What is dna repair mechanism?
DNA repair is a collection of processes by which a cell identifies and corrects damage to the DNA molecules that encode its genome.
dna repair definition
- One or two genomic DNA sets are typically present in each cell. The DNA’s information may be used to quickly replace broken proteins and RNA molecules, but DNA molecules cannot be repaired.
- A complex system of DNA repair mechanisms supports the cellular necessity of maintaining the integrity of the information in DNA.
- Numerous mechanisms, some spontaneous and others triggered by environmental factors, can degrade DNA.
- The informational content of DNA can occasionally be harmed by replication itself if errors introduce mismatched base pairs (such as G paired with T).
- DNA damage has a diverse and intricate chemistry. Numerous enzyme systems that catalyze some of the most fascinating chemical changes in DNA metabolism are part of the cellular response to this damage.
- We first consider the results of DNA sequence changes before thinking about particular repair mechanisms.
Types of dna repair mechanism
There are present four types of DNA Repair System such as;
- Mismatch Repair
- Base-excision repair
- Nucleotide-excision repair
- Direct repair
1. Mismatch Repair System
- Correction of the few mismatches remaining after replication in E. coli increases the overall replication fidelity by a factor of 10^2 to 10^3.
- The mismatches are almost always fixed to reflect the information in the old (template) strand; hence, the repair machinery must distinguish between the template and the newly synthesised strand.
- The cell achieves this by attaching methyl groups to the template DNA to distinguish it from newly produced strands.
- E. coli’s mismatch repair system consists of at least 12 protein components that function either in strand separation or in the repair process itself.
- The mechanism underlying strand discrimination in the majority of bacteria and eukaryotes has not been elucidated, however it is well understood in E. coli and certain closely related bacteria.
- Dam methylase, which, as you may recall, methylates DNA at the Af location of all adenines inside (5′)GATC sequences, is responsible for strand discrimination in these bacteria.
- There is a brief interval (a few seconds or minutes) following the passing of the replication fork during which the template strand is methylated but the freshly synthesised strand is not.
- The temporary unmethylated status of GATC sequences in the freshly synthesised strand distinguishes the newly synthesised strand from the template strand.
- Replication mismatches in the area of a hemimethylated GATC sequence are subsequently repaired using information from the methylated parent strand (template).
- If both strands are methylated at a GATC sequence, few mismatches are corrected; if neither strand is methylated, mismatches are mended but neither strand is favoured.
- From a hemimethylated GATC sequence, the methyl-directed mismatch repair machinery of the cell efficiently repairs mismatches of up to 1,000 base pairs.
Enzyme participate in dna repair process – Mismatch repair
Enzymes/protein | Type of damage |
Dam methylase | |
DNA ligase | |
RecJ nuclease | |
Exonuclease X | |
Exonuclease VII | Mismatches |
Exonuclease I | |
DNA Polymerase III | |
SSB | |
DNA helicase II | |
MutH, MutL, MutS proteins |
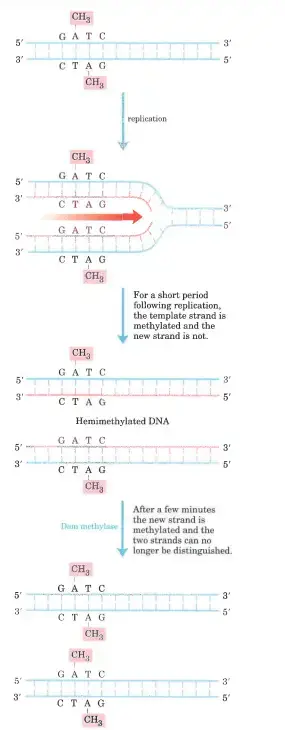
How is the mismatch correction process directed by relatively distant GATC sequences?
- MutL protein combines with MutS protein to produce a complex that binds all mismatched base pairs (except C-C).
- MutH protein binds to MutL and to GATC sequences encountered by the MutL-MutS complex. DNA on both sides of the mismatch is threaded through the MutL-MutS complex, forming a DNA loop.
- MutH has site-specific endonuclease activity that is inactive until a hemimethylated GATC sequence is encountered. At this location, MutH catalyses the cleavage of the unmethylated strand 5′ to the G in GATC, marking it for repair.
- The next steps in the pathway rely on the location of the mismatch relative to the cleavage site.
- When the mismatch is on the 5′ side of the cleavage site, the unmethylated strand is unravelled and destroyed from the cleavage site through the mismatch, and this section is replaced by new DNA.
- This procedure needs the concerted efforts of DNA helicase II, SSB, exonuclease I or exonuclease X (both of which degrade DNA strands in the 3′-5′ direction), DNA polymerase III, and DNA ligase.
- Exonuclease VII degrades single-stranded DNA in the 5′-3′ or 3′-5′ direction, whereas RecJ nuclease degrades single-stranded DNA in the 5-3′ direction.
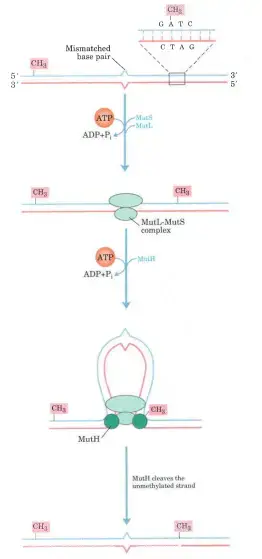
Features of Mismatch Repair System
- E. coli has to use a lot of energy to fix mismatches, which is a very expensive process.
- The difference could be at least 1,000 base pairs away from the GATC sequence.
- To fix a single mismatched base, it takes a lot of activated deoxynucleotide precursors to break down and replace a segment of this length of strand.
- This again shows how important genomic integrity is for the cell.
- There are several proteins in all eukaryotic cells that are structurally and functionally similar to the bacterial MutS and MutL proteins, but not to the MutH protein.
- Changes in human genes that code for these kinds of proteins cause some of the most common syndromes that make people more likely to get cancer. This shows again how important DNA repair systems are to the body.
- Most eukaryotes, from yeast to people, have MSH2, MSH3, and MSH6 as their main MutS homologs.
- Heterodimers of MSH2 and MSH6 usually bind to loops with only one mismatched base pair and bind less well to loops with two or more mismatched base pairs.
- In many organisms, the longer mismatches (2–6 bp) may be bound by a heterodimer of MSH2 and MSH3, or both types of heterodimers may bind to the mismatches at the same time.
- Homologs of MutL, which are mostly made up of MLHI (MutL homolog 1) and PMS1 (post-meiotic segregation), bind to MSH complexes and keep them stable.
- In eukaryotic mismatch repair, there are still a lot of details that need to be worked out. In particular, we don’t know how newly made DNA strands are recognised, though research has shown that it doesn’t have anything to do with the GATC sequence.
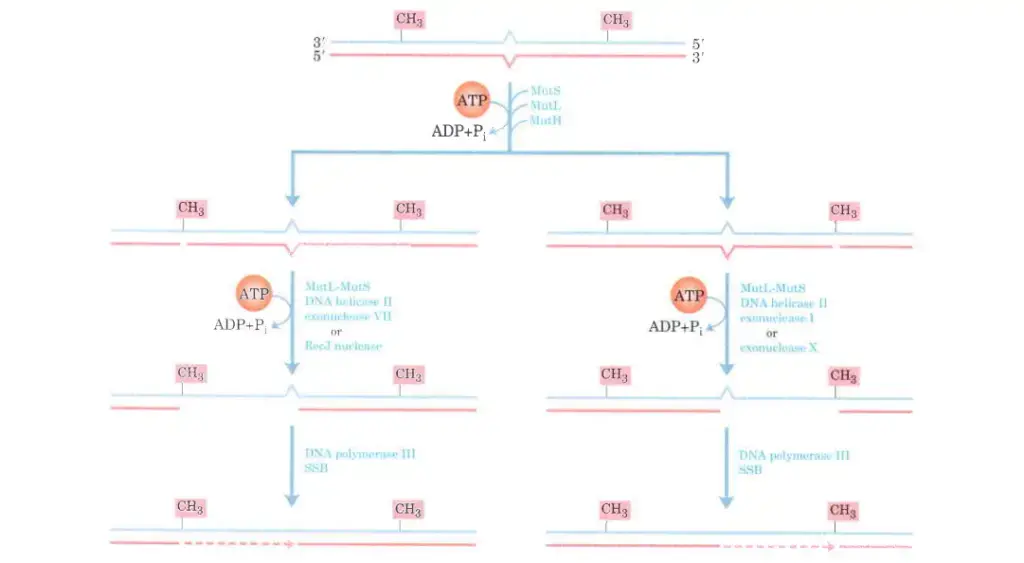
2. Base-Excision Repair
- DNA glycosylases are a type of enzyme that is found in every cell. These enzymes look for common DNA damage, like the products of cytosine and adenine deamination, and remove the damaged base by breaking the N-glycosyl bond.
- This cleavage makes an apurinic or apyrimidinic site, also called an AP site or an abasic site, in the DNA.
- Most of the time, each DNA glycosylase only works on one type of lesion.
- Uracil DNA glycosylases, which are found in most cells, remove the uracil from DNA that is made when cytosine breaks down on its own.
- A lot of G:C to A=T mutations happen in cells that have lost this enzyme. This enzyme doesn’t take out uracil or thymine residues from RNA or DNA.
- The ability to tell the difference between thymine and uracil, which comes from cytosine deamination and is needed for selective repair of uracil, may be one reason why DNA has thymine instead of uracil.
- Most bacteria only have one kind of uracil DNA glycosylase, but humans have at least four different kinds, which shows how important it is to get rid of uracil from DNA.
- The most common uracil glycosylase in humans is called UNG. It is found in the human replisome, where it gets rid of the occasional U residue that gets put in place of a T during replication.
- Deamination of C residues happens 100 times faster in single-stranded DNA than in double-stranded DNA, and humans have an enzyme called hSMUGl that gets rid of any U residues that happen during replication or transcription in single-stranded DNA.
- Two more human DNA glycosylases, TDG and MBD4, take out U or T residues that are paired with G. This happens when cytosine or 5-methylcytosine is deaminated.
- Other DNA glycosylases can find and get rid of different kinds of damaged bases, such as formamidopyrimidine and 8-hydroxyguanine, which come from purine oxidation, hypoxanthine, which comes from adenine deamination, and alkylated bases like 3-methyladenine and 7-methylguanine.
- Some types of organisms have also been found to have glycosylating enzymes that can recognise other types of damage, such as pyrimidine dimers.
- Remember that AP sites are also made when the N-glycosyl bonds in DNA slowly break down on their own.
- After a DNA glycosylase makes an AP site, it needs to be fixed by another type of enzyme.
- You can’t just put in a new base and make a new N-glycosyl bond to fix the problem. Instead, the deoxyribose 5′-phosphate that was left behind is taken away and a new nucleotide is put in its place.
- This process starts with one of the enzymes called AP endonucleases, which cuts the DNA strand at the AP site.
- Depending on the type of AP endonuclease, the incision can be made 5′ or 3′ from the AP site.
- The AP site is then cut out of a piece of DNA, which is replaced by DNA polymerase I, and the remaining nick is sealed by DNA ligase.
- In eukaryotes, nucleotide replacement is done by polymerases that are designed for this task.
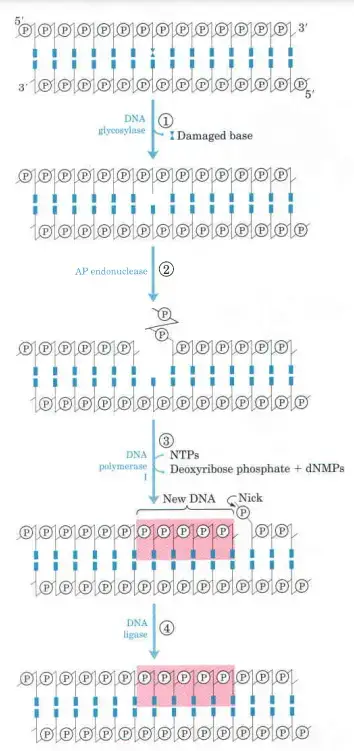
Enzyme participate in dna repair process – Base-Excision Repair
Enzyme and Proteins | Type of damage |
DNA glycosylases | |
AP endonucleases | Abnormal bases (uracil, hypoxanthine, xanthine) ; alkylated bases; in some other organisms, pyrimidine dimers |
DNA polymerase I | |
DNA ligase |
3. Nucleotide-Excision Repair
- The nucleotide-excision system is a repair pathway that is important for the survival of all free-living organisms. It fixes DNA lesions that cause big changes in the helical structure of DNA.
- In nucleotide-excision repair, a multisubunit enzyme called excinuclease breaks down two phosphodiester bonds, one on each side of the damage caused by the lesion.
- In E. coli and other bacteria, the enzyme system breaks the fifth phosphodiester bond on the 3′ side and the eighth phosphodiester bond on the 5′ side. This makes a fragment of 12 to 13 nucleotides (depending on whether the lesion involves one or two bases).
- In humans and other eukaryotes, the enzyme system breaks the sixth phosphodiester bond on the 3′ side and the twenty-second phosphodiester bond on the 5′ side. This makes a fragment of 27 to 29 nucleotides.
- After the double cut, the removed oligonucleotides are freed from the duplex, and DNA polymerase I in E. coli or DNA polymerase in humans fills in the gap. The nick is closed by DNA ligase.
- In E. coli, the ABC excinuclease is the most important enzyme complex. It is made up of three parts: UvrA (M.104,000), UwB (M.78,000), and UwC. (M.68,000).
- The name “excinuclease” comes from the fact that this enzyme complex is the only one that can catalyse two specific endonucleolytic cleavages. This makes it different from other endonucleases.
- A complex of the UwA and UwB proteins, called A2B, looks at the DNA and binds to a damaged spot.
- The UwA dimer then breaks apart, leaving a tight complex of UwB and DNA.
- The UwC protein then binds to UwB, and UwB cuts at the fifth phosphodiester bond on the 3′ side of the lesion. After this, a cut is made at the eighth phosphodiester bond on the 5′ side by UvrC.
- UvrD helicase then gets rid of the l2 to 13 nucleotide fragment that is left over.
- DNA polymerase I and DNA ligase then fill in the short gap that is left.
- This pathway is the main way that many types of DNA damage are fixed, such as cyclobutane pyrimidine dimers, 6-4 photoproducts, and several types of base adducts, such as benzo[o]pyrene-guanine, which is made in DNA when it is exposed to cigarette smoke.
- The ABC excinuclease has a unique nucleolytic activity in that it makes two cuts in the DNA.
- The way eukaryotic exonucleases work is similar to that of the bacterial enzyme, but the dual incision needs 16 polypeptides that have nothing in common with the E. coli excinuclease subunits.
- In eukaryotes, transcription is closely linked to both nucleotide-excision repair and base-excision repair. Humans with problems in their nucleotide excision repair genes are more likely to get a number of serious diseases.
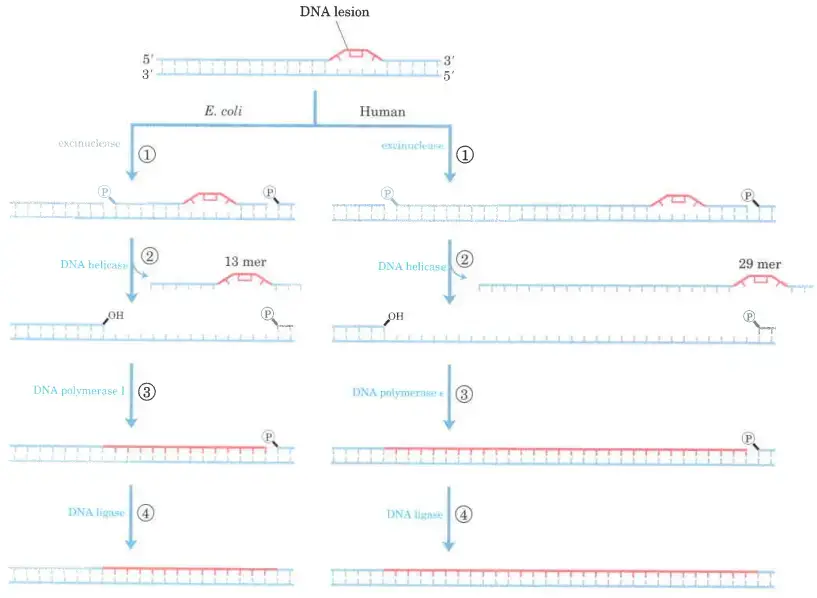
Enzyme participate in dna repair process – Nucleotide-Excision Repair
Enzymes/proteins | Type of damage |
ABC excinuclease | |
DNA polymerase I | DNA lesions that cause large structural changes (e.g., pyrimidine dimers) |
DNA ligase |
4. Direct Repair
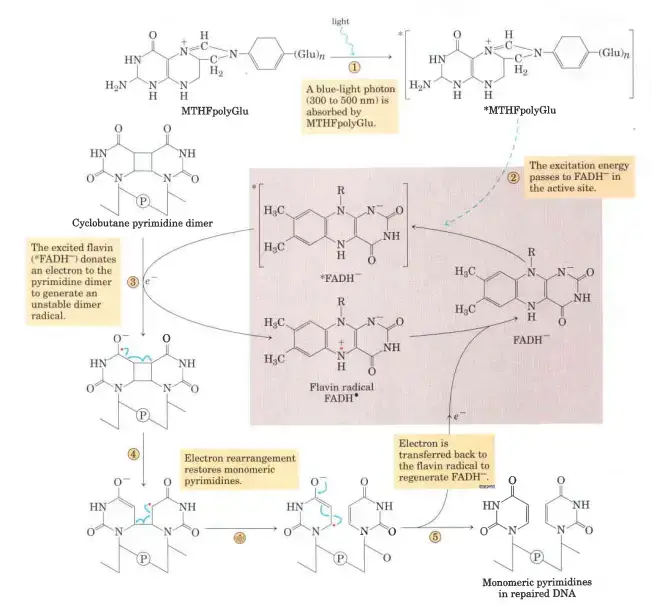
- There are different kinds of damage that can be fixed without taking out a base or nucleotide. DNA photolyases speed up the direct photoreactivation of cyclobutane pyrimidine dimers, which is the best-known example.
- UV light causes a reaction that makes pyrimidine dimers, and photolyases use the energy from light that has been absorbed to fix the damage.
- Most photolyases have two cofactors that act as chromophores, or substances that absorb light. FADH- is always one of the chromophores.
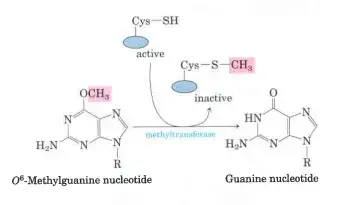
- The other colour in E. coli and yeast is a folate. As part of the way the reaction works, free radicals are made.
- DNA photolyases are not found in the cells of mammals that give birth in a placenta (which include humans). In the repair of nucleotides with alkylation damage, you can see more examples of this.
- When alkylating agents are present, the modified nucleotide O6-methylguanine is made. This is a common and very mutagenic lesion. lt tends to pair with thymine instead of cytosine during replication, which causes changes from G:C to A:T.
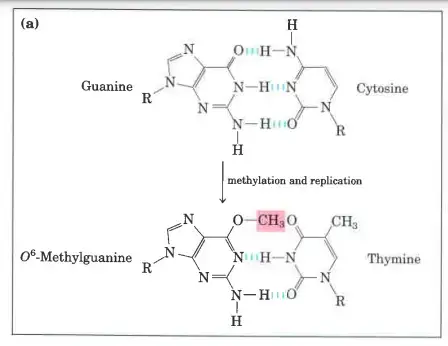
- O6-methylguanine-DNA methyltransferase is a protein that helps move the methyl group of O6-methylguanine to one of its own Cys residues. This is how direct repair of 06-methylguanine is done.
- This methyltransferase is not really an enzyme because a single methyl transfer permanently methylates the protein, making it inactive in this pathway.
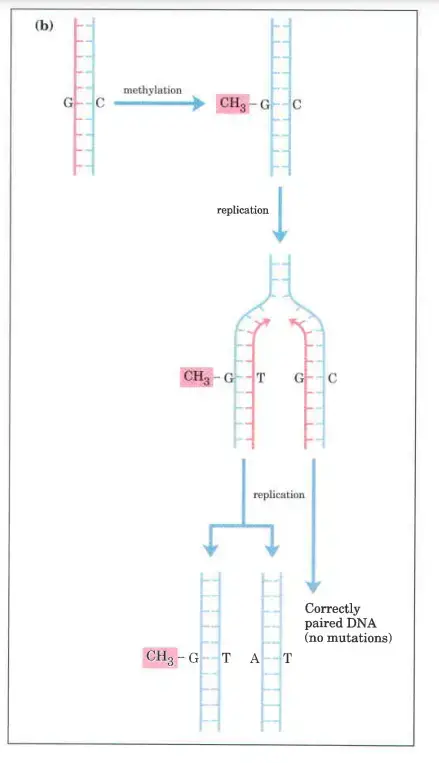
- Another clear example of how important it is to keep cellular DNA in good shape is that it takes an entire protein molecule to fix a single broken base.
- 1-methyladenine and 3-methylcytosine are fixed by a very different but still direct process.
- When the DNA is single-stranded, the amino groups of A and C residues are sometimes methylated. This has a direct effect on how well the bases pair.
- In E. coli, the AlkB protein, which is a member of the o-ketoglutarate-Fe2+-dependent dioxygenase superfamily, is in charge of the oxidative demethylation of these alkylated nucleotides.
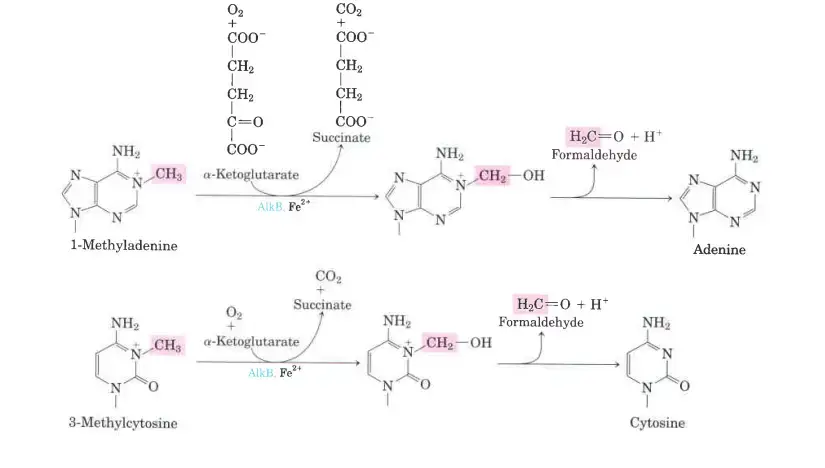
Enzyme participate in dna repair process – Direct Repair
Enzyme | Type of damage |
DNA photolyases | |
O6-Methylguanine-DNA methyltransferase | Pyrimidine dimers 06-Methylguanine |
AlkB protein | 1-Methylguanine, 3-methylcytosin |
the content of the subject matter is explained clearly to the point