Glycogen Phosphorylase:
- Two Forms: Glycogen phosphorylase also has two forms—glycogen phosphorylase a (active) and glycogen phosphorylase b (inactive).
- Activation by PKA: PKA activates glycogen phosphorylase through glycogen phosphorylase kinase, which phosphorylates glycogen phosphorylase.
- Deactivation by PP1: PP1 dephosphorylates glycogen phosphorylase, converting it to its inactive form, glycogen phosphorylase b.
- Liver Isoform as a “Glucose Sensor”: In the liver, glycogen phosphorylase requires glucose to bind to an allosteric site for PP1 to dephosphorylate it, acting as a glucose sensor to regulate glycogen breakdown.
Skeletal Muscle-Specific Regulation
- Muscle Glycogen Phosphorylase Activation:
- In skeletal muscle, calcium released during muscle contraction binds to calmodulin, activating glycogen phosphorylase kinase.
- Allosteric Regulators: Low energy signals like AMP, IMP, and Pi activate skeletal muscle glycogen phosphorylase, ensuring glucose is available during muscle activity.
Glycogen Metabolism in the Liver
Glycogen metabolism in the liver plays a crucial role in maintaining blood glucose levels and providing energy when needed. The liver is responsible for storing glycogen and releasing glucose into the bloodstream, depending on the body’s energy requirements.
- Glycogen Synthesis After Food Intake:
- Liver glycogen primarily restores itself during the postprandial period in healthy individuals.
- The glucose that forms glycogen in the liver comes either from the direct pathway (derived from ingested food) or through gluconeogenesis, an indirect pathway where precursors like lactate and alanine are converted into glucose 6-phosphate.
- Gluconeogenesis vs Direct Glycogen Synthesis:
- In patients with congenital glucokinase deficiency, the liver’s ability to phosphorylate glucose to glucose 6-phosphate is impaired. As a result, the indirect pathway (gluconeogenesis) takes precedence over the direct pathway for glycogen synthesis after food ingestion. This compensates for the inability to utilize food-derived glucose properly.
- Role of Other Monosaccharides:
- The contribution of monosaccharides like fructose and galactose to hepatic glycogen synthesis remains uncertain.
- Galactose has been estimated to account for about 19% of liver glycogen synthesis in healthy individuals, though its role is not fully defined.
- Some studies suggest that fructose or galactose supplementation is more effective than glucose for restoring liver glycogen, especially after exercise in trained individuals.
Glycogen Metabolism in Skeletal Muscle
Glycogen plays a critical role in skeletal muscle energy metabolism, especially during physical activity. As the main storage form of glucose in the body, glycogen is stored in muscles and liver to provide energy during muscle contraction. In skeletal muscle, glycogen serves as a vital energy reservoir, which is rapidly depleted during intense exercise.
- Exercise Reduces Muscle Glycogen
When muscles contract during exercise, glycogen is broken down to release glucose, which is then used for energy. The amount of glycogen in active skeletal muscle significantly drops after high-intensity exercise. Biopsies from healthy individuals show that glycogen levels are reduced in the muscles engaged in activity, while muscles not involved in exercise maintain their glycogen stores. Once muscle glycogen stores are exhausted, fatigue sets in, signaling the end of energy supply for continued contraction.
Interestingly, glycogen depletion varies based on exercise intensity, and supplementation strategies such as fructose intake have been shown to help spare glycogen during physical exertion. - Training Enhances Glycogen Storage
Regular exercise and training improve the skeletal muscle’s ability to store glycogen. After initial exercise, muscle glycogen levels rise during the recovery period and exceed baseline levels. This capacity for increased storage is enhanced over time with consistent training, contributing to better endurance. In endurance-trained individuals, skeletal muscles store more glycogen than in untrained individuals. The process of glycogen synthesis requires the breakdown of glycogen, a crucial mechanism that aids in the muscle’s ability to restore glycogen after depletion. - Nutritional Influences on Glycogen Storage
Diet alone does not significantly alter glycogen storage in resting muscles. Although high-carbohydrate meals can slightly increase muscle glycogen content, the effect is most pronounced in muscles that have recently been used in physical activity. In untrained individuals, the consumption of large amounts of carbohydrates does not result in a substantial increase in glycogen storage. Skeletal muscle biopsies indicate that the glycogen levels in inactive muscles show minimal changes after high or low carbohydrate diets, further highlighting that exercise, rather than diet alone, is the primary driver of glycogen replenishment. - Exercise and Glycogen Storage Diseases
Glycogen storage diseases (GSDs) can impact the skeletal muscle’s ability to metabolize and store glycogen. Many GSDs, such as McArdle’s disease, involve deficiencies in enzymes critical to glycogen metabolism, like glycogen phosphorylase. Patients with GSDs often experience exercise intolerance and muscle damage, including cramps and rhabdomyolysis. Despite this, exercise programs that emphasize light intensity activity may improve muscle function in some GSD patients by shifting muscle metabolism toward increased fatty acid oxidation. For patients with McArdle disease, regular exercise may help reduce reliance on glycogen during muscle contractions. - Impact of Glycogen on Muscle Function
Glycogen’s role extends beyond energy production. Its synthesis and breakdown are tightly regulated processes that are crucial for skeletal muscle performance. Disorders that impair glycogen metabolism can lead to fasting hypoglycemia, exercise intolerance, or both. Enzyme deficiencies in GSDs cause a range of symptoms, and understanding glycogen’s metabolic pathways is crucial for the development of targeted treatments. Additionally, glycogen’s interaction with fatty acid metabolism in skeletal muscle is an important consideration in both healthy individuals and patients with metabolic disorders. - Research and Treatment Implications
The growing understanding of glycogen metabolism has the potential for improving treatment strategies for GSDs. Enzyme replacement therapy exists for some conditions, but its effectiveness is not universal. Advances in gene therapy, including nonviral gene vectors, hold promise for future treatment options. Understanding the genetic and biochemical aspects of glycogen metabolism remains essential for developing new therapies and improving patient outcomes in those affected by glycogen storage diseases.
Clinical Significance of Glycogen
Glycogen is crucial for maintaining glucose levels in the body, but its regulation can be disrupted by various genetic conditions. Glycogen storage diseases (GSDs) arise from mutations in enzymes that control glycogen synthesis or breakdown. These disorders often affect children and are typically inherited in an autosomal recessive pattern. Here’s a breakdown of the major GSDs and the enzymes involved:
Major Glycogen Storage Diseases
- Type 0 GSD: Caused by mutations in muscle glycogen synthase (GYS1) or liver glycogen synthase (GYS2). These enzymes are essential for the synthesis of glycogen in muscle and liver tissue, respectively. The absence of these enzymes leads to impaired glycogen storage.
- Type Ia (Von Gierke Disease): Results from mutations in glycogen-6-phosphatase (G6PC), which is responsible for removing the phosphate group from glucose-6-phosphate (G6P). Without this enzyme, glucose cannot be released from the liver, causing severe hypoglycemia and other metabolic issues.
- Type 1b (Von Gierke Disease): Involves mutations in glycogen-6-phosphatase transporter (G6PT), which is responsible for transporting G6P into the endoplasmic reticulum. This defect also prevents the release of glucose from the liver, leading to similar symptoms as Type Ia GSD.
- Type II (Pompe Disease): Caused by mutations in acid alpha-glucosidase (GAA), an enzyme located in the lysosome. This enzyme is responsible for breaking down glycogen within the lysosome. Its deficiency leads to the accumulation of glycogen in various tissues, particularly in the muscles, causing severe muscle weakness.
- Type III (Cori Disease): Results from defects in the glycogen debranching enzyme. This enzyme is needed to remove branching points in glycogen. A defect leads to abnormal glycogen storage, causing liver and muscle problems.
- Type IV (Andersen Disease): Caused by mutations in the glycogen branching enzyme, which is necessary for proper glycogen structure. The absence of this enzyme results in an abnormal, less soluble glycogen form, which accumulates in tissues and causes liver failure.
- Type V (McArdle Disease): Occurs due to mutations in muscle and heart glycogen phosphorylase. This enzyme is involved in glycogen breakdown. Without it, muscles cannot break down glycogen into glucose during exercise, leading to muscle cramps and weakness.
- Type VI (Hers Disease): Caused by mutations in liver glycogen phosphorylase. This enzyme is key for glycogen breakdown in the liver. Its deficiency leads to hypoglycemia, particularly during periods of fasting.
Implications of Glycogen Storage Diseases
- Early Presentation: Most GSDs present early in life, often in infancy or childhood, with symptoms such as hypoglycemia, muscle weakness, and liver enlargement.
- Systemic Impact: These diseases impact organs that store glycogen, primarily the liver and muscles, and can affect overall metabolic function.
- Management: Treatment strategies often involve dietary management, such as frequent feedings of carbohydrates to maintain glucose levels or the use of glucose infusion during periods of fasting.
Function of Glycogen
Glycogen plays a pivotal role in energy metabolism, acting as a readily accessible source of glucose for various cells and tissues in the body. Its function is tightly regulated, ensuring that glucose is available when needed and stored when in excess.
- In Liver Cells:
- Glycogen serves as the primary buffer for blood glucose levels.
- When blood glucose is high, insulin promotes the uptake of glucose into liver cells, triggering the synthesis of glycogen through glyconeogenesis.
- As glucose and insulin levels drop, glycogen synthesis halts.
- When blood glucose decreases, glucagon signals liver cells to break down glycogen into glucose-1-phosphate, which is then converted into glucose and released into the bloodstream.
- The breakdown of glycogen in the liver is crucial for maintaining blood glucose levels during fasting or between meals.
- In Muscle Cells:
- Muscle stores glycogen in smaller amounts compared to the liver, making up about 1-2% of muscle mass. However, the total glycogen stored in muscle is greater due to the overall mass of muscle tissue.
- Unlike liver cells, muscle glycogen is not released into the bloodstream. Instead, it is utilized locally to provide energy for muscle contractions during physical activity.
- When muscle glycogen is broken down, it is converted into glucose-6-phosphate, which is used in glycolysis to produce ATP for energy.
- Muscle glycogen is essential during exercise and periods of physical stress (e.g., fight-or-flight response).
- In Other Tissues:
- Smaller amounts of glycogen are found in various tissues such as red blood cells, white blood cells, kidney cells, and some glial cells.
- In red blood cells, glycogen supports energy needs, as these cells rely on anaerobic metabolism.
- In the uterus, glycogen serves to provide energy for the embryo.
- In Microorganisms (Fungi and Bacteria):
- Glycogen is also an important energy reserve for microorganisms, such as Saccharomyces cerevisiae (baker’s yeast).
- Yeast synthesizes glycogen when nutrient levels are low, particularly when carbon or other nutrients are scarce.
- Bacteria store glycogen as a way to cope with periods of nutrient limitation.
- Glycogen accumulation in yeast is particularly relevant during sporulation and the response to stress, which can induce glycogen recycling to maintain cell function and avoid overproduction of ATP.
- Regulation and Metabolism:
- The body’s glycogen metabolism is tightly linked to glucose metabolism.
- In situations of low energy availability, glycogen reserves in the liver and muscles are mobilized to provide glucose.
- Fat is the primary energy source in the body, but during prolonged starvation, glycogen becomes critical as the liver works to release glucose into the bloodstream.
- As glycogen stores become depleted after 6-12 hours, the liver increases gluconeogenesis to continue producing glucose.
- The regulation of glycogen metabolism ensures that energy is available for both short-term and prolonged needs.
Quiz
What is the primary role of glycogen in the human body?
a) DNA synthesis
b) Protein synthesis
c) Energy storage
d) Lipid transport
Glycogen is primarily stored in which two organs of the human body?
a) Kidneys and Lungs
b) Liver and Heart
c) Liver and Skeletal Muscles
d) Brain and Kidneys
Which enzyme is responsible for the synthesis of glycogen?
a) Glycogen synthase
b) Glycogen phosphorylase
c) Hexokinase
d) Glucokinase
The branching points in the glycogen molecule are formed by which type of glycosidic bond?
a) α-1,2
b) α-1,4
c) α-1,6
d) β-1,4
Which disease is caused by a deficiency in the enzyme lysosomal acid α-glucosidase?
a) McArdle Disease
b) Andersen Disease
c) Pompe Disease
d) Cori Disease
Which enzyme is responsible for the breakdown of glycogen to release glucose-1-phosphate?
a) Glucokinase
b) Glycogen synthase
c) Glycogen phosphorylase
d) Hexokinase
Glycogen has a structure most similar to which plant polysaccharide?
a) Cellulose
b) Chitin
c) Amylose
d) Amylopectin
Which hormone stimulates the breakdown of glycogen in the liver?
a) Insulin
b) Glucagon
c) Adrenaline
d) Cortisol
In which cellular location is glycogen primarily found?
a) Mitochondria
b) Nucleus
c) Cytosol
d) Endoplasmic reticulum
Which of the following is NOT a characteristic of glycogen?
a) It is a branched polymer.
b) It serves as a primary energy storage molecule in plants.
c) It contains α-1,4 and α-1,6 glycosidic linkages.
d) It is stored in the liver and muscles.
FAQ
What is glycogen?
Glycogen is a branched polysaccharide that serves as the primary storage form of glucose in animals and humans.
Where is glycogen primarily stored in the body?
Glycogen is primarily stored in the liver and skeletal muscles.
How is glycogen different from starch?
While both glycogen and starch are glucose polymers, glycogen is more extensively branched and compact than starch. Starch is the primary form of glucose storage in plants, whereas glycogen is the primary form in animals.
What is the role of glycogen in energy metabolism?
Glycogen acts as an energy reservoir. When the body requires energy, glycogen is broken down to release glucose, which is then used to produce ATP, the body’s primary energy molecule.
How is glycogen synthesized in the body?
Glycogen synthesis, known as glycogenesis, involves the addition of glucose molecules to a growing glycogen chain, primarily facilitated by the enzyme glycogen synthase.
What happens to glycogen during prolonged exercise?
During prolonged exercise, the body depletes its glycogen stores to produce energy. Once glycogen stores are exhausted, the body experiences fatigue and relies more on fat as an energy source.
What are glycogen storage diseases?
Glycogen storage diseases are a group of inherited metabolic disorders resulting from enzyme deficiencies affecting the synthesis or degradation of glycogen. Examples include Pompe Disease, McArdle Disease, and Andersen Disease.
How does the body regulate glycogen synthesis and breakdown?
The body regulates glycogen metabolism through hormones like insulin (promotes glycogen synthesis) and glucagon (stimulates glycogen breakdown).
Why is glycogen referred to as “animal starch”?
Glycogen is sometimes called “animal starch” because of its structural and functional similarities to starch, the glucose storage molecule in plants.
Can the body convert fats and proteins to glycogen?
While the body primarily uses glucose to make glycogen, in certain situations, it can convert the glycerol portion of fats and some amino acids from proteins into glucose, which can then be stored as glycogen.
- Young, F. G. (1957). “Claude Bernard and the Discovery of Glycogen”. British Medical Journal. 1(5033 (Jun. 22, 1957)): 1431–7. doi:10.1136/bmj.1.5033.1431 ://www.bmj.com/content/1/5033/1431 Link
Berg, J. M., Tymoczko, J. L., & Lubert Stryer. (2002, January 1). Glycogen Metabolism. Retrieved from ://www.ncbi.nlm.nih.gov/books/NBK21190/ Link - Adeva-Andany, M. M., González-Lucán, M., Donapetry-García, C., Fernández-Fernández, C., & Ameneiros-Rodríguez, E. (2016). Glycogen metabolism in humans. BBA Clinical, 5, 85–100. ://doi.org/10.1016/j.bbacli.2016.02.001 Link
- Physiologic Effects of Insulin. (2019, January 1). Retrieved from ://www.vivo.colostate.edu/hbooks/pathphys/endocrine/pancreas/insulin-phys.html Link
- Eicke, S., Seung, D., Egli, B., Devers, E.A., and Streb, S. (2017) “Increasing the carbohydrate storage capacity of plants by engineering a glycogen-like polymer pool in the cytosol.” Metabolic Engineering. 40:23-32.
- Rocha Leão, M. H. M. (2003). GLYCOGEN. Encyclopedia of Food Sciences and Nutrition, 2930–2937. doi:10.1016/b0-12-227055-x/00563-0
- Hargreaves, M. and Richter, E.A. (1988) “Regulation of skeletal muscle glycogenolysis during exercise.” Canadian Journal of Sport Sciences. 13(4):197-203.
Ivy, J.L. (1991). “Muscle glycogen synthesis before and after exercise.” Sports Medicine. 11(1):6-19. - Daghlas SA, Mohiuddin SS. Biochemistry, Glycogen. [Updated 2023 May 1]. In: StatPearls [Internet]. Treasure Island (FL): StatPearls Publishing; 2024 Jan-. Available from: https://www.ncbi.nlm.nih.gov/books/NBK539802/
- https://en.wikipedia.org/wiki/Glycogen