What do you mean by Fate of Pyruvate?
Pyruvate, the end product of glycolysis, represents a central intermediate in cellular metabolism. Glycolysis is a sequence of enzyme-catalyzed reactions in which glucose is broken down into two molecules of pyruvate, generating ATP and NADH in the process. This pathway, also known as the Embden-Meyerhof-Parnas (EMP) pathway, occurs in the cytoplasm of cells and serves as a critical energy-producing mechanism.
In aerobic conditions, pyruvate undergoes oxidative decarboxylation in the mitochondria to form acetyl-CoA. This reaction is catalyzed by the pyruvate dehydrogenase complex and links glycolysis to the citric acid cycle, where acetyl-CoA is further metabolized to generate additional ATP through oxidative phosphorylation. Along with energy production, this pathway also provides precursors for biosynthetic processes.
Under anaerobic conditions, pyruvate is reduced to lactate through lactate dehydrogenase, regenerating NAD+ required for glycolysis to continue. This process is especially important in cells with limited oxygen supply, such as during intense muscle activity. In some microorganisms, pyruvate can also be converted into ethanol and carbon dioxide during fermentation.
The role of pyruvate extends beyond energy production. It serves as a key metabolite for gluconeogenesis, the process of glucose synthesis, and can be converted into amino acids like alanine. Its versatile metabolic fates highlight its significance in maintaining cellular energy balance and metabolic flexibility.
Overall, the fate of pyruvate depends on cellular oxygen availability and metabolic requirements, making it a crucial node in the regulation of energy and biosynthetic pathways.
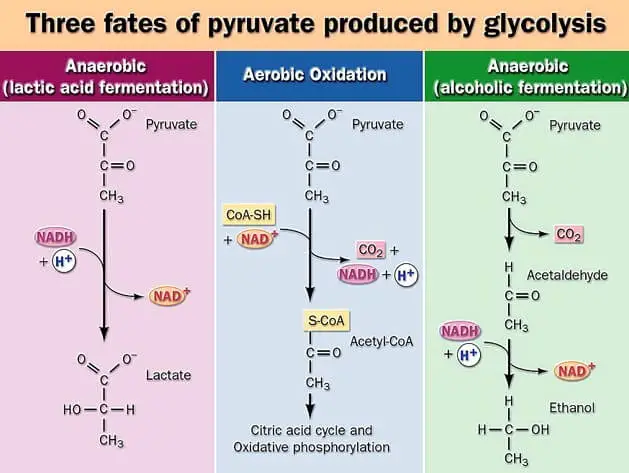
What are the Products of Glycolysis?
Glycolysis is a fundamental metabolic pathway in which glucose is broken down into smaller molecules, generating essential products for cellular energy. The major products formed during glycolysis include ATP, NADH, and pyruvate. These molecules are crucial for various cellular processes and energy production. Below is an overview of each product generated during glycolysis:
- ATP (Adenosine Triphosphate)
- ATP is the primary energy carrier in cells, often referred to as the “energy currency” of the cell.
- During glycolysis, ATP is produced in two key reactions:
- The oxidation of G-3-P (glyceraldehyde-3-phosphate) by G-3-P dehydrogenase leads to the addition of a high-energy phosphate group to ADP, forming ATP.
- The dehydration of 2-phosphoglycerate creates a high-energy phosphate bond, which is then transferred to ADP, forming another ATP.
- ATP is essential for energy transfer, fueling processes such as muscle contraction, protein synthesis, and active transport across cell membranes.
- NADH (Nicotinamide Adenine Dinucleotide)
- NADH is produced when NAD+ (Nicotinamide adenine dinucleotide) is reduced during the oxidation of glyceraldehyde-3-phosphate to 1,3-bisphosphoglycerate. This step is catalyzed by glyceraldehyde-3-phosphate dehydrogenase.
- NADH acts as a carrier of electrons in cellular respiration. It donates electrons to the electron transport chain (ETC), which leads to the generation of ATP through oxidative phosphorylation.
- From each NADH molecule produced, approximately 2.5 ATP molecules are generated in the ETC.
- Pyruvate (Pyruvic Acid)
- Pyruvate is the end product of glycolysis, formed when phosphoenolpyruvate transfers a phosphate group to ADP in a reaction catalyzed by pyruvate kinase.
- Two molecules of pyruvate are produced from one molecule of glucose.
- Pyruvate serves as a crucial intermediate in cellular metabolism. In aerobic conditions, it is transported to the mitochondria and converted into acetyl-CoA, which enters the Krebs cycle for further ATP production. In anaerobic conditions, pyruvate is reduced to lactate in muscles or converted into ethanol and CO2 in yeast and other microorganisms.
Summary Table of Glycolysis Products
Product | Formation Process | Role in Cellular Metabolism | Key Features |
---|---|---|---|
ATP | – Produced during substrate-level phosphorylation. | – Primary energy currency for the cell. – Powers active transport, muscle contraction, biosynthesis, and other cellular processes. | – High-energy molecule. – Hydrolysis releases 7.3 kcal/mol energy. – Water-soluble organic compound. |
NADH | – Formed during oxidation of glyceraldehyde-3-phosphate by glyceraldehyde-3-phosphate dehydrogenase. | – Electron donor in the electron transport chain. – Facilitates ATP generation during oxidative phosphorylation. | – Reduced form of NAD+. – One molecule yields ~2.5 ATP in the ETC. – Essential coenzyme for redox reactions. |
Pyruvate | – Produced by the final transfer of a phosphate group from phosphoenolpyruvate to ADP via pyruvate kinase. | – End product of glycolysis. – Precursor for acetyl-CoA, gluconeogenesis, and amino acid synthesis. – Fuel for the Krebs cycle. | – Three-carbon molecule. – Water-soluble. – Contains carboxylic and ketone functional groups. |
Fate of Pyruvate
A. Fate of Pyruvate in Presence of oxygen
When oxygen is available, pyruvate undergoes further biochemical transformations to enter the pathways that generate energy efficiently. These pathways ensure complete oxidation of glucose-derived carbons, maximizing ATP yield.
Key Pathways for Pyruvate in Aerobic Conditions
\text{Pyruvate} + \text{CoA-SH} + \text{NAD}^+ \xrightarrow{\text{Pyruvate Dehydrogenase Complex}} \text{Acetyl CoA} + \text{CO}_2 + \text{NADH}- Conversion to Acetyl-CoA
- Pyruvate is converted into Acetyl-CoA within the mitochondrial matrix.
- This process is catalyzed by the pyruvate dehydrogenase complex (PDC), which is composed of three enzymes (E1, E2, and E3).
- Five coenzymes are required: TPP (Thymine Pyrophosphate), FAD (Flavin Adenine Dinucleotide), Lipoate, CoA-SH, and NAD+.
- E1 (pyruvate dehydrogenase) binds pyruvate, leading to decarboxylation and the release of carbon dioxide (CO₂).
- The remaining acetyl group is attached to CoA-SH by the action of E2 (dihydrolipoyl transacetylase) in combination with TPP and Lipoate.
- E3 (dihydrolipoyl dehydrogenase) facilitates the transfer of hydrogen from Lipoate to FAD, and FAD transfers electrons to NAD+, forming NADH + H⁺.
- The result is Acetyl-CoA, which enters the TCA cycle for complete oxidation.
- Conversion to Oxaloacetate
- Pyruvate can also be converted into oxaloacetate through a carboxylation reaction.
- The enzyme pyruvate carboxylase catalyzes this reaction, requiring Biotin and Mg²⁺ ions as cofactors.
- This process consumes one ATP molecule.
- It replenishes TCA cycle intermediates when their levels drop.
- It serves as the starting substrate for gluconeogenesis, ensuring glucose can be synthesized when needed.
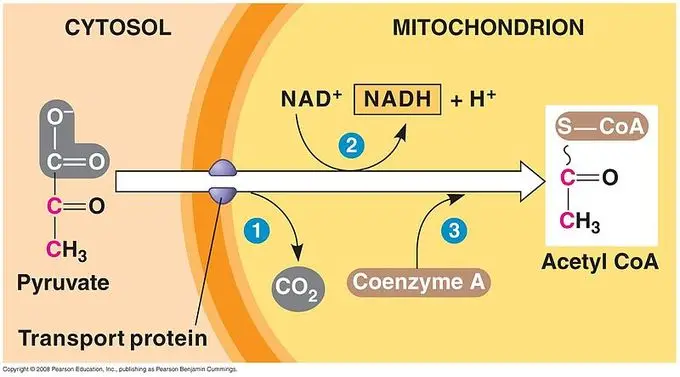
B. Fate of Pyruvate in Absence of oxygen
In the absence of oxygen, cells must rely on anaerobic pathways to continue generating ATP. Without oxygen, pyruvate cannot enter the mitochondria for complete oxidation via the TCA cycle, so it is diverted into alternative pathways that regenerate NAD+ to keep glycolysis running.
Key Pathways for Pyruvate in Anaerobic Conditions
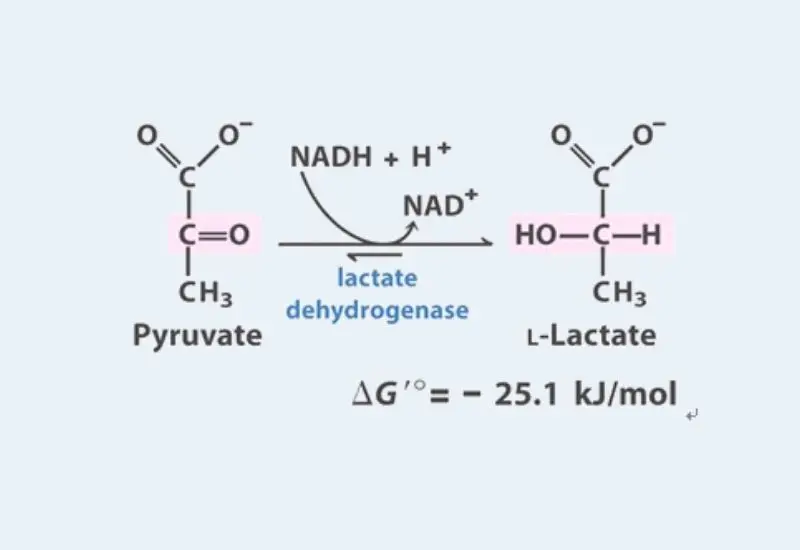
- Ethanol Formation (Fermentation in Yeast and Microorganisms)
- Pyruvate undergoes decarboxylation to form acetaldehyde in a reaction catalyzed by pyruvate decarboxylase.
- This step requires the presence of TPP (Thiamine Pyrophosphate) and Mg²⁺ ions.
- Acetaldehyde is then reduced to ethanol in a reaction catalyzed by alcohol dehydrogenase.
- Byproducts:
- CO₂ is released in the first step.
- NADH is oxidized to NAD+ in the second step.
- This process is vital in organisms like yeast, where it enables ATP production under anaerobic conditions.
Pyruvate ⇌ Acetaldehyde + CO2
Acetaldehyde + NADH/H+ ⇌ Ethanol + NAD+
Overall: Pyruvate + NADH/H+ ⇌ Ethanol + CO2 + NAD+
- Lactate Formation (Fermentation in Animals and Microbes)
- In tissues such as muscle cells during intense activity or in certain microbes, pyruvate is reduced to lactate.
- This reaction is catalyzed by lactate dehydrogenase.
- During lactate formation, NADH is oxidized back to NAD+, allowing glycolysis to continue.
- Lactate accumulation occurs in conditions where oxygen is limited, such as during vigorous exercise.
- Byproduct: The formation of lactate helps regenerate NAD+, enabling glycolysis to proceed.
Biosynthetic Pathway
- Pyruvate can further infiltrate into the biosynthetic pathways for example fatty acids biosynthesis and gluconeogenesis.
- Pyruvate converted into acetyl CoA by the activity of pyruvate dehydrogenase complex this acetyl CoA further enters into the biosynthetic pathway alongside TCA.
- Pyruvate can further infiltrate into gluconeogenesis by the activity of pyruvate carboxykinase transforming it into oxaloacetate which with several steps reaction transforms into glucose. When the glucose level of cells decreased they activate the gluconeogenesis pathway to generate glucose from Pyruvate.
Factors Influencing Pyruvate’s Fate
The metabolic fate of pyruvate is influenced by a combination of factors that dictate its conversion into various metabolites. Understanding these factors helps explain how pyruvate plays a central role in cellular energy production and metabolic regulation. Here’s a breakdown of the key influences:
- Oxygen Availability
- Aerobic Conditions: In the presence of oxygen, pyruvate is typically converted into acetyl-CoA. This enters the tricarboxylic acid (TCA) cycle, which drives ATP production through oxidative phosphorylation. This pathway is crucial for efficient energy generation in oxygen-rich tissues like the heart and brain.
- Anaerobic Conditions: When oxygen is limited, pyruvate undergoes fermentation. In animal cells, this results in lactate, while in yeast, pyruvate is converted into ethanol and carbon dioxide. This shift ensures the continuation of glycolysis by regenerating NAD+, which is necessary to maintain ATP production when oxidative phosphorylation is restricted.
- Enzymatic Regulation
- Pyruvate Dehydrogenase: This enzyme catalyzes the conversion of pyruvate into acetyl-CoA, a step that is crucial for entering the TCA cycle. Its activity is influenced by NAD+ availability and the presence of coenzyme A, both of which regulate the efficiency of this conversion.
- Pyruvate Carboxylase: This enzyme facilitates the conversion of pyruvate into oxaloacetate, particularly important in gluconeogenesis. The activity of pyruvate carboxylase is regulated by acetyl-CoA levels, which can signal the energy status of the cell.
- Lactate Dehydrogenase: Under anaerobic conditions or during intense physical activity, this enzyme converts pyruvate into lactate. This reaction helps regenerate NAD+ to keep glycolysis functioning when oxygen is scarce.
- Nutritional Status and Hormonal Regulation
- Fasting and Feeding: The metabolic fate of pyruvate is influenced by the body’s nutritional state. During fasting, increased glucagon levels promote gluconeogenesis, directing pyruvate toward glucose production. After eating, insulin levels rise, enhancing glycolysis and promoting pyruvate conversion into energy or storage.
- Insulin: Insulin plays a direct role in glucose uptake and its conversion into pyruvate, influencing whether pyruvate will be used for immediate energy production or stored as fat.
- Cellular Energy Demand
- The energy demands of the cell determine how pyruvate is utilized. When the cell needs more ATP, pyruvate is directed toward the TCA cycle for acetyl-CoA production. In contrast, when energy demand is low, pyruvate may be converted into lactate to regenerate NAD+ and allow glycolysis to continue without relying on oxygen.
- Genetic and Molecular Factors
- Genetic mutations in enzymes involved in pyruvate metabolism can lead to disruptions in normal cellular function. For example, mutations in mitochondrial pyruvate carriers can interfere with pyruvate’s proper conversion and utilization, contributing to metabolic disorders, cancer, or neurodegeneration.
- Environmental Factors
- External conditions such as temperature, pH, and the availability of cofactors like NAD+ and ATP can impact enzyme activity and, consequently, pyruvate’s fate. The presence or absence of certain cofactors can enhance or inhibit specific metabolic pathways, dictating whether pyruvate is converted to acetyl-CoA, lactate, or other metabolites.
- Gray LR, Tompkins SC, Taylor EB. Regulation of pyruvate metabolism and human disease. Cell Mol Life Sci. 2014 Jul;71(14):2577-604. doi: 10.1007/s00018-013-1539-2. Epub 2013 Dec 21. PMID: 24363178; PMCID: PMC4059968.
- Aaron D. Freedman, Leonard Kohn, Pyruvate Metabolism and Control: Factors Affecting Pyruvic Carboxylase Activity.Science145,58-60(1964).DOI:10.1126/science.145.3627.58
- KOEPPE RE, MOURKIDES GA, HILL RJ. Some factors affecting routes of pyruvate metabolism in rats. J Biol Chem. 1959 Sep;234:2219-22. PMID: 14410462.
- https://bio.libretexts.org/Bookshelves/Microbiology/Microbiology_(Boundless)/05:_Microbial_Metabolism/5.03:_Catabolism/5.3B:_Pyruvic_Acid_and_Metabolism
- https://reactome.org/content/detail/R-HSA-70268
- https://pmc.ncbi.nlm.nih.gov/articles/PMC4059968/
- https://pubchem.ncbi.nlm.nih.gov/pathway/Reactome:R-HSA-70268
- https://www.sciencedirect.com/topics/pharmacology-toxicology-and-pharmaceutical-science/pyruvic-acid
- https://www.journalofdairyscience.org/article/S0022-0302(64)88927-X/pdf
- https://www.mdpi.com/2218-273X/10/7/1068
- https://www.sciencedirect.com/science/article/pii/S002192581869787X/pdf?md5=3f5acb008c02f535197d4b241aa4f4a5&pid=1-s2.0-S002192581869787X-main.pdf
- https://www.science.org/doi/10.1126/science.145.3627.58
- https://en.wikipedia.org/wiki/Pyruvate