What is Gluconeogenesis?
- Gluconeogenesis is a metabolic process that synthesizes glucose from non-carbohydrate precursors. This mechanism is essential during fasting or periods of limited dietary carbohydrate intake, ensuring the maintenance of blood glucose levels required for energy production in vital organs, especially the brain and red blood cells.
- The process primarily occurs in the liver and, to a lesser extent, in the kidneys. It utilizes substrates such as lactate, pyruvate, glycerol, and glucogenic amino acids, derived from protein breakdown, lipid metabolism, and the Cori cycle. In some conditions, such as prolonged fasting, acetone from ketone bodies can also contribute to glucose synthesis.
- The reactions of gluconeogenesis take place in both the cytosol and mitochondria of cells. This pathway functions opposite to glycolysis, reversing the conversion of glucose into pyruvate. However, certain steps of glycolysis are bypassed by unique, enzyme-catalyzed reactions in gluconeogenesis to overcome the energetically unfavorable steps.
- The pathway demands significant energy input, consuming four molecules of ATP and two molecules of GTP to convert pyruvate into glucose-6-phosphate. This energy is primarily supplied by beta-oxidation of fatty acids. Thus, gluconeogenesis becomes particularly critical during states of energy deficit, such as fasting or intense physical activity.
- Hormonal regulation plays a pivotal role in controlling the rate of gluconeogenesis. Insulin inhibits this pathway, while glucagon and cortisol stimulate it, adjusting glucose production based on the body’s metabolic needs. Dysregulation of gluconeogenesis is a hallmark of metabolic disorders like diabetes, where excessive glucose production exacerbates hyperglycemia.
- Overall, gluconeogenesis is an indispensable metabolic process that ensures glucose availability when carbohydrate intake is insufficient. It underscores the body’s ability to adapt and maintain metabolic balance during varying physiological conditions.
Gluconeogenesis Definition
Gluconeogenesis is a metabolic process that synthesizes glucose from non-carbohydrate precursors, such as lactate, glycerol, and amino acids, primarily in the liver and kidneys, to maintain blood glucose levels during fasting or low carbohydrate intake.
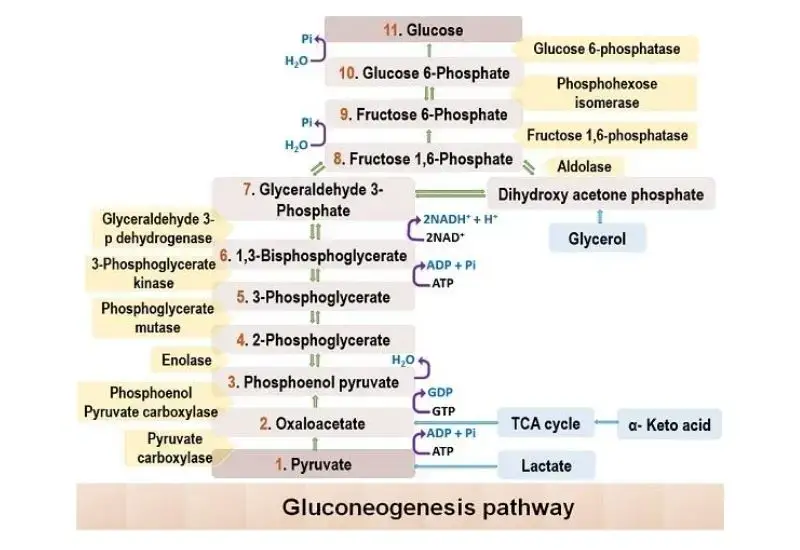
Location of Gluconeogenesis – Where does Gluconeogenesis Occur?
Gluconeogenesis primarily occurs in the liver, which serves as the main site for glucose synthesis and its release into the bloodstream. The liver possesses the necessary enzymes, such as pyruvate carboxylase and glucose-6-phosphatase, that drive the pathway, enabling it to efficiently produce glucose from non-carbohydrate sources like lactate, glycerol, and amino acids.
The kidneys are a secondary site for gluconeogenesis, contributing less to glucose production under normal conditions. However, their role becomes more prominent during prolonged fasting, starvation, or intense physical activity, when the demand for glucose increases. The renal cortex is specifically involved in this process, as it contains the metabolic machinery required for gluconeogenesis.
Together, the liver and kidneys ensure a stable glucose supply, particularly during periods of low carbohydrate availability. This process is vital for maintaining blood glucose levels and providing energy to glucose-dependent tissues such as the brain and red blood cells.
Gluconeogenesis Pathway Steps
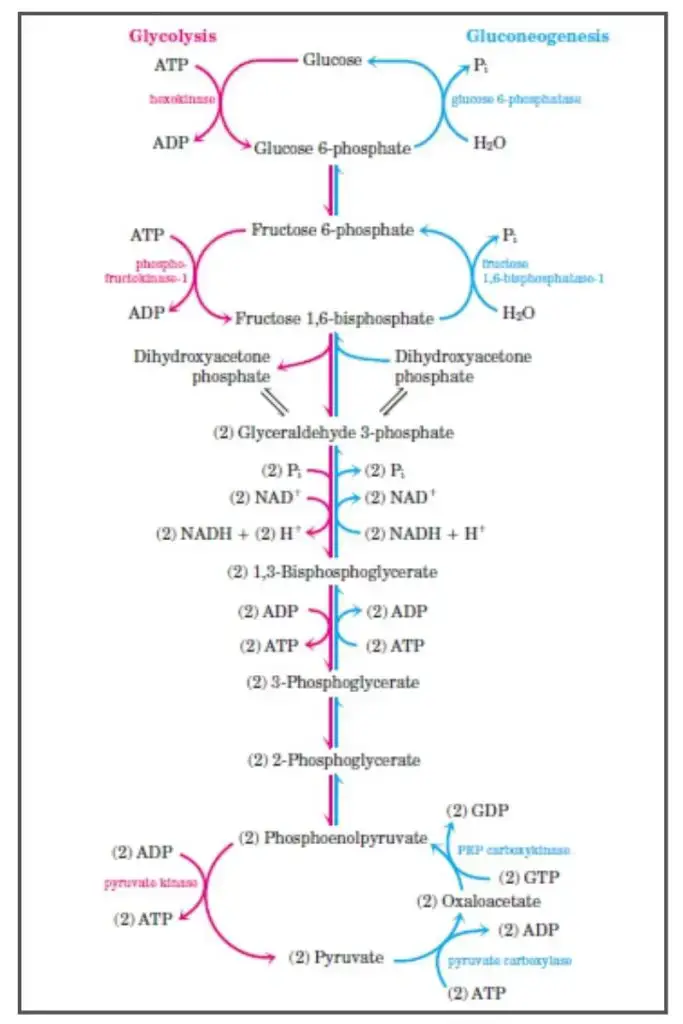
Gluconeogenesis is the metabolic pathway that generates glucose from non-carbohydrate precursors. The process is essentially the reverse of glycolysis, but several key enzymes replace those involved in glycolysis, making the pathway unidirectional where necessary. The mechanism of gluconeogenesis involves several stages, beginning with the conversion of pyruvate to glucose.
- Pyruvate to Oxaloacetate:
- The first step in gluconeogenesis is the carboxylation of pyruvate to oxaloacetate, a reaction catalyzed by the enzyme pyruvate carboxylase (PC).
- Pyruvate carboxylase uses ATP and biotin as a cofactor and requires CO2 as a carbon source.
- The reaction occurs in the mitochondria, and oxaloacetate is then shuttled to the cytosol through the malate shuttle. This shuttle involves the reduction of oxaloacetate to malate, which can cross the mitochondrial membrane, and then its reoxidation back to oxaloacetate in the cytosol.
- Oxaloacetate to Phosphoenolpyruvate (PEP):
- In the cytosol, oxaloacetate is converted into phosphoenolpyruvate (PEP) by the enzyme PEP carboxykinase (PEPCK).
- This reaction uses GTP as the phosphate donor. PEPCK is induced by glucocorticoids like cortisol, which regulate gene expression for this enzyme.
- Reversible Steps in the Cytosol:
- After the formation of PEP, a series of reversible reactions, common to both gluconeogenesis and glycolysis, proceed.
- Enolase catalyzes the conversion of PEP to 2-phosphoglycerate.
- Phosphoglycerate mutase converts 2-phosphoglycerate into 3-phosphoglycerate.
- Phosphoglycerate kinase uses ATP to convert 3-phosphoglycerate to 1,3-bisphosphoglycerate.
- Glyceraldehyde-3-phosphate dehydrogenase reduces 1,3-bisphosphoglycerate to glyceraldehyde-3-phosphate, with the oxidation of NADH.
- Triose Phosphate Interconversion:
- Glyceraldehyde-3-phosphate can be converted into dihydroxyacetone phosphate (DHAP) by triosephosphate isomerase.
- Aldolase A then catalyzes the conversion of glyceraldehyde-3-phosphate into fructose-1,6-bisphosphate, preparing the pathway for the rate-limiting step.
- Fructose-1,6-bisphosphate to Fructose-6-phosphate:
- The conversion of fructose-1,6-bisphosphate to fructose-6-phosphate is an irreversible step, making it a critical point of regulation in gluconeogenesis.
- The enzyme fructose-1,6-bisphosphatase catalyzes this reaction and is activated by ATP and citrate, which signal the cell’s energy status.
- Conversely, AMP and fructose-2,6-bisphosphate (F2,6BP) inhibit this enzyme, signaling low energy availability.
- Glucagon activates protein kinase A (PKA) through cAMP, leading to the phosphorylation and inactivation of phosphofructokinase-2 (PFK-2), thereby reducing F2,6BP levels, which decreases the inhibition on fructose-1,6-bisphosphatase.
- Final Step – Glucose Production:
- The final irreversible step in gluconeogenesis is the conversion of glucose-6-phosphate to glucose by glucose-6-phosphatase.
- This enzyme is primarily found in the liver, kidneys, and intestinal epithelium, and the reaction occurs in the endoplasmic reticulum.
- Muscle cells do not have this enzyme and thus cannot release glucose into the bloodstream, relying on glucose for their own energy needs instead.
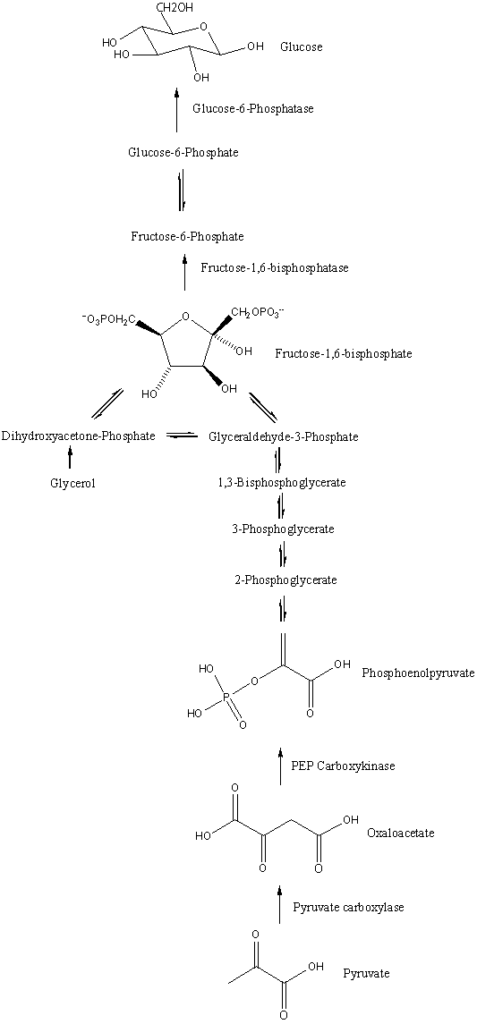
Gluconeogenesis Reactions
This process involves a series of enzyme-catalyzed reactions that reverse the steps of glycolysis, with a few key exceptions at the irreversible steps. Below is a detailed breakdown of these critical reactions.
- Conversion of Pyruvate to Oxaloacetate:
- Enzyme: Pyruvate Carboxylase
- Reaction: Pyruvate + ATP + CO₂ → Oxaloacetate + ADP + Pi
- Location: Mitochondria
- Notes: This reaction is essential for the initiation of gluconeogenesis. It requires biotin as a cofactor and is activated by acetyl-CoA, signaling an abundance of energy for glucose production.
- Conversion of Oxaloacetate to Phosphoenolpyruvate (PEP):
- Enzyme: Phosphoenolpyruvate Carboxykinase (PEPCK)
- Reaction: Oxaloacetate + GTP → PEP + GDP + CO₂
- Location: Mitochondria and cytosol
- Notes: This step involves both decarboxylation and phosphorylation, where GTP acts as the phosphate donor to convert oxaloacetate into phosphoenolpyruvate (PEP).
- Conversion of PEP to 2-Phosphoglycerate:
- Enzyme: Enolase (reversible reaction)
- Reaction: PEP → 2-Phosphoglycerate
- Conversion of 2-Phosphoglycerate to 3-Phosphoglycerate:
- Enzyme: Phosphoglycerate Mutase
- Reaction: 2-Phosphoglycerate ↔ 3-Phosphoglycerate
- Conversion of 3-Phosphoglycerate to 1,3-Bisphosphoglycerate:
- Enzyme: Phosphoglycerate Kinase
- Reaction: 3-Phosphoglycerate + ATP → 1,3-Bisphosphoglycerate + ADP
- Conversion of 1,3-Bisphosphoglycerate to Glyceraldehyde 3-Phosphate (G3P):
- Enzyme: Glyceraldehyde 3-Phosphate Dehydrogenase
- Reaction: 1,3-Bisphosphoglycerate + NADH → G3P + NAD⁺
- Formation of Fructose 1,6-Bisphosphate:
- Enzyme: Aldolase
- Reaction: G3P + Dihydroxyacetone Phosphate (DHAP) → Fructose 1,6-Bisphosphate
- Conversion of Fructose 1,6-Bisphosphate to Fructose 6-Phosphate:
- Enzyme: Fructose 1,6-Bisphosphatase
- Reaction: Fructose 1,6-Bisphosphate + H₂O → Fructose 6-Phosphate + Pi
- Notes: This reaction represents the rate-limiting step of gluconeogenesis and is tightly regulated to control the overall rate of glucose production.
- Conversion of Fructose 6-Phosphate to Glucose 6-Phosphate:
- Enzyme: Phosphohexose Isomerase
- Reaction: Fructose 6-Phosphate ↔ Glucose 6-Phosphate
- Final Conversion to Glucose:
- Enzyme: Glucose-6-Phosphatase
- Reaction: Glucose 6-Phosphate + H₂O → Glucose + Pi
- Location: Endoplasmic reticulum
- Notes: This step is critical for releasing free glucose into the bloodstream, allowing it to be transported to tissues for energy or storage.
Precursors of Gluconeogenesis
Gluconeogenesis is the process through which glucose is synthesized from non-carbohydrate sources, primarily to maintain blood glucose levels during periods of fasting or intense physical activity. Several precursors contribute to this process, primarily lactate, glycerol, glucogenic amino acids, and some citric acid cycle intermediates. The role of these substrates is crucial for ensuring an adequate supply of glucose in the body.
- Lactate:
- Lactate is produced by anaerobic metabolism, primarily in skeletal muscles and erythrocytes.
- It is transported to the liver, where it is converted to pyruvate via the Cori cycle using the enzyme lactate dehydrogenase.
- Once converted to pyruvate, it enters the gluconeogenic pathway, ultimately contributing to glucose production.
- The contribution of lactate from the Cori cycle to overall glucose production increases with fasting duration. For instance, after 12, 20, and 40 hours of fasting, the contribution was 41%, 71%, and 92%, respectively.
- Glycerol:
- Glycerol is a byproduct of triglyceride hydrolysis in adipose tissue. It is transported to the liver, where it serves as a substrate for gluconeogenesis.
- In the liver, glycerol is phosphorylated by glycerol kinase to form glycerol-3-phosphate.
- The glycerol-3-phosphate is then oxidized to dihydroxyacetone phosphate, an intermediate in glycolysis, which feeds into the gluconeogenesis pathway.
- Glucogenic Amino Acids:
- These amino acids are derived from the hydrolysis of tissue proteins and include compounds like glutamine and alanine.
- They contribute by entering gluconeogenesis through two primary pathways: pyruvate and oxaloacetate.
- The carbon skeletons of these amino acids can directly enter the gluconeogenic cycle or enter indirectly via intermediates in the citric acid cycle, such as α-ketoglutarate and succinyl-CoA.
- Citric Acid Cycle Intermediates:
- Intermediates from the citric acid cycle can also act as precursors. For example, oxaloacetate plays a key role in feeding into gluconeogenesis.
- Some intermediates, such as succinyl-CoA and fumarate, can be converted to oxaloacetate, which in turn can be utilized in gluconeogenesis.
- Odd-Chain Fatty Acids:
- Odd-chain fatty acids are oxidized to produce propionate, which is a precursor for gluconeogenesis. Propionate is converted to succinyl-CoA and enters the citric acid cycle, which eventually leads to the production of glucose.
- This process is more prominent in ruminants, but has some relevance in nonruminants like humans, though it contributes to a lesser extent.
- Even-Chain Fatty Acids:
- The oxidation of even-chain fatty acids yields acetyl-CoA, but this cannot directly contribute to gluconeogenesis in mammals because it cannot form glucose without the glyoxylate cycle.
- The glyoxylate cycle, which is found in some organisms such as fungi, plants, and bacteria, allows the conversion of acetyl-CoA into glucose. However, this cycle is absent in humans, meaning even-chain fatty acids cannot be converted to glucose in humans.
Enzymes of Gluconeogenesis
Gluconeogenesis is the metabolic process that generates glucose from non-carbohydrate precursors. Several enzymes are involved in this process, each playing a crucial role, especially at the irreversible steps, distinguishing it from glycolysis. Here’s a breakdown of the key enzymes involved.
- Pyruvate Carboxylase:
- Location: Mitochondria.
- Function: Converts pyruvate into oxaloacetate using ATP and bicarbonate as substrates. This reaction is vital as it marks the initiation of gluconeogenesis and requires biotin as a cofactor.
- Regulation: Activated by acetyl-CoA and inhibited by ADP and glucose.
- Phosphoenolpyruvate Carboxykinase (PEPCK):
- Location: Both mitochondria and cytosol (exists in isozymes).
- Function: Catalyzes the conversion of oxaloacetate into phosphoenolpyruvate (PEP) using GTP as a phosphate donor. This step is essential for the continuation of gluconeogenesis.
- Regulation: Induced by glucagon and glucocorticoids, which enhance its gene expression.
- Fructose-1,6-Bisphosphatase:
- Location: Cytosol.
- Function: Converts fructose-1,6-bisphosphate into fructose-6-phosphate, marking the rate-limiting step of gluconeogenesis.
- Regulation: Activated by ATP and citrate, while inhibited by AMP and fructose-2,6-bisphosphate.
- Glucose-6-Phosphatase:
- Location: Endoplasmic reticulum (ER) of liver and kidney cells.
- Function: Converts glucose-6-phosphate into free glucose, which is released into the bloodstream.
- Significance: Muscle cells lack this enzyme, so they cannot release glucose but use it for their own energy needs.
- Other Enzymes Involved: While the enzymes above are crucial for the irreversible steps, several enzymes contribute to the reversible steps shared with glycolysis:
- Enolase: Converts PEP to 2-phosphoglycerate.
- Phosphoglycerate Mutase: Converts 2-phosphoglycerate to 3-phosphoglycerate.
- Phosphoglycerate Kinase: Converts 3-phosphoglycerate to 1,3-bisphosphoglycerate.
- Glyceraldehyde 3-Phosphate Dehydrogenase: Reduces 1,3-bisphosphoglycerate to glyceraldehyde 3-phosphate.
- Triose Phosphate Isomerase: Interconverts dihydroxyacetone phosphate (DHAP) and glyceraldehyde 3-phosphate.
- Aldolase A: Combines two glyceraldehyde 3-phosphate molecules to form fructose 1,6-bisphosphate.
Substrates of Gluconeogenesis
Gluconeogenesis relies on various substrates to synthesize glucose from non-carbohydrate sources, ensuring that blood glucose levels remain stable during periods of fasting or increased energy demands. These substrates come from different metabolic pathways, including the breakdown of fats, proteins, and other intermediates.
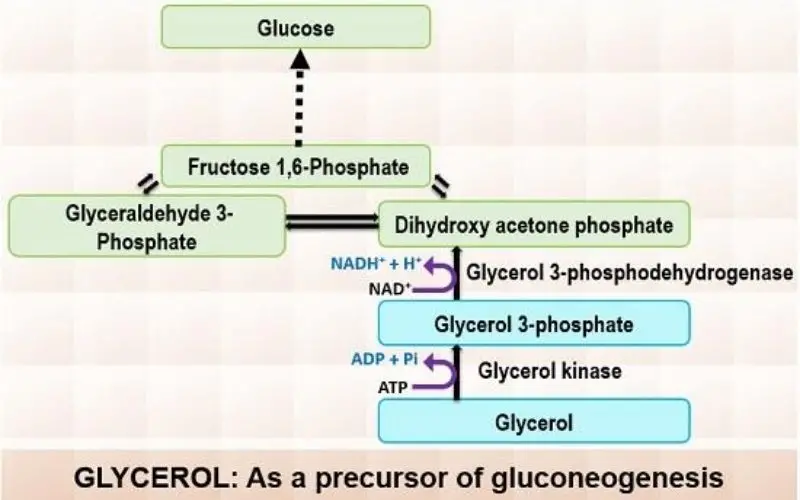
- Glycerol:
- Glycerol is derived from the hydrolysis of triglycerides in adipose tissue and is transported to the liver via the bloodstream.
- In the liver, glycerol kinase catalyzes the phosphorylation of glycerol to glycerol-3-phosphate using ATP.
- The glycerol-3-phosphate is then oxidized to dihydroxyacetone phosphate, an intermediate in glycolysis, with NAD+ being reduced to NADH in the process. This enables glycerol to feed into the gluconeogenic pathway and ultimately contribute to glucose formation.
- Lactate:
- Lactate is produced during anaerobic glycolysis in tissues like skeletal muscles and erythrocytes.
- It is transported to the liver, where it is converted back to pyruvate. This process is essential for lactate to contribute to gluconeogenesis.
- Once converted to pyruvate, lactate can enter the gluconeogenic pathway and ultimately be used to form glucose.
- Glucogenic Amino Acids:
- These amino acids are released during the hydrolysis of tissue proteins.
- Glucogenic amino acids, such as α-ketoglutarate, succinyl-CoA, fumarate, and oxaloacetate, are the primary contributors to gluconeogenesis.
- These amino acids can enter the gluconeogenesis pathway via two key entry points: pyruvate and oxaloacetate. From there, they are converted into glucose, playing a critical role in maintaining glucose levels during periods of low dietary intake or fasting.
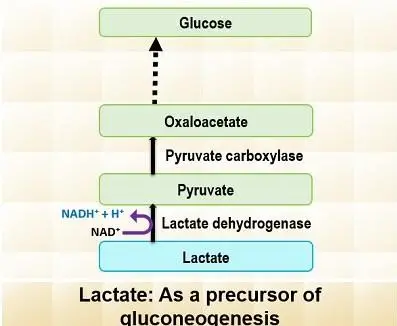
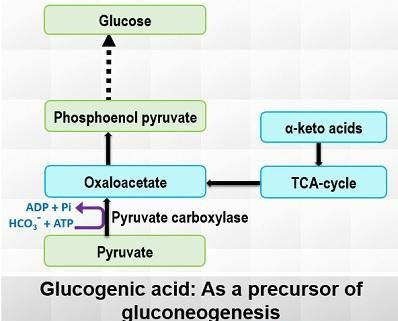
Gluconeogenesis Regulation
The regulation of gluconeogenesis ensures the synthesis of glucose when the body requires it most, particularly during fasting or low-energy conditions. This process is tightly controlled at various levels, involving key enzymes, hormonal signals, and the body’s energy state.
- Key Regulatory Enzymes
- Pyruvate Carboxykinase (PEPCK):
- Allosteric Regulation: PEPCK is activated by Acetyl-CoA, a signal indicating high energy. ADP serves as an inhibitor, signaling low energy and thus reducing the rate of gluconeogenesis.
- Transcriptional Regulation: The expression of PEPCK is upregulated by glucagon and glucocorticoids through the activation of the CREB transcription factor, enhancing its gene expression.
- Fructose 1,6-Bisphosphatase:
- Allosteric Inhibition: This enzyme is inhibited by AMP and fructose 2,6-bisphosphate, both of which signal low energy states. Their presence reduces the enzyme’s activity to align gluconeogenesis with cellular energy demands.
- Competitive Inhibition: The presence of fructose 2,6-bisphosphate competes with the enzyme’s substrate, reducing its effectiveness when substrate levels are low.
- Glucose-6-Phosphatase:
- This enzyme controls the final step of gluconeogenesis by converting glucose-6-phosphate to glucose. Its activity increases with higher glucose-6-phosphate concentrations, ensuring glucose release when needed.
- Pyruvate Carboxykinase (PEPCK):
- Hormonal Regulation
- Glucagon: This hormone plays a key role during fasting, increasing gluconeogenesis. It stimulates the expression of crucial enzymes like PEPCK and pyruvate carboxylase, enhancing transcription and promoting glucose synthesis.
- Insulin: In contrast, insulin inhibits gluconeogenesis in postprandial states (after eating). It downregulates gluconeogenic genes to prevent excessive glucose production, shifting metabolism toward glycolysis instead.
- Nutritional and Energy State Influence
- Energy Availability: When cellular ATP levels are high, gluconeogenesis is inhibited. Conversely, when ATP/ADP ratios are low, gluconeogenesis is promoted to generate glucose as an energy source.
- Substrate Availability: Gluconeogenesis is also driven by the availability of substrates like lactate and amino acids, especially during fasting, when these substrates are mobilized from other metabolic pathways to support glucose production.
Clinical Significance of Gluconeogenesis
Gluconeogenesis is essential not just for maintaining normal glucose levels but also for preventing metabolic disorders. The pathway’s dysfunction can lead to various clinical conditions that severely impact overall health. Specific diseases linked to gluconeogenesis emphasize its critical role in metabolic balance, glucose regulation, and energy supply.
- Glycogen Storage Disease Type 1 (Von Gierke Disease):
- This disease results from a deficiency of glucose-6-phosphatase, an enzyme crucial for the final step of gluconeogenesis, preventing glucose release from the liver.
- Liver and kidney enlargement occur due to the accumulation of glucose-6-phosphate, a precursor in both gluconeogenesis and glycogenolysis.
- Severe hypoglycemia arises during fasting, as glucose cannot be released from the liver into the bloodstream.
- Lactic acidosis occurs due to the blockage of gluconeogenesis, causing the accumulation of lactate in the bloodstream.
- Hypertriglyceridemia results from elevated glucose-6-phosphate levels that drive glycolysis and acetyl-CoA production, inhibiting fatty acid beta-oxidation by disrupting carnitine acyltransferase 1 activity.
- Hyperuricemia occurs as lactate competes with uric acid for excretion, leading to increased uric acid in the bloodstream.
- Other symptoms include protruding abdomen, truncal obesity, short stature, muscle wasting, and a rounded doll’s face.
- Pyruvate Carboxylase Deficiency:
- Caused by a lack of pyruvate carboxylase, an enzyme that converts pyruvate into oxaloacetate, this deficiency disrupts gluconeogenesis.
- Results in lactic acidosis, hyperammonemia, and hypoglycemia due to a failure to synthesize glucose from non-carbohydrate sources.
- Hyperammonemia occurs because pyruvate cannot be converted to oxaloacetate, which is needed for the urea cycle. This results in reduced aspartate production, impacting ammonia processing.
Importance of Gluconeogenesis
Gluconeogenesis is a crucial metabolic pathway that ensures a steady supply of glucose when dietary intake is low or absent. This process plays a significant role in blood glucose regulation, energy supply for vital tissues, and metabolic balance, especially during periods of fasting, starvation, or intense exercise.
- Blood Glucose Regulation:
- Gluconeogenesis is essential for maintaining blood glucose levels when dietary carbohydrates are lacking, particularly during fasting or periods of low carbohydrate intake.
- When glycogen stores are depleted, gluconeogenesis becomes the primary mechanism for glucose production.
- The liver is the main site of gluconeogenesis, with the kidneys also contributing, especially during prolonged fasting.
- Energy Supply for Essential Tissues:
- Red blood cells (RBCs), neurons, and skeletal muscles are heavily dependent on glucose for energy.
- Gluconeogenesis ensures these tissues receive the glucose needed to maintain cellular functions and energy metabolism, particularly when other energy sources are unavailable.
- Metabolite Clearance:
- The gluconeogenesis pathway helps clear certain metabolites from the bloodstream, such as lactate and glycerol.
- Lactate, produced during anaerobic respiration in muscles and RBCs, is converted back into glucose in the liver via gluconeogenesis.
- This process is a critical part of the Cori cycle, which helps maintain metabolic balance by recycling metabolites.
- Adaptation to Stress and Starvation:
- During periods of stress or prolonged fasting, gluconeogenesis becomes increasingly important.
- The pathway enables the body to produce glucose from amino acids like alanine, and non-carbohydrate precursors such as lactate and glycerol.
- This ensures the continuous supply of glucose to vital organs, even when external sources of carbohydrates are not available.
- Role in Acid-Base Balance:
- Gluconeogenesis helps maintain acid-base homeostasis by utilizing metabolites that could otherwise lead to acidosis.
- By converting lactate and other acidic metabolites into glucose, gluconeogenesis plays a role in stabilizing the body’s pH level, preventing excess acid accumulation.
Difference between Gluconeogenesis and Glycogenolysis – Glycogenolysis vs Gluconeogenesis
Gluconeogenesis and glycogenolysis are two vital metabolic pathways involved in maintaining glucose levels in the body. While they both serve the purpose of providing glucose, they operate through different processes and mechanisms. Here’s a breakdown of how they differ.
- Process Type:
- Gluconeogenesis is an anabolic process, meaning it builds larger molecules (glucose) from smaller precursors like lactate, pyruvate, glycerol, and amino acids.
- Glycogenolysis is a catabolic process, which breaks down larger molecules like glycogen into smaller units (glucose or glucose-6-phosphate).
- Substrates Used:
- Gluconeogenesis relies on non-carbohydrate substrates, such as lactate, pyruvate, glycerol, and certain glucogenic amino acids, to generate glucose.
- Glycogenolysis, on the other hand, uses glycogen, a stored form of glucose, to produce glucose or glucose-6-phosphate.
- Location:
- Gluconeogenesis primarily takes place in the liver, and to a lesser extent in the kidneys.
- Glycogenolysis occurs in both the liver and muscle cells, providing glucose to different tissues based on immediate energy needs.
- Hormonal Regulation:
- Gluconeogenesis is stimulated by glucagon and cortisol, hormones that promote glucose production during states of fasting or low blood sugar.
- Glycogenolysis is triggered by glucagon as well, but epinephrine (adrenaline) also plays a crucial role in stimulating glycogen breakdown, particularly during exercise or stress when quick energy is required.
- Final Products:
- Gluconeogenesis ultimately produces glucose, which is released into the bloodstream to maintain blood glucose levels during periods of fasting or after prolonged exercise.
- Glycogenolysis also produces glucose or glucose-6-phosphate, which can either be used immediately for energy within the cell or converted to free glucose for release into the bloodstream.
Feature | Gluconeogenesis | Glycogenolysis |
---|---|---|
Type | Anabolic | Catabolic |
Substrates | Lactate, pyruvate, glycerol, amino acids | Glycogen |
Location | Liver (and kidneys) | Liver and muscle |
Hormonal Regulation | Stimulated by glucagon and cortisol | Stimulated by glucagon and epinephrine |
Final Product | Glucose | Glucose (or glucose-6-phosphate) |
- Melkonian EA, Asuka E, Schury MP. Physiology, Gluconeogenesis. [Updated 2023 May 1]. In: StatPearls [Internet]. Treasure Island (FL): StatPearls Publishing; 2023 Jan-. Available from:https://www.ncbi.nlm.nih.gov/books/NBK541119/
- Chourpiliadis C, Mohiuddin SS. Biochemistry, Gluconeogenesis. [Updated 2023 Jun 5]. In: StatPearls [Internet]. Treasure Island (FL): StatPearls Publishing; 2024 Jan-. Available from: https://www.ncbi.nlm.nih.gov/books/NBK544346/
- https://www.google.com/search?q=Gluconeogenesis&sourceid=chrome&ie=UTF-8#:~:text=GluconeogenesisPathwaySignificance,orggluconeogenesis
- https://biologyreader.com/gluconeogenesis.html
- https://en.wikipedia.org/wiki/Gluconeogenesis
Cite this post:
Sourav Pan. (2024, December 17). Gluconeogenesis – Definition, Steps, Reactions, Substrates, Importance. Biology Notes Online. Retrieved from https://biologynotesonline.com/gluconeogenesis/
Sourav Pan. "Gluconeogenesis – Definition, Steps, Reactions, Substrates, Importance." Biology Notes Online, 17 December 2024, biologynotesonline.com/gluconeogenesis/.
Sourav Pan. "Gluconeogenesis – Definition, Steps, Reactions, Substrates, Importance." Biology Notes Online (blog). December 17, 2024. https://biologynotesonline.com/gluconeogenesis/.