What is Distant Hybridization (Wide hybridization)?
- Distant hybridization, also known as wide hybridization, involves crossing individuals from different species or genera that are more distantly related than varieties within the same species. This technique contrasts with standard hybridization, which typically involves crossing different varieties within a single species. In crop improvement programs, distant hybridization becomes essential when hybrid vigor or new traits are sought beyond the capabilities of intraspecific crosses.
- Interspecific hybridization occurs when individuals from two distinct species within the same genus are crossed. For example, crossing cultivated rice (Oryza sativa) with wild rice (Oryza perennis) illustrates this type of hybridization. On the other hand, intergeneric hybridization involves crossing individuals from different genera. An example is the hybridization between wheat (Triticum spp.) and rye (Secale cereale). Such crosses often yield hybrids with novel characteristics, though these are frequently less predictable and more challenging to manage.
- Distant hybridization can thus encompass both interspecific and intergeneric hybridization. This process is valuable in broadening genetic diversity and introducing new traits into crops. An early example of distant hybridization is the cross between carnation (Dianthus caryophyllus) and sweet William (Dianthus barbatus) conducted by Thomas Fairchild in 1717, known as Fairchild’s mule. Despite its limited agricultural value, this hybrid demonstrated the potential of distant crosses.
- In some cases, distant hybrids have been developed for specific applications. For instance, the intergeneric hybrid Raphanobrassica, created by Karpechenko from radish and cabbage, was an amphidiploid with limited practical use. Conversely, the development of Triticale, a hybrid of wheat and rye by Rimpu, represents a successful example with significant agricultural potential. Such hybrids can offer desirable traits such as improved disease resistance or better adaptability, underscoring the importance of distant hybridization in advancing crop science and agriculture.
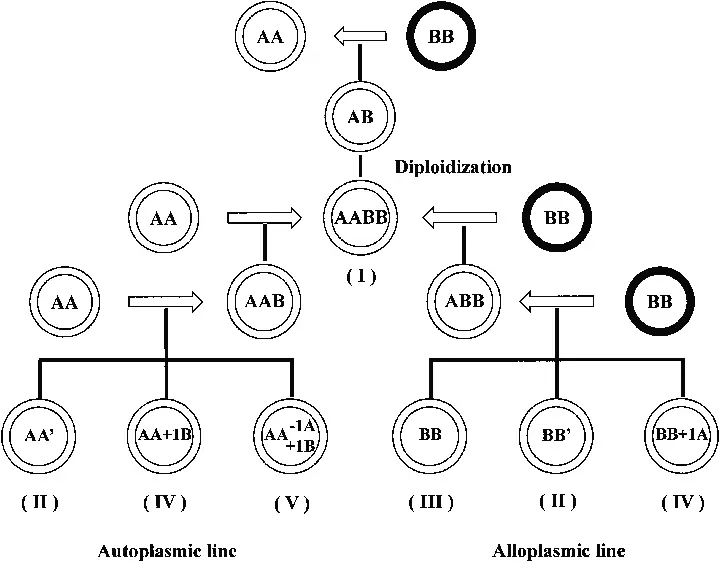
Definition of Distant Hybridization
Distant hybridization, or wide hybridization, refers to the crossing of individuals from different species or genera that are more distantly related than varieties within the same species, aiming to introduce novel traits and increase genetic diversity.
History of Distant Hybridization
- Early Developments
- The earliest documented case of distant hybridization dates back to 1717, when Thomas Fairchild successfully created a hybrid between the carnation (Dianthus caryophyllus) and sweet William (Dianthus barbatus). This hybrid, known as Fairchild’s mule, marked a significant milestone in the field, demonstrating the potential of crossing species from the same genus.
- Growth in Interspecific Hybridization
- Following Fairchild’s work, a considerable number of interspecific hybrids were produced. These hybrids involved crossing different species within the same genus. However, many of these hybrids had limited agricultural value and were primarily of academic interest. Despite this, several interspecific hybrids found commercial use, especially among ornamental plants.
- Notable Intergeneric Hybrids
- In 1928, Russian scientist Karpechenko developed an intergeneric hybrid known as Raphanobrassica. This amphidiploid resulted from a cross between radish (Raphanus sativus) and cabbage (Brassica oleracea). The goal was to combine the desirable root characteristics of radish with the leafy traits of cabbage. However, Raphanobrassica exhibited roots similar to cabbage and leaves similar to radish, proving to be of limited practical use.
- Introduction of Triticale
- Around 1890, the first successful intergeneric hybrid with significant potential was developed: Triticale. This amphidiploid hybrid resulted from crosses between wheat (Triticum spp.) and rye (Secale cereale). Triticale demonstrated considerable improvement in various characteristics and has since become a valuable crop with enhanced traits.
- Current Applications
- Today, distant hybridization plays a critical role in crop improvement programs. While interspecific hybridization has contributed to the development of specific crop species like sugarcane (Saccharum officinarum), distant hybridization is increasingly employed to transfer desirable genes, such as those for disease resistance, from wild relatives to cultivated crops. This approach has been crucial in enhancing crop resilience and ensuring food security.
- Impact on Agriculture
- The transfer of genes from wild species has proven indispensable in agricultural practices. As Jack Harlan noted, there are numerous instances where genes from wild relatives have been vital in preventing starvation and mitigating economic risks. Thus, distant hybridization remains a fundamental technique in advancing crop breeding and improving agricultural sustainability.
Objectives of Distant Hybridization
- Transfer of Desirable Traits
- One primary objective of distant hybridization is to introduce beneficial traits from wild relatives into cultivated varieties. For instance, genes for disease resistance and insect resistance, which are often present in wild species but absent in domesticated crops, can be transferred to improve the resilience of cultivated plants.
- Enhancement of Wide Adaptability
- Distant hybridization aims to develop crops with broader adaptability. Traits such as drought resistance and cold tolerance can be introduced to help crops thrive in diverse environmental conditions. This adaptation is crucial for maintaining agricultural productivity under varying climate scenarios.
- Improvement of Quality
- Another goal is the enhancement of quality attributes in crops. For example, the fiber quality in cotton can be improved through distant hybridization. This improvement in quality can lead to better performance and higher value products.
- Increase in Yield
- Yield enhancement is a significant objective of distant hybridization. Crops like oats, tobacco, maize, and sugarcane have benefited from this technique, leading to higher productivity and more efficient crop production.
- Modification of Other Traits
- Distant hybridization can also be used to modify various other characteristics. This includes introducing cytoplasmic male sterility (CMS) for hybrid seed production or altering morphological traits such as earliness and dwarfness, which can be advantageous for specific agricultural practices.
- Exploitation of Heterosis
- The exploitation of heterosis, or hybrid vigor, is another objective. This is particularly relevant in vegetatively propagated and ornamental crops, where increased growth rates and improved performance are desirable outcomes.
- Prolongation of Growth Periods
- Distant hybridization can be employed to extend the vegetative and blooming periods of crops. This prolongation can enhance the productivity and aesthetic value of plants, especially in ornamental horticulture.
- Creation of Novel Genotypes
- Finally, distant hybridization aims to create novel genotypes, including new species or F1 hybrids that do not naturally occur. These novel hybrids can offer unique combinations of traits and provide new options for crop breeding and cultivation.
Barriers to the Production of Distant Hybrids
The production of distant hybrids—crosses between species that are not closely related—can present significant challenges. These barriers can be broadly categorized into three main areas: failure of zygote formation, failure of zygote development, and failure of F1 seedling development. Understanding these barriers is essential for improving hybridization techniques and advancing plant breeding strategies.
- Failure of Zygote Formation
- Pollen Tube Incompatibility: One major barrier is the failure of the pollen tube to reach the embryo sac. This can occur if the pollen tube is unable to grow through the style of the female parent, as seen in some species combinations in Datura. Additionally, if the pollen tube is too short relative to the style, such as in crosses between maize (Zea mays) and Tripsacum species, fertilization fails. Solutions include using a shorter-style species as the female parent or modifying the style length.
- Polyploid-Diploid Incompatibility: When a diploid species is crossed with a polyploid species, the pollen tube of the polyploid may not grow effectively through the diploid style. This issue can be mitigated by using the diploid as the male parent or by shortening the style of the diploid female.
- Failure of Zygote Development
- Lethal Genes: Some species possess lethal genes that cause zygote death during early embryonic development. These genes are typically harmless within the parent species but detrimental in hybrids. For instance, Aegilops umbellulata contains a lethal gene affecting hybrids with diploid wheats. Similarly, Crepis tectorum has a lethal gene causing early embryo death in certain interspecific crosses. The issue can be managed by using strains of the parent species that do not carry the lethal allele.
- Genotypic Disharmony: Genetic imbalance between the genomes of the parental species can also lead to zygote death. This disharmony may sometimes be mistaken for lethal gene effects, as observed in cotton hybrids involving Gossypium species. While some cases have been linked to known lethal genes, others remain ambiguous. Endosperm abortion, where the endosperm does not develop properly, can be addressed by culturing hybrid embryos on suitable media before endosperm abortion occurs.
- Failure of Hybrid Seedling Development
- Chlorophyll Deficiency: Some distant hybrids may exhibit chlorophyll deficiency and fail to survive, as seen in certain Melilotus hybrids. Such plants may be grown to maturity through grafting onto normal plants or in vitro culture.
- Seedling Mortality: Interspecific hybrids, such as those in cotton, may appear normal initially but die at various stages of seedling development or after flowering. The mechanisms causing these issues are likely similar to those affecting embryo development, including lethal genes, genetic imbalance, and cytoplasmic incompatibility.
Techniques for the Production of Distant Hybrids
To achieve successful hybridization, various techniques are employed to overcome compatibility barriers and ensure successful fertilization. The following points outline these techniques:
- Choice of Parents
- Genetic Compatibility: Selecting compatible parent plants is crucial for successful hybridization. Genetic differences among species can impact cross compatibility. Therefore, choosing parents with greater genetic compatibility enhances the likelihood of successful crosses.
- Pollination Techniques
- Sufficient Pollination: Ensuring a large number of flowers are pollinated increases the chances of obtaining viable hybrids. Adequate pollination helps overcome potential fertilization barriers.
- Reciprocal Crosses
- Technique: Reciprocal crosses involve using both species in reverse roles (male and female). This approach can sometimes resolve incompatibility issues, as seen with Phaseolus aureus and P. mungo, where successful crosses occur only when P. aureus is used as the female parent.
- Manipulation of Ploidy
- Chromosome Number Adjustment: When crossing species with differing chromosome numbers, manipulating ploidy levels can be essential. Techniques include using a higher ploidy species as the female parent or doubling the chromosome number of the hybrid (F1) to address sterility issues.
- Bridge Crosses
- Intermediate Species: In cases where direct crosses between two species (e.g., species ‘A’ and ‘C’) are not possible, a third species (e.g., species ‘B’) that can cross with both ‘A’ and ‘C’ is used. This method facilitates gene transfer, as demonstrated in tobacco and wheat hybrids. For example, Nicotiana repanda and N. tabacum do not cross directly, but N. sylvestris, which can cross with both, serves as a bridge species.
- Use of Pollen Mixtures
- Overcoming Incompatibility: Cross incompatibility may arise from unfavorable interactions between pistil proteins and pollen. Using mixtures of pollen from compatible and incompatible parents can sometimes overcome this issue and promote successful fertilization.
- Manipulation of Pistil
- Adjusting Style Length: In species with long styles and short pollen tubes, fertilization can be hindered. Techniques such as cutting the style to a normal length or conducting reciprocal crosses can facilitate pollen tube penetration, as seen in maize × Tripsacum crosses.
- Growth Regulation
- Enhancing Pollen Tube Growth: Slow pollen tube growth can lead to fertilization failure. Growth regulators such as IAA, NAA, 2,4-D, and GA3 can accelerate pollen tube growth or prolong pistil viability, improving the chances of successful fertilization.
- Large Number of Crosses
- Increasing Success Rate: Since the success rate of seed set in wide crosses is generally low, making a large number of crosses is often necessary to obtain viable seeds.
- Protoplast Fusion
- Cell Fusion Technique: When sexual crosses are not feasible, protoplast fusion can be employed. This technique allows for the fusion of plant cells from different species to produce hybrids.
- Embryo Culture
- Culturing Hybrid Embryos: Embryo culture is used to support the development of interspecific or intergeneric hybrids when hybrid zygotes fail to develop naturally. This method has been successfully applied to crops such as Triticum, Hordeum, Phaseolus, Nicotiana, Gossypium, Lycopersicon, Trifolium, and Cucumis.
- Grafting
- Hybrid Support: Grafting interspecific hybrids onto cultivated species can aid in their development and success. This technique helps in overcoming initial growth challenges and supports the hybrid’s establishment.
Sterility in Distant Hybrids
Sterility in distant hybrids, which are crosses between species that are not closely related, can vary significantly. This variability ranges from complete fertility to absolute sterility. Understanding the causes of sterility and the methods to address it is crucial for successful hybridization.
- Types of Sterility
- Partial Fertility: Some distant hybrids exhibit partial fertility, allowing them to be maintained through self-pollination, intercrossing, or backcrossing to the parent species. For example, hybrids between Lycopersicon esculentum (tomato) and Lycopersicon pimpinellifolium demonstrate almost complete fertility.
- Complete Sterility: Other hybrids are completely sterile and cannot produce viable seeds. An example includes the hybrid between sugarcane and maize, which requires clonal propagation for maintenance.
- Causes of Sterility
- Cytogenetic Factors
- Chromosome Pairing: In many interspecific hybrids, chromosomes may fail to pair properly during meiosis. Extreme cases may result in all chromosomes appearing as univalents, leading to unbalanced gametes and reduced fertility. This phenomenon is often associated with irregular chromosome distribution, such as rings, chains, bridges, fragments, and loops observed during various stages of meiosis.
- Structural Chromosome Changes: Stebbins identified that minor structural changes in chromosomes, termed cryptic structural changes, can lead to sterility. These changes may not affect chromosome pairing significantly or be detectable at metaphase I but still result in reduced fertility.
- Chromosome Doubling: One method to improve fertility in these hybrids is chromosome doubling, which creates amphidiploids. These doubled chromosome hybrids often show improved fertility due to more regular chromosome pairing.
- Genetic Factors
- Gene-Based Sterility: In some cases, sterility in hybrids with regular chromosome pairing may be attributed to specific genes rather than structural chromosome changes. For example, the F1 hybrid between Setaria italica (foxtail millet) and Setaria viridis showed normal chromosome pairing but exhibited significant pollen and ovule sterility. Similarly, hybrids between Oryza sativa (rice) varieties exhibit variable sterility due to complementary gene interactions.
- Cryptic Structural Changes: Sterility attributed to genes might actually be caused by small structural chromosome changes. These cryptic structural changes, which involve heterozygosity for small chromosomal rearrangements, duplications, or deficiencies, can contribute to sterility.
- Cytoplasmic Factors
- Cytoplasmic Influence: In some hybrids, sterility is influenced by the cytoplasm. This is evident when reciprocal crosses between species yield different fertility outcomes based on which parent species was used as the female. For example, certain races of Culex pipiens mosquitoes exhibit reciprocal differences in fertility, with one race providing fertile hybrids while the other results in sterility when used as the female parent. Similar cytoplasmic effects on sterility are observed in plants, such as Epilobium and Oenothera.
- Cytogenetic Factors
- Strategies to Mitigate Sterility
- Chromosome Manipulation: Techniques such as chromosome doubling can help address sterility caused by cytogenetic factors. By creating amphidiploids, the fertility of hybrids can be improved.
- Selecting Compatible Strains: Using different strains of the parental species may help overcome sterility issues, especially when genetic factors are involved.
- Embryo Culture: For hybrids that suffer from endosperm abortion, embryo culture techniques can facilitate the recovery of viable embryos and improve the chances of hybrid success.
Consequences of Segregation in Distant Hybrids
Distant hybrids, resulting from crosses between species within the same genus or between different genera, present complex outcomes in subsequent generations due to extensive genetic variation and segregation. The following points detail the consequences of segregation in these hybrids:
- Wide Range of Phenotypes
- High Genetic Heterozygosity: Distant hybrids are typically heterozygous for a large number of genes due to the genetic differences between the parent species. This high level of heterozygosity results in a broad spectrum of phenotypes in the F1 generation and subsequent generations.
- New Characteristics: Segregation of these genes produces many phenotypes that are distinct from those of the parent species, leading to the emergence of new characteristics not previously seen in the parental lines.
- Inferior Recombined Character Combinations
- Adaptive Significance: The parental species have evolved to possess character combinations well-suited to their environments. When these characters are recombined at random, many of the resulting new combinations are less adapted and weaker compared to the parental combinations.
- Reduced Fitness: As a result, most segregants in distant hybrids exhibit traits that are less effective or inferior in terms of adaptation and survival compared to the parental species.
- Limited Range of Recombination
- Extent of Variability: While the recombination in distant hybrids results in a wide range of phenotypes, the actual range of recombinants recovered is only a fraction of the total possible variability.
- Restricted Variability: Factors such as gametic elimination, zygotic elimination, and linkage contribute to a restricted range of recombinants. This limitation occurs because not all possible recombinants are viable or observed.
- Chromosomal and Genetic Challenges
- Reduced Chromosome Pairing: Interspecific hybrids often exhibit reduced chromosome pairing due to chromosome differentiation and lack of homology. This can lead to irregular chromosome distribution and the formation of unbalanced gametes.
- Impaired Recombination: Even when pairing occurs, crossing over or recombination between linked genes is significantly reduced, leading to deviations from expected Mendelian ratios in F2 generations. This impairment results from irregular meiosis, reduced recombination, and high rates of gametic and zygotic loss.
- Male Gamete Sensitivity
- High Male Sterility: Male gametes in distant hybrids are often more sensitive to chromosomal, genetic, or environmental factors compared to female gametes. Consequently, many distant hybrids exhibit high male sterility.
- Backcrossing: To overcome this issue, backcrossing with one of the parental species, typically used as the male parent, is common. However, even when F1 hybrids show partial fertility, the resulting F2 generation may resemble the backcross progeny more than a true F2 due to the survival of male gametes that are closer to one of the parental chromosome complements.
- Survival of Male Gametes
- Parental-like Gametes: In extreme cases, surviving male gametes may be almost identical to those of one of the parent species. For instance, pollen from the F1 hybrid of Triticum aestivum and T. timopheevii may closely resemble that of T. timopheevii.
- Varied F2 Range: Despite this, many surviving male gametes are close to, but not identical with, the parental chromosome complements. This results in the F2 generation showing a wider range of traits compared to the backcross progeny.
Limitations of Distant Hybridization in Crop Improvement
The following points outline the primary challenges associated with distant hybridization:
- Incompatible Crosses
- Challenges: Many distant crosses are feasible with advanced techniques; however, some crosses remain incompatible despite numerous efforts. Identifying the precise cause of such failures is often complex and time-consuming.
- Limitations: When the reason for incompatibility is unknown, overcoming these barriers becomes a matter of trial and error. While interspecific crosses (within the same genus) often yield useful hybrids, intergeneric (between genera) and interfamily (between families) crosses present significant difficulties. Techniques such as somatic hybridization are under development to address these incompatibilities, but standardization and practical application of these methods remain incomplete.
- Sterility in F1 Hybrids
- Challenges: F1 hybrids from distant crosses frequently exhibit varying degrees of sterility. Complete fertility in these hybrids is rare, as seen in crosses like Cicer arietinum × C. reticulatum.
- Limitations: Partial sterility can often be managed through self-pollination or backcrossing, but fully sterile F1 hybrids present significant challenges. Maintaining sterile hybrids requires additional resources and may necessitate chromosome doubling, which is not always feasible.
- Difficulties in Creating New Species
- Challenges: Forming new crop species through allopolyploidy following distant hybridization can result in several issues, including reduced economic yields and agronomic sterility.
- Limitations: Although traits can be improved through breeding and selection—as evidenced by triticale—such advancements require considerable time, effort, and resources.
- Lack of Chromosomal Homology
- Challenges: The transfer of specific genes from related species is often hindered by limited chromosomal pairing and recombination between the alien and recipient species.
- Limitations: While chromosome manipulation techniques may improve recombination in polyploid species, they still require extensive cytogenetic analysis. Most gene transfer relies on spontaneous pairing, which is less controlled and often less efficient.
- Undesirable Gene Linkages
- Challenges: Transferring genes may inadvertently include undesirable linked traits, which can undermine the commercial viability of the hybrid.
- Limitations: Breaking these undesirable linkages often requires extensive breeding efforts, consuming significant time and resources.
- Recessive Traits and Quantitative Characters
- Challenges: Transferring recessive traits or quantitative characters through distant hybridization is typically less successful compared to the transfer of dominant monogenic traits.
- Limitations: Dominant traits are more readily transferred and represent the majority of interspecific gene transfers.
- Flowering Issues in F1 Hybrids
- Challenges: Some interspecific hybrids, particularly those from certain genera, fail to produce flowers.
- Limitations: Techniques to induce flowering in these hybrids have often been unsuccessful. For instance, hybrids between species within the Rhizomatosae and section Arachis of peanuts, and certain Glycine hybrids, face challenges in flowering despite grafting techniques.
- Selection of Parent Varieties
- Challenges: Selecting the optimal parent variety is crucial. Transfers from related species to agronomically poor varieties necessitate further breeding.
- Limitations: High-performing modern varieties may not cross easily with wild relatives, which often prefer crossing with older land races rather than improved varieties.
- Seed Dormancy
- Challenges: Some F1 seeds from interspecific hybrids exhibit significant dormancy, remaining viable but ungerminated for extended periods.
- Limitations: Persistent seed dormancy can hinder the practical application of some hybrids, making them less usable in crop improvement efforts.
Applications of Distant Hybridization in Crop Improvement
The key applications are outlined as follows:
- Alien Addition Lines
- Concept: Alien addition lines involve the integration of an additional chromosome pair from a different species into the somatic chromosome complement of a crop species.
- Applications:
- Disease Resistance: For instance, wheat, oats, and tobacco have benefited from the introduction of chromosomes carrying disease resistance genes from related species. This approach has been instrumental in developing varieties with enhanced resistance to various pathogens.
- Alien Substitution Lines
- Concept: Alien substitution lines feature one chromosome pair from a different species replacing a chromosome pair of the recipient species.
- Applications:
- Trait Improvement: By substituting specific chromosomes, breeders can introduce novel traits from related species into a crop. This technique is particularly useful for incorporating traits like disease resistance or stress tolerance that are not present in the recipient species.
- Introgression of Genes
- Concept: This technique involves transferring small chromosome segments, which contain desirable genes, from one species to another.
- Applications:
- Disease Resistance: In cotton, for example, genes for resistance to black arm disease have been introgressed from Gossypium arboreum to Gossypium barbadense.
- Wider Adaptation: Cold tolerance genes have been transferred from wild relatives to various crops such as wheat, onion, potato, tomato, and grape, enhancing their ability to withstand lower temperatures.
- Quality Improvement: In oil palm, genes from wild relatives have been used to improve oil quality, demonstrating how introgression can enhance commercial traits.
- Changing Reproduction Modes: Introgression can also be used to alter reproductive strategies, which can be beneficial for crop breeding programs.
- Yield Enhancement: The introgression of genes related to yield can lead to significant improvements in crop productivity.
- Development of New Crop Species
- Concept: Wide hybridization, combined with chromosome doubling, can create entirely new crop species with novel genetic combinations.
- Applications:
- Example: Triticale, a hybrid between wheat and rye, illustrates how combining the genetic material from different species can result in a new crop with enhanced properties, such as better adaptability and improved yield.
Achievements of Distant Hybridization in Crop Improvement
The following points summarize the significant achievements realized through distant hybridization:
- Transfer of Disease Resistance
- Example: The transfer of disease resistance genes has been particularly successful in wheat. Rust resistance genes have been successfully introduced from related wild species into cultivated wheat varieties.
- Case Study: The development of Parbhani Kranti bhindi demonstrates the potential of distant hybridization. This variety, derived from a cross between Abelmoschus esculentus cv. Pusa Sawani and Abelmoschus manihot, exhibits complete resistance to yellow vein mosaic virus. It yields 110-120 quintals per hectare in the Kharif season and 85-90 quintals per hectare in summer, surpassing the yields of its parent variety. The fruit quality is also improved, contributing to its growing popularity.
- Cytoplasmic Male Sterility
- Applications: Cytoplasmic male sterility (CMS) has been achieved in crops such as wheat (Triticum aestivum), tobacco (Nicotiana tabacum), and cotton (Gossypium hirsutum) through the transfer of cytoplasm from related species.
- Limitations: Despite its potential, CMS systems have faced challenges in commercial applications, including issues with hybrid seed production and undesired side effects, as noted in tobacco.
- Development of New Crop Varieties
- Special Case: Sugarcane has benefited extensively from distant hybridization, which has led to the development of improved varieties. This success is partly due to the crop’s polyploid nature, which accommodates genetic variation more readily than diploid species.
- Limitations: The application of distant hybridization in diploid crops has been less successful, with fewer examples of significant improvements.
- Creation of New Crop Species
- Chromosome Doubling: Combining distant hybridization with chromosome doubling has been used to create new crop species. For instance, Triticum × Aegilops, Raphanobrassica, and Festuca × Lolium hybrids illustrate how distant hybridization, when paired with chromosome manipulation, can produce viable and productive new crop varieties.
- Example: Triticale, a hybrid between wheat and rye, showcases the benefits of this approach. While initially inferior, extensive breeding has significantly enhanced its quality and utility.
- Other Notable Hybrids
- Diverse Applications: Distant hybridization has been employed to develop hybrids between various plant genera, such as Triticum–Agropyron and Triticum–Aegilops. These hybrids have provided new opportunities for enhancing crop traits and developing new varieties.
- Liu, D., Zhang, H., Zhang, L., Yuan, Z., Hao, M., Zheng, Y. (2014). Distant Hybridization: A Tool for Interspecific Manipulation of Chromosomes. In: Pratap, A., Kumar, J. (eds) Alien Gene Transfer in Crop Plants, Volume 1. Springer, New York, NY. https://doi.org/10.1007/978-1-4614-8585-8_2
- Kaneko, Yukio & Bang, S.. (2014). Interspecific and intergeneric hybridization and chromosomal engineering of Brassicaceae crops. Breeding science. 64. 14-22. 10.1270/jsbbs.64.14.
- https://www.slideshare.net/slideshow/wide-hybridization-241046175/241046175
- https://www.jetir.org/papers/JETIRDR06028.pdf
- http://courseware.cutm.ac.in/wp-content/uploads/2020/05/barriers-in-widecrosshybridization.pdf
- https://www.rpcau.ac.in/wp-content/uploads/2020/03/Breeding-Methods.pdf
- http://courseware.cutm.ac.in/wp-content/uploads/2020/05/Download-Notes-25.pdf
- https://www.ijpab.com/form/2017%20Volume%205,%20issue%206/IJPAB-2017-5-6-1312-1315.pdf
- https://www.slideshare.net/slideshow/distant-hybridization/56359945
- https://www.slideshare.net/slideshow/distant-hybridisation-by-anakha-mohan-plant-breeding/80130463