Gause’s Principle, also known as the competitive exclusion principle or Gause’s law, is a fundamental concept in ecology that describes the competition between species for limited resources. It states that two species that compete for the same resources cannot coexist in the long term at constant population values. When one species has even a slight advantage over the other, it will eventually dominate and outcompete the weaker competitor.
The principle suggests that in a stable environment with limited resources, such as food, water, or territory, species with similar ecological niches cannot coexist indefinitely. The reason behind this is that when two species occupy the same niche and have identical resource requirements, they will inevitably compete for the same resources. As competition intensifies, the species with a competitive advantage, whether it’s due to superior adaptations, more efficient resource utilization, or higher reproductive rates, will eventually outcompete and displace the other species.
According to Gause’s Principle, this competitive exclusion leads to one of two outcomes. First, the weaker competitor may face extinction if it fails to find alternative resources or adapt to a different ecological niche. The stronger species will utilize the resources more effectively, leaving less for the weaker species, eventually leading to its decline and disappearance. Second, in some cases, the weaker competitor may undergo an evolutionary or behavioral shift to occupy a different ecological niche, thereby reducing direct competition with the dominant species. This shift allows both species to coexist by utilizing different resources or occupying different habitats.
Although Gause is often associated with the competitive exclusion principle, it is important to note that he did not formulate it himself. The principle is actually rooted in Charles Darwin’s theory of natural selection, which emphasizes the struggle for existence and the differential survival and reproduction of individuals with advantageous traits. Gause’s work, however, contributed to a better understanding of the principle through experiments and observations.
Over time, the interpretation and acceptance of Gause’s Principle have evolved. Initially, it was considered more of an a priori assumption, stating that two coexisting species must have different ecological niches. As research progressed and experimental evidence accumulated, it became evident that coexisting species indeed tend to occupy different niches, supporting the competitive exclusion principle as an experimental truth.
Gause’s Principle has profound implications for understanding species interactions, community dynamics, and the organization of ecosystems. It highlights the importance of resource partitioning and niche differentiation in promoting species coexistence. By occupying different ecological niches, species can reduce direct competition and coexist within the same ecosystem, contributing to the biodiversity and stability of natural communities.
In summary, Gause’s Principle, or the competitive exclusion principle, states that two species competing for the same limited resources cannot coexist indefinitely. The principle reflects the outcomes of intense competition, where the species with a competitive advantage will dominate, leading to the decline or displacement of the weaker competitor. Understanding this principle enhances our understanding of species interactions and the dynamics of ecological communities.
What is Gause’s Principle?
- Gause’s Principle, also known as the competitive exclusion principle (CEP), is a concept in ecology that states two species with identical niches and competing for a single resource cannot coexist together indefinitely. The principle, often attributed to Gause and first introduced in 1934, has become one of the most influential and controversial ideas in the field of ecology.
- The competitive exclusion principle is a simple yet powerful statement that has profound implications for our understanding of species coexistence and diversity. It serves as a centralizing paradox in ecology because if similar competing species cannot coexist, then how do we explain the tremendous patterns of diversity observed in nature? How do competitors manage to coexist despite their overlapping resource requirements?
- For over a century, ecologists have focused their research on resource competition as the primary interaction that regulates and structures populations and communities. Early ecologists, including Darwin, recognized that species occupy specific roles in their environment and that ecologically similar species, particularly closely related ones, engage in strong competition that can lead to the extinction of less competitive species. They also acknowledged that species could diverge in their resource use, resulting in reduced niche overlap. These interactions were believed to be the driving mechanisms behind speciation and the vast diversity of life on Earth.
- In the early 20th century, Grinnell coined the term “niche” to describe the requirements and habitat use of species. He expressed the notion that no two species can occupy the same niche. Building upon this concept, Elton later expanded the definition of niche to include a species’ role and effects in its habitat.
- The competitive exclusion principle challenges our understanding of how species coexist despite their similar resource requirements. If two species have identical niches and directly compete for the same resource, one would expect that the superior competitor would eventually outcompete and exclude the other species, leading to its extinction. However, we observe a remarkable diversity of species coexisting in nature, which suggests that there must be mechanisms that allow for coexistence.
- To reconcile this paradox, ecologists have proposed that niche differences among species play a vital role in the maintenance of species coexistence. When species evolve to utilize different resources or occupy slightly different ecological niches, niche overlap decreases, reducing direct competition. This niche differentiation allows species to partition resources and coexist in the same habitat.
- The mechanisms underlying species coexistence and diversity are complex and multifaceted. In addition to niche differentiation, other factors such as predation, mutualism, disturbance, and environmental variability also contribute to the maintenance of species diversity. Ecologists continue to investigate these mechanisms to gain a deeper understanding of how species coexist and interact within ecological communities.
- Gause’s Principle, or the competitive exclusion principle, serves as a foundational concept in ecology, driving research and theoretical approaches to species coexistence and diversity. It highlights the importance of niche differentiation and resource partitioning in enabling the persistence of multiple species in ecological communities, despite their potential for competition. By studying the mechanisms that allow species to coexist, ecologists contribute to our broader understanding of the intricate web of life on our planet.
The Struggle of Existence
- The concept of “the struggle for existence” has a long history, but it was Charles Darwin who popularized the term in his seminal work published in 1859. Darwin used this concept to describe the intense competition among individuals of the same species or different species for limited resources. However, despite this struggle for survival, we observe the persistence of species within ecological communities, raising intriguing questions about the patterns we observe at the community level.
- Understanding the nature of species dominance, persistence, and extinction, as well as the structure and assembly of communities, patterns of biodiversity, and the mechanisms underlying these patterns, has been a central focus of ecological research. In the early 20th century, two scientists, Lotka and Volterra, independently developed mathematical equations to describe the population dynamics of competing species. These equations aimed to determine the conditions under which species would either go extinct or stably coexist in a population.
- For instance, when two species compete for a single resource, only one species can persist in the long term. However, if the intensity of competition within each species (intraspecific competition) is greater than the competition between the two species (interspecific competition), similar species may coexist stably. These mathematical models provided insights into the dynamics of species interactions and the conditions necessary for coexistence.
- In 1934, Gause conducted pioneering experiments to test the predictions of Lotka-Volterra equations. He focused on studying competition between unicellular organisms, such as protozoa and yeast, in laboratory settings. Gause’s experiments aimed to quantify species interactions and determine the conditions required for coexistence. One notable experiment involved two Paramecium species that exhibited logistic growth when grown alone but resulted in the extinction of one species when grown together. This pattern of competitive exclusion, where one species outcompetes and eliminates the other, had been previously observed by naturalists and theoreticians. Gause’s contribution was to unite the concepts of niche differentiation and competitive interactions, both conceptually and empirically.
- Gause’s book, “The Struggle for Existence,” presented the first experimental tests of the Lotka-Volterra equations and shed light on the mechanisms underlying species coexistence and competition. His work demonstrated the significance of competition in shaping ecological communities and provided empirical evidence for the theoretical models proposed by Lotka and Volterra.
- Today, ecologists continue to investigate the complexities of species interactions, the factors influencing species coexistence, and the patterns of biodiversity in communities. They explore not only the role of competition but also other ecological processes such as predation, mutualism, and environmental factors in shaping the structure and dynamics of communities. By integrating empirical research, mathematical modeling, and theoretical frameworks, ecologists strive to unravel the intricacies of the struggle for existence and gain a deeper understanding of the remarkable diversity of life on Earth.
Contributions of Gause’s
- Gause’s contributions to the field of ecology, particularly in the areas of competition, the niche, and species coexistence, have had a lasting impact on the discipline. His pioneering work, which involved experimentally testing mathematical models, set a standard for ecologists and provided valuable insights into ecological interactions.
- Gause’s experimental results have become widely recognized and frequently cited examples in textbooks, particularly in the context of competition and predator-prey interactions. His approach of combining empirical research with mathematical modeling has become a cornerstone of ecological studies, allowing researchers to better understand and predict ecological dynamics.
- Following the publication of Gause’s book, various terms were used to refer to the concept now known as the “competitive exclusion principle.” It was not until 1960 that the term “competitive exclusion principle” was formally coined by Hardin. Hardin’s article not only summarized the historical development of the principle but also provided a critical evaluation of its applicability and the challenges associated with testing it.
- One of the main critiques raised by Hardin and others is that the competitive exclusion principle is difficult to falsify. When extinction occurs between competing species, it supports the principle. However, when species coexist, the conclusion is often that they must have some ecological differences or occupy different niches. This raises the question of how different two species must be in order to coexist. The issue of determining the extent of niche differentiation required for coexistence has been a subject of ongoing debate and exploration in ecology.
- Despite the challenges and critiques, competition experiments continue to play a prominent role in ecological research. Over the years, the approaches and experimental designs have become more quantitative and rigorous, allowing for a deeper understanding of the mechanisms underlying species interactions and coexistence. Researchers have developed sophisticated mathematical models, conducted controlled experiments, and analyzed large datasets to elucidate the dynamics of competition and its implications for community structure and biodiversity.
- Gause’s contributions have paved the way for advancements in understanding the complexities of species interactions and the maintenance of biodiversity. His work continues to inspire ecologists to explore new avenues of research, develop innovative experimental approaches, and refine our understanding of the ecological processes that shape the natural world.
Species Coexistence
- Species coexistence, the phenomenon in which multiple species with similar ecological requirements inhabit the same habitat, has been a central focus in ecology. Understanding the factors that enable species to coexist has been a long-standing goal for ecologists. The concept of competitive exclusion, which suggests that species with identical niches cannot coexist indefinitely, has played a significant role in shaping our understanding of species interactions.
- To explain species coexistence, researchers have explored various mechanisms and outcomes of competitive interactions. Trait differences among species, as implied by the competitive exclusion principle, have been identified as a key factor in facilitating coexistence. Intraspecific interactions, interactions within the same species, are often stronger than interspecific interactions, interactions between different species. This difference in interaction strength allows species to partition resources and reduce direct competition, promoting coexistence.
- Mathematical models, such as the Lotka-Volterra equations, have been employed to study the conditions under which coexistence is possible. Theoretical studies have indicated that the number of resources or limiting factors should exceed the number of species for coexistence to occur. Other factors, including nonlinear functional response relationships, varying densities, and temporal variation in resources, have also been identified as conditions that facilitate coexistence.
- The nature of competitive interactions can vary depending on environmental conditions. Different habitats or varying spatial and temporal scales can lead to variations in the strength of competition, influencing coexistence patterns. Habitat heterogeneity, both spatially and temporally, plays a crucial role in facilitating coexistence by providing niche differentiation opportunities for species.
- Character displacement, a process driven by resource competition, can contribute to species coexistence. When competitors evolve to reduce niche overlap and diverge in their resource use, they can coexist. This process is often associated with morphological differences that reflect differential resource utilization. Evidence supporting character displacement includes nonrandom size differences, genetic basis for trait differences, evolutionary shifts, morphological differences in resource use, and established competitive interactions.
- The concept of the niche, pioneered by G.E. Hutchinson, has played a significant role in understanding species coexistence. Hutchinson emphasized that species must differ sufficiently in body size and resource use to coexist. He introduced the Hutchinsonian ratio, suggesting that a minimum difference in body size of 130% is necessary for coexistence. While the niche concept faced criticism based on statistical and inferential grounds, it has experienced a resurgence in recent years. The niche has been enhanced through the incorporation of species traits, and niche tradeoffs have been proposed as a mechanism for coexistence.
- The metacommunity concept has emerged as a framework to understand community dynamics at different spatial scales. Local and regional processes interact to shape patterns of abundance and diversity. The neutral theory of species diversity provides an alternative perspective, assuming ecological and demographic similarities among species, and emphasizing stochastic processes in driving diversity patterns.
- Niche tradeoffs, which involve negative functional relationships between species traits, have gained attention as a mechanism for species coexistence. These tradeoffs can occur along multiple niche axes in spatial and temporal dimensions, potentially contributing to higher levels of diversity. Spatial dynamics and colonization abilities have also been proposed as factors promoting coexistence in spatially structured environments.
- The relationship between evolutionary history and ecological traits in species coexistence is an ongoing area of research. The importance of competitive exclusion in structuring communities has been evaluated through empirical studies, yielding diverse results. Other factors, such as stochastic processes, dispersal limitation, apparent competition, and abiotic conditions, have also been recognized as influential in determining coexistence patterns.
- While the understanding of species coexistence continues to evolve, the competitive exclusion principle and its modern extensions remain influential in ecological research. Advances in technology, empirical studies, and theoretical developments will further contribute to our knowledge of species interactions and coexistence in the future.
The Competitive Exclusion Principle, or Gause’s law Explanation
The Competitive Exclusion Principle, also known as Gause’s law, posits that when two species compete for the same limited resources in an ecological niche, one species will eventually outcompete and drive the other species to local extinction. This principle applies to both intraspecific competition, which occurs within the same species, and interspecific competition, which involves competition between different species.
In the case of intraspecific competition, individuals of the same species compete for resources such as food, territory, or mates. This competition limits the population size, as the available resources can only support a certain number of individuals. This maximum population size is referred to as the carrying capacity, which represents the threshold beyond which the environment cannot sustain the species.
Interspecific competition, on the other hand, occurs when different species vie for the same resources. When two species occupy the same ecological niche and have similar resource requirements, the Competitive Exclusion Principle suggests that the species that is better adapted to the niche will have a competitive advantage. Even a slight advantage can lead to the eventual elimination of the less adapted species, driving it to local extinction.
Georgy Gause, a biologist, conducted influential research on interspecific competition using Paramecium, a type of single-celled organism. In his experiments, Gause observed that when two Paramecium species were grown together, one species consistently outcompeted the other, resulting in the extinction of the weaker species. These findings, along with similar research, led Gause to formulate the Competitive Exclusion Principle.
The principle highlights the significance of competition in shaping species interactions and community dynamics. It emphasizes the role of adaptation and resource utilization in determining the outcome of competition. According to Gause’s law, species that are better adapted to a specific niche or have superior resource utilization abilities will have a competitive edge, ultimately leading to the exclusion of less adapted species.
The Competitive Exclusion Principle has been supported by numerous experimental studies and observations in various ecological systems. However, it is important to note that the principle is a generalization and does not account for all cases of species coexistence. Other factors, such as habitat heterogeneity, spatial dynamics, temporal variation, and niche differentiation, can contribute to species coexistence by reducing direct competition or promoting resource partitioning.
In summary, the Competitive Exclusion Principle, or Gause’s law, asserts that when two species compete for the same limited resources in an ecological niche, the better adapted species will ultimately drive the other species to local extinction. This principle provides valuable insights into the dynamics of species interactions and the structuring of ecological communities.
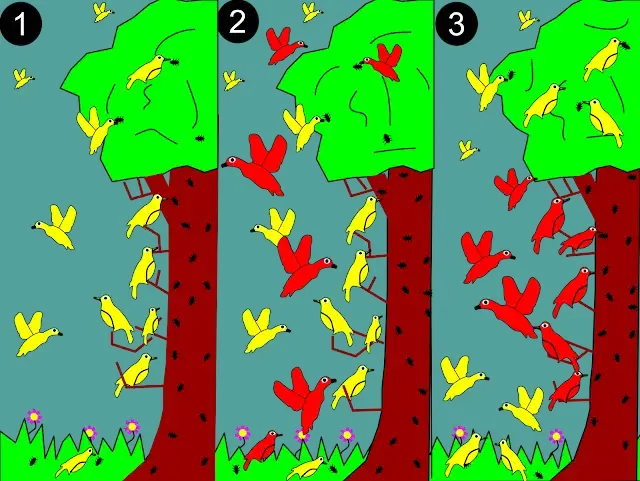
Direct or interference Competetion
- Direct or interference competition refers to a type of competition where individuals or species directly interact and interfere with one another’s access to resources, leading to a negative impact on their fitness and population dynamics. This form of competition can occur between individuals of the same species (intraspecific competition) or different species (interspecific competition).
- One classic example of direct competition is the study conducted by Robert T. Paine in the rocky intertidal zone of Scotland, known as Connel’s field experiment. The experiment focused on two species of barnacles, Balanus balanoides and Chthamalus stellatus, which compete for space on the rocky substrate. Balanus, the larger barnacle species, dominates the lower intertidal zone, while Chthamalus occupies the upper zone.
- The larvae of both species have the ability to settle and attach themselves over a wider vertical range than the zone occupied by adults. However, the presence of Balanus prevents the establishment and survival of Chthamalus in the lower zone. This is because Chthamalus is more tolerant of physical desiccation caused by exposure to air during low tides, while Balanus is less tolerant. As a result, Balanus outcompetes and removes Chthamalus from the lower intertidal zone.
- Connell’s experiment highlighted the importance of biotic factors, such as competition with other species, in determining the distribution and abundance of intertidal organisms. The lower limit of distribution for these barnacle species is primarily determined by the competitive interactions between them. In contrast, the upper limit of their distribution is often constrained by physical factors, such as the dry conditions experienced during low tides.
- This example illustrates how direct competition can shape the structure and composition of ecological communities. When species compete directly for limited resources, such as space, food, or other essential factors, the better-adapted or more competitive species often gains a competitive advantage and excludes or suppresses the other species.
- Direct competition can have significant impacts on population dynamics, species interactions, and community structure. It can lead to the exclusion or displacement of weaker competitors, alter species distributions, and influence the evolution of traits related to resource acquisition and utilization.
- Understanding the dynamics of direct competition is crucial for comprehending the processes that shape biodiversity patterns and the coexistence or exclusion of species in various ecosystems. By investigating the mechanisms and outcomes of direct competition, ecologists gain insights into the intricate relationships and interactions among organisms in their natural environments.
Indirect or exploitation competition
- Indirect or exploitation competition refers to a form of competition in which individuals or species compete for shared resources indirectly, without direct physical interaction or interference. Instead, competition occurs through the exploitation of limited resources, such as food, habitat, or other essential factors.
- One way to study and understand indirect competition is through experiments that test the Competitive Exclusion Principle. An example of such an experiment is conducted by students, following a design similar to the original Paramecium experiment by Gause. In this investigation, students focus on two species of Paramecium: Paramecium caudatum and Paramecium aurelia.
- Paramecium caudatum and Paramecium aurelia are suitable model organisms for testing the Competitive Exclusion Principle due to their similarity and their direct competition for food resources. However, they differ in size, with Paramecium caudatum being approximately four times larger than Paramecium aurelia.
- In the experiment, students set up three separate culture samples: one with only Paramecium caudatum, one with only Paramecium aurelia, and one with both species together. It is crucial to maintain the same environmental conditions for all three cultures, as the Competitive Exclusion Principle applies only when ecological factors remain constant.
- Over a period of three weeks, students observe and track the population growth of the two Paramecium species in each culture. By comparing the growth patterns of the species when grown separately versus when grown together, students can assess the impact of competition for resources on population growth.
- If the Competitive Exclusion Principle holds true, the species that is better adapted or more efficient at exploiting the available resources should outcompete and suppress the other species. As a result, the population of the inferior competitor would decline or become extinct in the presence of the dominant competitor.
- Through this experiment, students can directly observe the effects of indirect competition on population dynamics and test the predictions of the Competitive Exclusion Principle. The results of the experiment can provide insights into the role of resource availability and competition in shaping population growth and species interactions.
- Studying indirect competition is crucial for understanding the dynamics of ecological communities and the factors that influence species coexistence or exclusion. It helps elucidate the mechanisms by which organisms interact and compete for shared resources, shedding light on the patterns and processes that govern biodiversity and community structure in various ecosystems.
Experimental basis of Competitive Exclusion Principle
The Experimental basis of the Competitive Exclusion Principle stems from the pioneering work of scientists such as Joseph Grinnell and Georgy Gause, who conducted field observations and laboratory experiments to test the dynamics of species competition.
Joseph Grinnell, in 1904, observed the competitive interactions between species in the field and formulated the principle of competitive exclusion. He noted that when two species with similar food habits occupy the same region, they are unlikely to maintain a long-term balance in numbers. Eventually, one species would outcompete and displace the other, resulting in the dominance of a single species. This observation highlighted the role of competition in shaping species distributions and community structure.
Georgy Gause further expanded on the concept of competitive exclusion through his laboratory experiments. Using two species of Paramecium, P. aurelia and P. caudatum, Gause conducted competition experiments under controlled conditions. He ensured a constant supply of food and daily addition of fresh water. Initially, P. caudatum dominated the environment. However, as the experiment progressed, P. aurelia recovered and eventually drove P. caudatum to extinction through exploitative resource competition. Gause’s experiments demonstrated the outcome predicted by the Competitive Exclusion Principle.
However, Gause also observed that by altering the environmental parameters, such as the availability of food and water, he could prevent the extinction of P. caudatum. This observation emphasized that the Competitive Exclusion Principle holds true only when ecological factors remain constant. Changes in environmental conditions can create opportunities for coexistence or alter the outcome of competition.
In addition to Paramecium, Gause also investigated competition between two species of yeast, Saccharomyces cerevisiae and Schizosaccharomyces kefir. He discovered that Saccharomyces cerevisiae consistently outcompeted Schizosaccharomyces kefir by producing a higher concentration of ethyl alcohol. This experiment further supported the Competitive Exclusion Principle by demonstrating how one species can gain a competitive advantage over another through specific traits or resource utilization.
The experimental studies conducted by Grinnell and Gause provided empirical evidence for the Competitive Exclusion Principle. These experiments demonstrated the dynamics of competition, the displacement of one species by another, and the influence of ecological factors on the outcome of competition. The principles derived from these experiments have since become foundational concepts in ecology and continue to shape our understanding of species interactions and community dynamics.
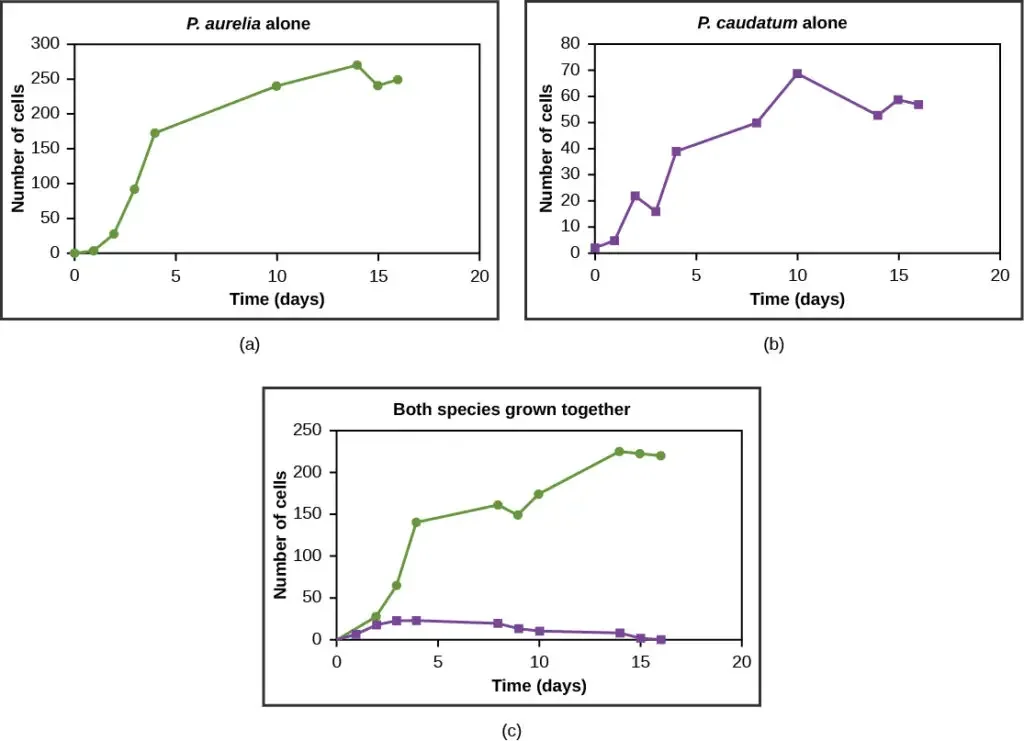
Prediction
The prediction of Gause’s law, also known as the Competitive Exclusion Principle, is often supported by mathematical and theoretical models of competition, such as the Lotka-Volterra models. These models suggest that when two species compete for the same limited resources, one species will eventually outcompete and drive the other to extinction.
However, in natural ecosystems, the observation of competitive exclusion is rare, and many biological communities seem to violate Gause’s law. One notable example is the “paradox of the plankton,” which challenges the principle of competitive exclusion. In the open sea, plankton species rely on limited resources such as solar energy and dissolved minerals in the water. According to the Competitive Exclusion Principle, only a few plankton species should be able to coexist under these resource constraints. However, in reality, numerous plankton species coexist within small regions of the open sea, defying the predicted outcomes of competitive exclusion.
While competitive exclusion is not commonly observed in natural ecosystems, there are some communities that appear to uphold the principle. MacArthur’s warblers and Darwin’s finches are often cited as examples. These species demonstrate ecological overlap and competition, but their coexistence is maintained under specific conditions. For instance, in the case of Darwin’s finches, competition may negatively impact the species only under extreme conditions, allowing for coexistence in other ecological contexts.
The discrepancy between the predictions of competitive exclusion and the observed patterns in natural communities suggests that there are factors beyond resource competition influencing species coexistence. These factors could include niche differentiation, spatial heterogeneity, temporal variation, predation, or other biotic and abiotic interactions. The complexities of real-world ecosystems challenge the simplistic predictions of competitive exclusion, highlighting the need to consider a broader range of ecological processes and mechanisms when studying species interactions and community dynamics.
Laboratory example of Gause’s Principle
Background
The competitive exclusion principle, also known as Gause’s law, states that two species competing for the same limited resources cannot coexist or maintain constant population values in a sustainable manner. Intraspecific competition refers to organisms of the same species contending for resources, resulting in the population reaching carrying capacity. Carrying capacity is the utmost population size a species can support within the constraints of its environment. Interspecific competition is the competition for resources between organisms of distinct species. Both their carrying capacity (intraspecific competition) and interspecific competition can limit species. When two species compete for the same ecological niche, the competitive exclusion principle predicts that the better-adapted species, even if it is only marginally more adapted, will drive the other species to local extinction. In a groundbreaking study of competition in Paramecium conducted in the 1930s, biologist Georgy Gause investigated the concept of interspecific competition. Paramecia are aquatic unicellular ciliates that consume bacteria, yeast, algae, and other tiny protozoa for sustenance. Gause formulated the competitive exclusion principle based on the findings of this experiment and other research.
Students examine how competition affects population growth and evaluate the competitive exclusion principle in this investigation. Students evaluate two Paramecium species, Paramecium Caudatum and Paramecium Aurelia, using an experiment design remarkably similar to Gause’s original Paramecium experiment. Due to their similarity and direct competition for sustenance, these two species are excellent model organisms for examining this principle. However, their sizes differ, with Paramecium Caudatum being approximately four times larger than Paramecium Aurelia. Students are required to cultivate the two species separately and in combination in a culture medium. Gause’s law only applies if ecological factors remain constant, so the three culture samples are maintained under identical environmental conditions. Students observe population development over the course of three weeks to determine how competition for resources affects population growth.
Preparation – By Lab Technician
Upon receiving the shipment of Paramecium culture jars, it is important for the lab technician to follow proper procedures to ensure the viability and health of the cultures. Here are the steps involved in preparing the cultures:
- Inspect the Culture: Open the shipping container and carefully remove the Paramecium culture jars. Check the jars for any signs of damage or leakage. It is crucial to handle the cultures with care to avoid contamination or disruption of the organisms.
- Loosen the Lids: Gently loosen the lids on the culture jars. This allows for proper airflow and prevents the buildup of excessive pressure within the containers.
- Aerate the Culture: Using a plastic pipette, aerate the culture by introducing fresh air into the culture water. Insert the pipette tip into the water and squeeze the bulb to expel air. Raise the pipette, allowing it to fill with air again, and repeat this process four more times. Each culture should have a separate pipette to prevent cross-contamination.
- Replace the Lid: After aerating the cultures, place the lids back on the containers. Make sure not to screw them on too tightly, as it is important to allow for some airflow while maintaining a closed environment.
- Maintain Ideal Conditions: Store the culture jars at room temperature, around 21°C (approximately 70°F). Keep them away from direct sunlight, as excessive light exposure can affect the growth and behavior of the Paramecium. Ensure that the cultures are kept in a stable environment to minimize fluctuations in temperature and other factors that could impact the organisms’ well-being.
- Timing Considerations: Plan to conduct the experiment with the Paramecium cultures within three days of their arrival. This timeframe helps to ensure that the organisms remain healthy and active. Keep in mind that some Paramecium may not survive during shipping or in the initial stages of culture setup, so it is important to have sufficient quantities of Paramecium available for each student or experimental setup.
By following these preparation steps, the lab technician can create a suitable and conducive environment for the Paramecium cultures, setting the stage for a successful experiment. Attention to detail and adherence to proper handling techniques are essential in maintaining the health and viability of the organisms throughout the study.
Method – Student Activity
The following is a step-by-step guide for students to conduct the experiment involving Paramecium cultures. This activity aims to observe and analyze the population dynamics of Paramecium aurelia and Paramecium caudatum under different conditions. Here’s how to proceed:
- State your Hypothesis: Begin by formulating a hypothesis that predicts the outcome of the experiment. For example, you may hypothesize that the presence of both Paramecium aurelia and Paramecium caudatum in the same culture dish (Petri dish B) will lead to a different population dynamic compared to dishes with only one species (dishes A and C).
- Prepare the Petri Dishes: Take three clean Petri dishes and label them as A, B, and C. Ensure that the dishes are free from any contamination.
- Add Paramecium Culture Medium: Pour 50 mL of Paramecium culture medium into each Petri dish. The culture medium provides the necessary nutrients and environment for the Paramecium to grow.
- Transfer Paramecium Aurelia to Dish A: Using a graduated cylinder, transfer 20 mL of Paramecium aurelia culture into Petri dish A. This dish will serve as the control group, containing only Paramecium aurelia.
- Transfer Paramecium Caudatum and Paramecium Aurelia to Dish B: Transfer 10 mL of Paramecium caudatum culture and 10 mL of Paramecium aurelia culture into Petri dish B. This dish will simulate the competition between the two species.
- Transfer Paramecium Caudatum to Dish C: Transfer 20 mL of Paramecium caudatum culture into Petri dish C. This dish will contain only Paramecium caudatum, allowing for observation of its growth in the absence of competition.
- Add Grains of Rice: Place six grains of rice into each Petri dish. The rice grains will provide a food source for the Paramecium.
- Cover the Petri Dishes: Cover each Petri dish containing the Paramecium cultures with cheesecloth. This will prevent contamination while allowing airflow.
- Store the Petri Dishes: Place the Petri dishes on a flat surface away from direct sunlight, ensuring a consistent temperature of 24°C. Avoid disturbing the dishes throughout the experiment to maintain a stable environment.
- Prepare Sedgewick Rafter Cell Samples: Using a fresh sterile pipette, take 1 mL of liquid from each sample (dishes A, B, and C) and place it in a Sedgewick Rafter cell. This will allow for microscopic observation and counting of the Paramecium populations.
- Count Paramecium Population: Using a compound microscope, count the relative number of Paramecium of each species in each Petri dish. Repeat this count every second day for a total of three weeks. Record your observations and note any changes in population sizes over time.
By following this method, students can conduct the experiment and gather data on the population dynamics of Paramecium aurelia and Paramecium caudatum under different conditions. This will help them analyze the impact of competition on the growth and survival of these organisms.
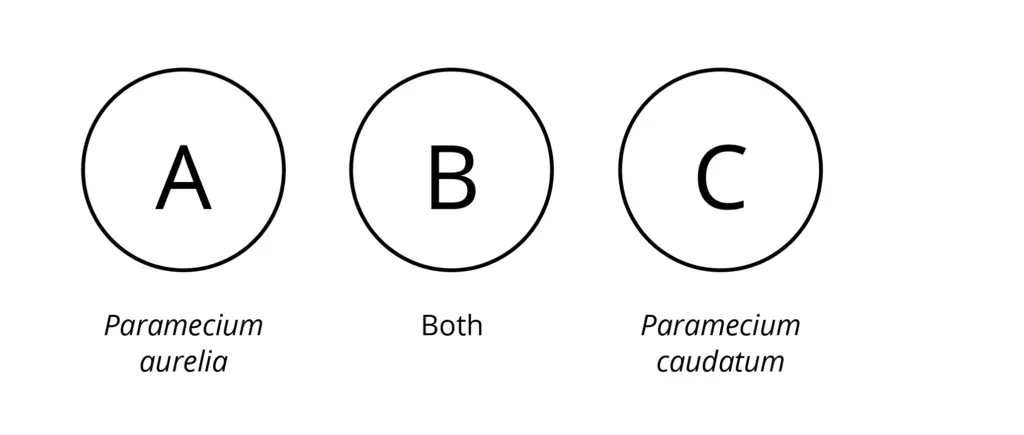
Observation And Results
After conducting the experiment and observing the population dynamics of Paramecium aurelia and Paramecium caudatum in the different Petri dishes, it’s time to analyze the results. Here is an example of expected results based on an initial population density of 10 Paramecium per 1 mL. Keep in mind that individual results may vary.
- Plot Individual Results: a. Petri Dish A (Paramecium aurelia only):
- Time (x-axis): Every 2 days
- Population size (y-axis): Paramecium per 1 mL
- Plot the growth of Paramecium aurelia over time in Petri dish A as a line graph. Observe whether the population increases, decreases, or remains stable.
b. Petri Dish B (Paramecium caudatum and Paramecium aurelia):
- Time (x-axis): Every 2 days
- Population size (y-axis): Paramecium per 1 mL
- Plot the growth of both Paramecium caudatum and Paramecium aurelia over time in Petri dish B on the same axes as separate lines. Observe any changes in population sizes and identify whether they increase, decrease, or remain stable.
c. Petri Dish C (Paramecium caudatum only):
- Time (x-axis): Every 2 days
- Population size (y-axis): Paramecium per 1 mL
- Plot the growth of Paramecium caudatum over time in Petri dish C as a line graph. Note any changes in population size and determine if it increases, decreases, or remains stable.
- Combine Class Results: Collaborate with the rest of the class to compile the results from each group. Collect the data for each Petri dish (A, B, and C) and calculate the average population sizes at different time points.
- Plot Class Results: a. Time (x-axis): Every 2 days b. Population size (y-axis): Paramecium per 1 mL c. Plot the average population sizes for each Petri dish (A, B, and C) on the same graph as separate lines. This will provide an overview of the class results and allow for comparisons between the different conditions.
- Describe the Population Dynamics: Analyze the observations made in populations A, B, and C based on the graphs and data collected. Describe the changes in population size over time and identify whether the populations increased, decreased, or remained stable.
For example:
- Population A (Paramecium aurelia only): The population of Paramecium aurelia in Petri dish A may have shown an initial growth, reaching a peak and then stabilizing or declining over time.
- Population B (Paramecium caudatum and Paramecium aurelia): The population dynamics in Petri dish B may have exhibited competition between Paramecium caudatum and Paramecium aurelia. Observe whether one species dominates and causes the decline or extinction of the other species, or if a balance is reached.
- Population C (Paramecium caudatum only): The population of Paramecium caudatum in Petri dish C may have shown growth over time, potentially reaching a stable population size.
By analyzing and describing the population dynamics in each Petri dish, you can draw conclusions about the impact of competition and coexistence between Paramecium aurelia and Paramecium caudatum in different conditions.
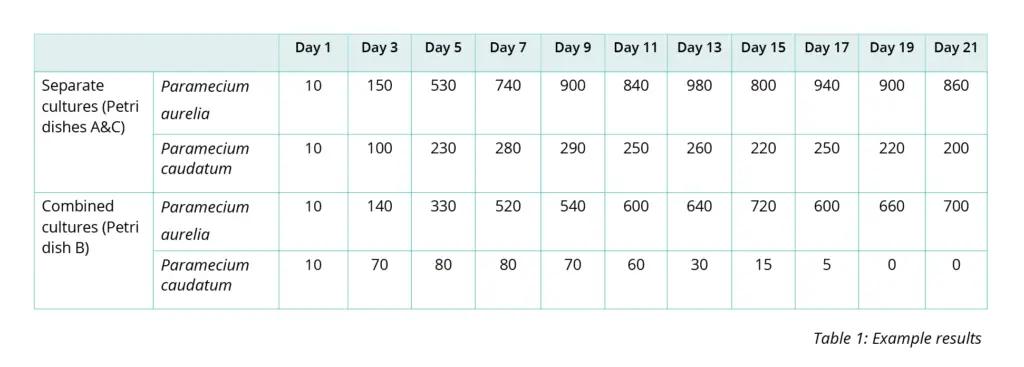
Investigations
- Hypothesis Evaluation: Evaluate whether your hypothesis was supported or not based on the observed results. Compare the expected outcomes stated in your hypothesis with the actual outcomes observed in the experiment. Provide reasons for the similarities or differences between the expected and observed results. For example, if your hypothesis predicted that Paramecium caudatum would outcompete Paramecium aurelia in mixed cultures (B), but the results showed a different trend, discuss the possible factors that might have influenced the outcome.
- Advantages and Disadvantages of Counting Technique: Discuss the advantages and disadvantages of the counting technique used to measure the population sizes of Paramecium. Some advantages might include its simplicity, cost-effectiveness, and ability to provide quantitative data. On the other hand, disadvantages could include potential inaccuracies due to human error in counting or subjective interpretation of what constitutes a viable Paramecium. Discuss the limitations of the technique and how it might affect the reliability and validity of the results.
- Limitations in Experiment Design and Suggestions for Improvement: Identify any limitations in the experimental design that could have influenced the results or introduced confounding variables. For example, potential limitations could include the lack of replication, absence of controls, or variations in environmental conditions between the Petri dishes. Suggest improvements to address these limitations, such as increasing the sample size, incorporating control groups, or maintaining more consistent environmental conditions across all cultures.
- Comparison of Population Sizes: Compare the population sizes of each Paramecium species in the separated cultures (A and B) and mixed culture (C). Analyze the results and discuss how competition affected population growth. Identify any patterns or trends observed in the population sizes and relate them to the presence or absence of competition between the species. For instance, if the population size of one species decreased in the presence of the other, it suggests that competition negatively affected its growth.
- Evaluation of the Competitive Exclusion Principle: Evaluate whether your results support or refute the competitive exclusion principle. Provide evidence from your findings to support your conclusion. If the results show that one species dominated and caused the decline or extinction of the other species in the mixed culture, it supports the competitive exclusion principle. Conversely, if both species were able to coexist and maintain stable populations in the mixed culture, it may challenge the principle.
- Comparison of Class Results: Compare your own results with the results obtained by other groups in the class. Identify any differences or similarities between your findings and those of your peers. Discuss possible reasons for the variations, such as different experimental conditions, techniques, or biological variations. Analyze the implications of the differences or similarities in the class results and how they may contribute to a broader understanding of competition and population dynamics.
By addressing these investigative questions, you can critically evaluate the experiment, its outcomes, and its implications for understanding the dynamics of Paramecium populations and competition.
Field examples of Gause’s Principle
Gause’s Principle, also known as the Competitive Exclusion Principle, suggests that two species with similar ecological niches and competing for the same limited resources cannot coexist indefinitely. Here are some field examples that illustrate Gause’s Principle:
- Darwin’s Finches: The finches in the Galapagos Islands studied by Charles Darwin provide a classic example of Gause’s Principle. Different species of finches have evolved to occupy different ecological niches based on their beak sizes and shapes, which allow them to exploit different food sources. When two species of finches overlap in their ecological niches, competition for food becomes intense, leading to the dominance of one species over the other.
- Anolis Lizards: Anolis lizards are found in various ecosystems across the Americas. In communities where multiple Anolis species coexist, researchers have observed that each species occupies different microhabitats, such as different heights in trees or different branches. This spatial separation allows them to reduce competition for resources like food and perching sites, thereby adhering to Gause’s Principle.
- Warblers: MacArthur’s warblers, studied by ecologist Robert MacArthur, provide an example of coexistence within the framework of Gause’s Principle. Different species of warblers that inhabit the same forest have been observed to occupy different vertical strata within the tree canopy. Each species feeds on different insects found in specific canopy layers, thus minimizing competition for food and coexisting in the same area.
- Antelope Species: In African savannas, multiple species of antelopes, such as impalas, gazelles, and kudus, inhabit the same grassland habitats. These species have evolved different adaptations to feed on grasses at different heights or utilize different foraging strategies. By partitioning resources and reducing competition, these antelope species are able to coexist and maintain stable populations.
- Plankton Communities: In aquatic ecosystems, plankton communities provide an interesting example that challenges Gause’s Principle. Despite limited resources such as sunlight and nutrients, numerous plankton species coexist within the same water column. This is known as the “paradox of the plankton.” Various factors, including niche differentiation, temporal and spatial variations in resource availability, and predator-prey dynamics, contribute to the coexistence of multiple plankton species, defying the predictions of competitive exclusion.
These field examples demonstrate the complexities of ecological interactions and the factors that influence species coexistence. While Gause’s Principle provides a valuable framework for understanding competition and its implications for population dynamics, real-world ecosystems often exhibit more intricate dynamics that involve a combination of competitive interactions, resource partitioning, and other ecological mechanisms.
Importance of Gause’s Principle
Gause’s Principle, also known as the Competitive Exclusion Principle, is of significant importance in the field of ecology. Here are some key reasons why Gause’s Principle holds importance:
- Understanding Species Interactions: Gause’s Principle helps us comprehend the dynamics of species interactions, particularly competition between species. It highlights the role of competition in shaping ecological communities and influencing species distributions. By understanding the competitive relationships between species, we can gain insights into the factors that structure ecosystems.
- Predicting Species Coexistence: Gause’s Principle provides a framework for predicting the outcome of species interactions in resource-limited environments. It suggests that two species with similar ecological requirements and competing for the same resources cannot coexist indefinitely. This principle aids in predicting which species will dominate a given habitat and which species are more likely to go extinct or be excluded.
- Ecological Conservation and Management: The Competitive Exclusion Principle has practical applications in ecological conservation and management. By recognizing the competitive interactions between species, conservation efforts can focus on preserving habitat diversity and minimizing the impacts of invasive species. Understanding the potential for competitive exclusion can help guide the restoration of degraded ecosystems and inform the management of species at risk.
- Evolutionary Processes: Gause’s Principle has implications for evolutionary processes, particularly in the context of resource specialization and niche differentiation. It highlights the importance of evolutionary adaptations that allow species to exploit different resources or occupy different ecological niches, reducing competition and promoting coexistence.
- Research and Experimental Design: Gause’s Principle has shaped the design of ecological experiments and studies. It has influenced the selection of study organisms, experimental setups, and data analysis approaches. By considering competitive interactions and potential outcomes based on Gause’s Principle, researchers can design experiments to test hypotheses related to species coexistence and competition.
- Education and Scientific Understanding: Gause’s Principle is a fundamental concept taught in ecology and biology courses. It helps students develop a deeper understanding of the mechanisms underlying species interactions and population dynamics. By learning about Gause’s Principle, individuals gain insights into the complexities of natural ecosystems and the factors that govern the distribution and abundance of species.
In summary, Gause’s Principle is important for understanding species interactions, predicting species coexistence, guiding ecological conservation and management efforts, studying evolutionary processes, and fostering scientific understanding of ecological dynamics. It serves as a foundational concept in ecology and has practical applications in various fields related to biodiversity and ecosystem functioning.
FAQ
What is Gause’s Principle?
Gause’s Principle, also known as the Competitive Exclusion Principle, states that two species competing for the same limited resources cannot coexist indefinitely. One species will outcompete and eventually drive the other species to extinction in a given ecological niche.
Who formulated Gause’s Principle?
Gause’s Principle was formulated by Russian biologist Georgy Gause based on his laboratory experiments with Paramecium species in the 1930s.
What does Gause’s Principle imply about species coexistence?
Gause’s Principle suggests that species with similar ecological requirements and competing for the same resources cannot stably coexist in the long term. The better adapted species will eventually exclude or outcompete the other species.
Are there any exceptions to Gause’s Principle?
While Gause’s Principle provides a useful framework, there are exceptions observed in natural ecosystems. Some communities exhibit species coexistence despite competing for the same resources. This is often attributed to niche differentiation, resource partitioning, or other factors that reduce competition.
How does Gause’s Principle relate to biodiversity?
Gause’s Principle has implications for biodiversity as it helps explain the observed patterns of species distribution and abundance in ecosystems. It highlights the importance of resource availability and competition in shaping species diversity.
Can Gause’s Principle be applied to real-world ecological systems?
Gause’s Principle provides a theoretical understanding of species interactions, but its direct application to complex ecological systems is challenging. Real-world ecosystems involve numerous interacting factors, such as predation, mutualism, and environmental variability, which can influence species coexistence.
How is Gause’s Principle relevant to conservation biology?
Gause’s Principle is relevant to conservation biology as it highlights the potential impacts of invasive species on native biodiversity. Understanding competitive interactions can help predict the outcomes of species introductions and guide management strategies to protect vulnerable species.
Does Gause’s Principle apply only to competition for resources?
Gause’s Principle primarily applies to competition for resources, as it was initially formulated based on resource competition experiments. However, it can also extend to other forms of competition, such as competition for space, mates, or other limiting factors necessary for survival and reproduction.
Can Gause’s Principle be tested experimentally?
Yes, Gause’s Principle can be tested experimentally using laboratory or field studies. Researchers can manipulate resource availability, introduce competing species into controlled environments, and monitor their interactions over time to determine if competitive exclusion occurs.
How does Gause’s Principle contribute to our understanding of ecological communities?
Gause’s Principle is a foundational concept in ecology that helps us understand the structure and dynamics of ecological communities. It provides insights into the processes that shape species distributions, coexistence patterns, and community assembly, contributing to our broader understanding of ecosystems.
References
- Kneitel, Jamie. (2013). Gause’s Competitive Exclusion Principle. 10.1016/B978-0-12-409548-9.00816-2.
- Kneitel, J. M. (2018). Gause’s Competitive Exclusion Principle ☆. Reference Module in Earth Systems and Environmental Sciences. doi:10.1016/b978-0-12-409548-9.00816-2
- http://faculty.washington.edu/stevehar/hardin1960science.pdf
- https://surendranathcollege.ac.in/new/upload/TARIQUL_ISLAM_GOLDARGause%60s%20Principle2021-02-12Gause%60s%20Principle.pdf
- http://www.kaliganjgovtcollege.ac.in/studyMaterial/0232competition-exclution.pdf
- https://biologywise.com/competitive-exclusion-principle-explained-with-examples
- https://www.southernbiological.com/competitive-exclusion-principle/
- https://www.lemonade-ed.com/level-2/2-6-ecological-patterns/fundamentals-of-ecology
- https://www.lkouniv.ac.in/site/writereaddata/siteContent/202004080628302359ashish_zool_Ecology_2.pdf
- http://harennotes4u.blogspot.com/2016/11/lotka-volterra-gauses-principleresource.html
- https://www.toppr.com/ask/question/state-gauses-competitive-exclusion-principle/
- https://users.wfu.edu/silmanmr/bio377/assignments/Readings/ExperimentalDesign/Diamond.pdf