What is Crossing over?
- Crossing over is a fundamental biological process occurring during meiosis, specifically at the pachytene stage of prophase I. This mechanism involves the exchange of genetic material between non-sister chromatids of homologous chromosomes, leading to genetic recombination. The term “crossing over” was introduced by Thomas Hunt Morgan in 1912, marking a significant advancement in understanding genetic variation.
- During the pachytene stage, homologous chromosomes pair closely, aligning so that corresponding segments of their chromatids are in proximity. This alignment facilitates the exchange of genetic material between non-sister chromatids within the synaptonemal complex—a protein structure that stabilizes the chromosome pairing. The process involves the breaking and rejoining of chromosomal segments, resulting in new combinations of genes.
- Crossing over is primarily observed in germinal cells during gametogenesis, a process known as meiotic or germinal crossing over. It contributes to genetic diversity by creating novel combinations of alleles, which are crucial for evolution and adaptation. Although crossing over is a universal phenomenon, it is less common in somatic cells during mitosis, referred to as somatic or mitotic crossing over.
- The underlying theories explaining the origins of crossing over include its role in DNA repair and its evolutionary development from bacterial transformation. One theory posits that crossing over serves as a mechanism to replace damaged DNA regions. Another suggests that meiosis evolved from bacterial mechanisms to enhance genetic diversity.
- During crossing over, chromatids from the same chromosome, known as sister chromatids, separate, while non-sister chromatids from homologous chromosomes exchange segments. This exchange creates recombinant chromatids, which are crucial for generating genetic variation. However, external factors such as heat shock, chemicals, and radiation can influence the frequency and efficiency of crossing over. Notably, certain organisms, like male Drosophila and female Bombyx mori (silk moth), do not exhibit crossing over.
Definition of Crossing over
Crossing over is the exchange of genetic material between non-sister chromatids of homologous chromosomes during prophase I of meiosis, resulting in new combinations of genes.
Characteristics of Crossing over
- Two Levels of Occurrence:
- Chromosomal Level: This is known as chromosomal crossing over, which involves the physical exchange of chromosomal segments.
- DNA Level: Referred to as genetic recombination, this involves the exchange of genetic material at the molecular level between DNA molecules.
- Reciprocal Exchange:
- Crossing over entails a reciprocal exchange of genetic material between homologous chromosomes in heterozygotes. This exchange ensures that each chromosome in the homologous pair acquires segments from its partner, contributing to genetic diversity.
- Mechanism of Exchange:
- The process involves the break-and-exchange of genetic material between non-sister chromatids following DNA replication. This occurs within the synaptonemal complex during prophase I of meiosis, leading to the formation of recombinant chromatids.
- Frequency and Mapping:
- The frequency of crossing over is influenced by the physical distance between genes on a chromosome. A higher frequency of crossing over typically occurs between genes that are farther apart. This property is utilized in constructing genetic maps, helping to determine the relative positions of genes on chromosomes.
At which stage Crossing over occurs?
- Zygotene Stage:
- Crossing over begins during the zygotene stage of prophase I in meiosis. During this phase, homologous chromosomes pair closely in a process known as synapsis. This alignment allows the chromosomes to come into proximity, facilitating the exchange of genetic material.
- Pachytene Stage:
- The primary events of crossing over occur during the pachytene stage. At this point, the paired homologous chromosomes, now called bivalents, align side by side. The mutual attraction between allelic genes on these chromosomes ensures that similar segments are closely juxtaposed, setting the stage for genetic exchange.
- Diplotene Stage:
- By the diplotene stage, each chromosome has split longitudinally into two chromatids. The bivalent, which initially consisted of two chromosomes, now consists of four chromatids—two from each homologous chromosome. This four-strand arrangement, also known as the tetrad, is where crossing over can be observed. The process involves the exchange of segments between the maternal and paternal chromatids.
- Detection of Crossing Over:
- The crossing over event is detectable at the tetrad stage. The exchange of genetic material primarily occurs between the non-sister chromatids of the homologous chromosomes, contributing to genetic recombination and diversity.
Types of Crossing over
Crossing over can be categorized based on its occurrence in different types of cells and the number of chiasmata formed during the process. The two primary classifications are based on the cell type where crossing over occurs, and the secondary classification is based on the number of chiasmata.
- Based on Cell Type:
- Somatic or Mitotic Crossing Over:
- This type of crossing over occurs in the chromosomes of somatic or body cells during mitotic cell division. It is relatively rare and does not have significant genetic implications compared to meiotic crossing over. Reports of somatic crossing over have been documented in species such as Drosophila and the fungus Aspergillus nidulans. Despite its rarity, it provides insights into genetic processes and chromosome behavior.
- Germinal or Meiotic Crossing Over:
- This type occurs in germinal cells during meiosis, specifically during the formation of gametes. Meiotic crossing over is universal and plays a crucial role in genetic diversity. It involves the exchange of genetic material between homologous chromosomes and is essential for proper chromosome segregation and the generation of genetic variation.
- Somatic or Mitotic Crossing Over:
- Based on Number of Chiasmata:
- Single Cross Over:
- Involves the formation of a single chiasma, where genetic material is exchanged between two chromatids out of the four present in a tetrad. This type of crossing over results in relatively straightforward genetic recombination.
- Double Cross Over:
- Characterized by the formation of two chiasmata, which can involve two, three, or all four chromatids. This type of crossing over results in a more complex recombination of genetic material, leading to a higher variability in the resultant chromatids.
- Multiple Cross Over:
- Involves the formation of more than two chiasmata, although this is relatively rare. Multiple cross overs can involve extensive genetic recombination but occur less frequently compared to single and double cross overs.
- Single Cross Over:
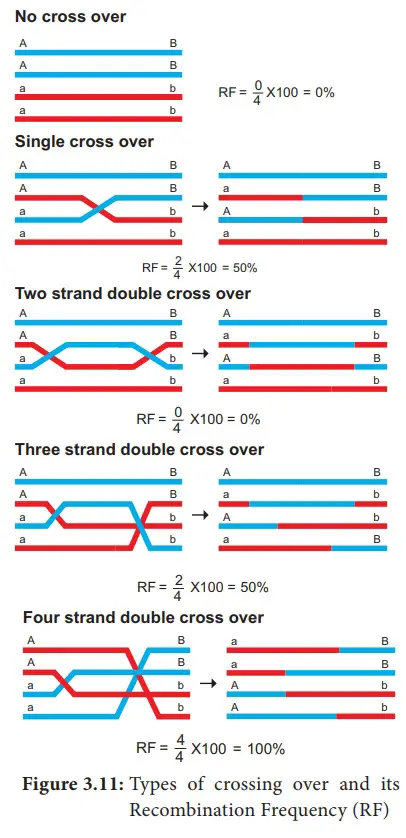
Theories of Chromosomal Crossing Over
Several theories have been proposed to explain this complex process:
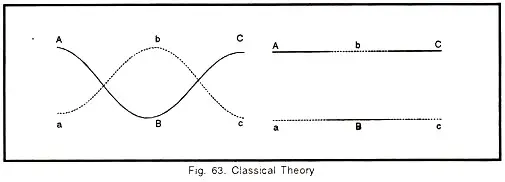
- Classical Theory:
- Proposed by: Morgan and Sharp (1934)
- Core Idea: Also known as the two-plane theory, this model suggests that crossing over occurs when chromatids from homologous chromosomes come into contact. According to this theory, chiasma formation—where non-sister chromatids physically cross each other—occurs when chromatids from different chromosomes interact.
- Process:
- During synapsis, sister chromatids of homologous chromosomes remain attached.
- As homologous chromosomes begin to separate during diplotene, physical strain at the chiasma site may cause chromatid breakage.
- The broken chromatids then rejoin, leading to genetic recombination.
- Key Points:
- Chiasma formation is considered the result of crossing over.
- Each chiasma is thought to be associated with a recombination event.
- Limitations: This theory has been largely rejected due to insufficient experimental support. It is historically significant but does not fully account for all observed phenomena in crossing over.
- Chiasma Type Theory (Breakage and Reunion Theory):
- Proposed by: Janssens (1909), expanded by Belling and Darlington
- Core Idea: This theory, also known as one-plane theory, posits that crossing over is initiated by the breakage of non-sister chromatids, followed by their reunion. Chiasmata form at the points where crossing over occurs.
- Process:
- During diplotene, homologous chromosomes move apart, and chiasmata are formed at crossover points.
- The formation of chiasmata is a direct consequence of crossing over.
- Key Points:
- Chiasma formation is the result of crossing over, not the cause.
- Each chiasma corresponds to a recombination event.
- A 1:1 ratio is expected between the number of chiasmata and the frequency of crossing over.
- Support: This theory is widely accepted and supported by evidence linking chiasmata and crossing over. However, some exceptions, such as the presence of chiasmata without crossing over in certain cases, challenge its universality.
- Copy-Choice Theory:
- Proposed by: J. Lederberg (1955)
- Core Idea: This hypothesis suggests that crossing over involves simultaneous duplication and recombination. According to this theory, chromatids duplicate by synthesizing new genes, which then recombine to form new genetic arrangements.
- Process:
- Chromatids duplicate, producing new strands.
- Recombination occurs between these newly synthesized chromatids.
- Key Points:
- Only two of the four chromatids in a tetrad are involved in crossing over, leaving two original strands intact.
- Duplication and recombination occur simultaneously, but evidence suggests that DNA replication precedes synapsis.
- Limitations: The theory faces objections due to its discrepancy with observed timing of DNA replication and involvement of only two chromatids in crossing over.
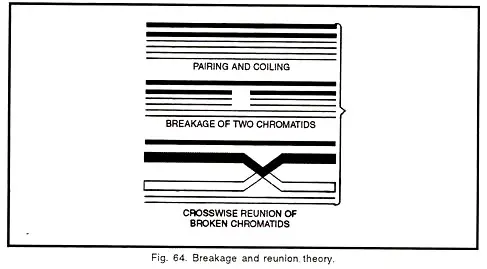
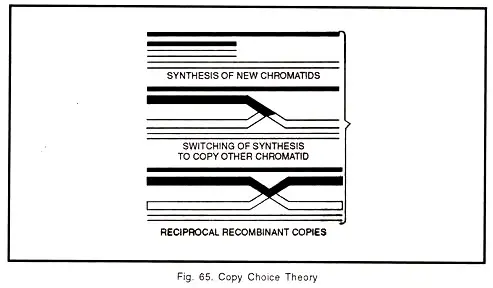
Other Theories for Mechanism of Crossing Over
Understanding the mechanisms behind crossing over is crucial in genetics, and several theories have been proposed to explain how this process occurs. Here are the key theories:
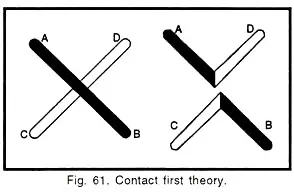
- The ‘Contact First’ Theory:
- Proposed by: Serebrovsky
- Core Idea: According to this theory, the initial step in crossing over involves two non-sister chromatids coming into contact with each other. This contact happens at specific points along the chromatids. Subsequently, these chromatids break at the contact points. The broken segments then reunite, leading to the formation of new genetic combinations. This theory emphasizes the importance of the initial contact between chromatids in initiating crossing over.
- The ‘Breakage First’ Theory:
- Proposed by: Muller
- Core Idea: This theory suggests a different sequence of events compared to the Contact First Theory. It posits that crossing over begins with the breakage of two non-sister chromatids without any prior crossing. The broken segments then rejoin, resulting in the formation of new arrangements of genetic material. This theory is widely accepted and underscores the role of chromatid breakage in initiating crossing over.
- Strain Theory (Precosity Theory):
- Proposed by: Darlington
- Core Idea: Darlington’s theory proposes that crossing over results from strain or tension during the pairing of homologous chromosomes. When chromosomes pair and twist around each other, they experience tension, leading to breakage at the points of contact. The broken ends of chromatids then rejoin, potentially with segments from different chromatids, facilitating genetic recombination. Darlington emphasized the role of physical strain in breaking chromatids and suggested that chiasmata, the points where chromatids cross, are crucial for holding the broken ends together. He also introduced concepts like exchange pairing and distributive pairing to explain different pairing scenarios.
- Belling’s Hypothesis:
- Proposed by: Belling (1933)
- Core Idea: Belling’s hypothesis presents an alternative view, suggesting that crossing over results from the exchange of newly duplicated chromatids. According to this theory, crossing over is not caused by the breakage and reunion of existing chromosomes but by interactions between newly formed chromatids. Belling proposed that new chromomeres form alongside their sister chromatids, and interconnecting fibers may form between non-sister chromomeres, leading to crossing over. This hypothesis highlights the role of newly duplicated genetic material in the crossing-over process.
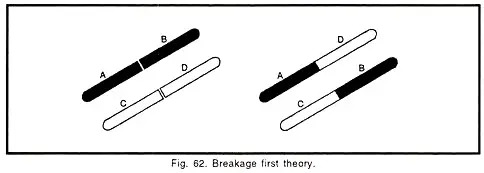
Mechanism of Crossing over
The mechanism of crossing over is a multi-step process occurring during meiosis that facilitates genetic recombination. This process can be detailed as follows:
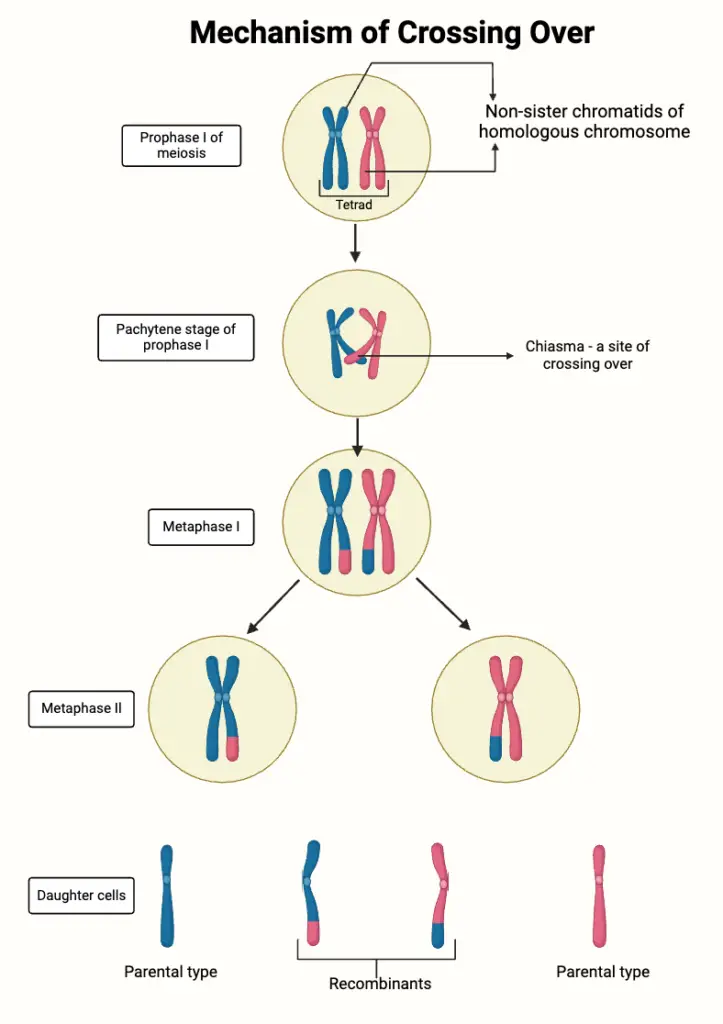
- Synapsis:
- Initiation: Synapsis begins during the zygotene stage of prophase I in meiosis. Homologous chromosomes, each consisting of two chromatids, align closely with each other. This alignment can initiate from the chromosome ends or internal regions and progresses in a zipper-like manner.
- Formation of Bivalents: During synapsis, homologous chromosomes are paired to form a structure known as a bivalent, where each chromosome is in close contact with its homologous partner. This pairing is driven by mutual attraction between allelic genes.
- Types of Synapsis:
- Procentric Synapsis: Pairing starts from the middle of the chromosomes.
- Proterminal Synapsis: Pairing begins at the telomeres.
- Random Synapsis: Pairing can start at any point along the chromosome.
- Duplication of Chromosomes:
- Stage: Occurs during the pachytene stage of prophase I.
- Process: Each chromosome in the bivalent undergoes longitudinal splitting to form two sister chromatids per chromosome. Thus, the bivalent now consists of four chromatids, forming a tetrad. This duplication is a result of previously replicated DNA and chromosomal proteins.
- Crossing Over:
- Occurrence: Crossing over takes place during the pachytene stage when the chromosomes are in the tetrad formation.
- Mechanism: The process involves the exchange of chromosomal segments between non-sister chromatids of homologous chromosomes. Initially, recombination nodules become visible at points where chromatids will undergo exchange. Endonucleases induce breaks in the chromatids at corresponding points. These broken segments are then exchanged and joined by ligase, resulting in new combinations of genetic material. This exchange forms structures known as chiasmata.
- Chiasma Formation: Chiasmata are cross-shaped structures where chromatids exchange segments. The frequency and number of chiasmata depend on the length of the chromosomes and the distance between genes.
- Terminalization:
- Process: After crossing over, the chiasmata move towards the ends of the chromatids in a process called terminalization.
- Stages:
- Diplotene: During this phase, desynapsis begins, leading to the dissolution of the synaptonemal complex. Homologous chromosomes start to pull apart.
- Diakinesis: Chromosomes detach from the nuclear envelope, and the bivalents, now clearly visible with four separate chromatids, exhibit terminalization. The chiasmata move towards the ends of the chromatids, leading to the complete separation of homologous chromosomes.
Crossing Over Percentage
The percentage of crossing over is a crucial metric in genetics, providing insights into the relative positions of genes on a chromosome. It reflects how often genetic recombination occurs between two genes and is instrumental in constructing chromosome maps. Here’s an overview of how the percentage of crossing over varies and its significance:
- Variation with Gene Distance:
- Principle: The frequency of crossing over is influenced by the distance between genes on a chromosome. Specifically, the likelihood of crossing over increases with greater distances between the genes.
- Example: If two genes, A and B, are located close to each other, the percentage of crossing over between them is relatively low. Conversely, if genes A and C are positioned farther apart, the percentage of crossing over between them is higher.
- Explanation: The physical distance between genes affects the probability of recombination. Longer distances provide more opportunities for chromatids to exchange segments, thereby increasing the percentage of crossing over.
- Correlation with Chromosome Maps:
- Importance: The percentage of crossing over is directly proportional to the distance between genes, making it a valuable tool for constructing genetic maps.
- Application: By measuring the percentage of crossing over, researchers can estimate the linear distance between genes on a chromosome. This information helps in mapping genes and understanding their relative positions.
- Morgan as a Unit of Measurement:
- Definition: The unit used to quantify the percentage of crossing over is termed a “morgan,” named after the geneticist Thomas Hunt Morgan.
- Conversion: A crossing over percentage of 15% between two genes is equivalent to 15 “morgans” on the chromosome map. This unit facilitates the representation of gene distances and recombination frequencies.
- Implications for Genetic Analysis:
- Genetic Mapping: Accurate measurements of crossing over percentages are essential for constructing detailed chromosome maps. These maps provide a framework for studying gene interactions, genetic disorders, and evolutionary relationships.
- Recombination Frequency: The recombination frequency, derived from crossing over percentages, is used to determine the genetic distance between genes. This helps in identifying gene linkage and understanding the genetic architecture of organisms.
Factors Affecting Crossing Over
The following points outline the primary factors that affect crossing over:
- Sex:
- Drosophila: In male Drosophila melanogaster, crossing over is often suppressed or significantly reduced. This pattern contrasts with female Drosophila, where crossing over is more frequent.
- Mammals: Similarly, in male mammals, crossing over frequencies are generally lower compared to females.
- Silk Moth: In the silk moth (Bombyx mori), crossing over is absent in females.
- General Observation: Typically, the heterogametic sex (e.g., males in many species) exhibits lower crossing over frequencies compared to the homogametic sex.
- Mutation:
- Effect on Crossing Over: Genetic mutations can impact crossing over rates. For example, Gowen identified a mutation in Drosophila that reduces crossing over across all chromosomes.
- Age:
- Impact on Frequency: The age of an individual can influence crossing over rates. Research by Bridges indicated that in Drosophila females, crossing over tends to decrease as the organism ages.
- Inversion:
- Chromosomal Inversions: Inversions involve rearrangements within a chromosome segment. These inversions generally reduce crossing over within the inverted segment. However, crossing over frequencies may increase in chromosome regions outside the inversion. The exact mechanisms behind these changes remain under investigation.
- X-Radiation:
- Influence of Radiation: Exposure to X-rays and other forms of radiation, such as radium, has been shown to increase crossing over frequencies. Studies by Hanson and Muller demonstrated that irradiation can induce higher crossing over rates.
- Temperature:
- Temperature Effects: Both low and high temperatures have been observed to increase crossing over frequencies. For instance, in female Drosophila, temperature variations can influence the rate of genetic recombination.
- Centromere Vicinity:
- Chromosomal Regions: Crossing over is notably frequent near centromeres and at the ends of chromosomes. Additionally, certain factors influence somatic or mitotic crossing over, although these factors are not yet fully understood.
- Somatic Crossing Over: Somatic crossing over, observed by Stern in Drosophila, occurs in somatic cells and results in a patch of crossover tissue. This type of crossing over does not affect gametes and has no direct genetic importance.
Cytological Evidence of Crossing Over
The concept of crossing over—where genetic material is exchanged between homologous chromosomes—was once a theoretical construct in genetics. However, through the pioneering work of cytologists, this hypothesis gained substantial empirical support. Here, we explore the cytological evidence that validates crossing over, highlighting key experiments and their contributions to our understanding.
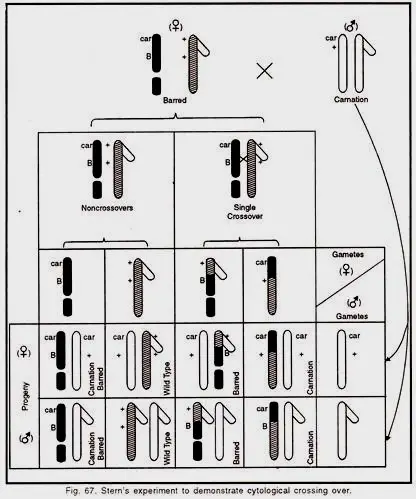
- Challenges in Observing Crossing Over:
- Similar Appearance of Homologous Chromosomes: Homologous chromosomes are indistinguishable under a microscope due to their identical appearance, making it difficult to observe crossing over directly.
- Intimate Coiling of Chromatids: During crossing over, chromatids are tightly coiled around one another, further complicating direct observation.
- Invisibility in Living Cells: Crossing over cannot be observed in living cells, and fixed and stained preparations do not provide clear evidence of chromatid exchanges.
- Stern’s Experiments on Drosophila:
- Experimental Design: Stern utilized a variety of Drosophila melanogaster with a broken X chromosome attached to a segment of the Y chromosome. This setup allowed for differentiation between crossover and non-crossover progeny.
- Genetic Markers: The experimental flies carried mutant genes for eye color and shape: carnation (car) for darkish red eyes and bar (B) for narrow eyes. In females (XX), one X chromosome had these mutations, while the other X contained normal alleles. The Y chromosome segment was used as a marker.
- Results: When these females were crossed with males, progeny exhibited four types of gametes: two with crossover and two without. This setup allowed Stern to distinguish between crossover and non-crossover events, thereby providing cytological evidence of crossing over.
- Creighton and McClintock’s Experiments on Maize:
- Experimental Design: Creighton and McClintock studied maize with a pair of chromosomes where one had a translocated piece from another chromosome and a distinctive knob at one end.
- Genetic Markers: The normal chromosome carried genes for colorless endosperm (c) and starchy endosperm (W). The knobbed chromosome had alleles for colored endosperm (C) and waxy endosperm (w). They crossed these plants with others possessing recessive genes for colorless and waxy endosperm (ccww).
- Results: The progeny from these crosses showed four types of offspring: two with crossover and two without. Non-crossover plants had either normal or knobbed chromosomes, while crossover plants displayed combinations of these features, demonstrating chromosomal exchanges.
- Significance of Cytological Evidence:
- Validation of Crossing Over: These experiments provided concrete cytological evidence that supports the genetic hypothesis of crossing over. By visualizing chromosomal exchanges, scientists were able to confirm that crossing over involves the physical exchange of chromosomal segments.
- Implications for Genetic Mapping: The ability to observe crossing over cytologically has important implications for genetic mapping and understanding gene linkage. These experiments laid the groundwork for more advanced studies in genetics and cytogenetics.
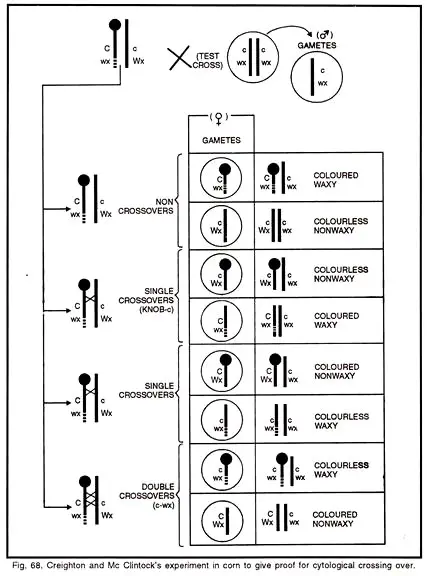
What is Non-Homologous Crossover?
Non-homologous crossover, also known as non-homologous recombination or non-homologous end joining, refers to a type of genetic recombination that occurs between DNA sequences that are not strictly homologous. This process can lead to several significant outcomes in terms of genetic variation and genome stability. Below is a detailed explanation of non-homologous crossover:
- Definition and Context
- Non-Homologous Crossover: Unlike traditional homologous recombination, which involves the exchange of genetic material between homologous chromosomes with similar sequences, non-homologous crossover occurs between regions of DNA that do not have a high degree of sequence similarity. This type of recombination can lead to the insertion, deletion, or rearrangement of genetic material.
- Mechanism
- Repetitive DNA Sequences: A significant portion of the genome consists of repetitive DNA sequences or satellite DNA. These repetitive segments are relatively uniform within a species and can sometimes align incorrectly due to their similar sequences.
- DNA Replication: During DNA replication, each strand serves as a template for creating new complementary strands. Typically, this process results in the production of two identical sister chromatids. However, when sequence alignments are imperfect, it can lead to non-homologous crossover events.
- Crossover Events: Non-homologous crossover occurs less frequently than homologous crossover but can still have a considerable impact on the genome. These events often involve unequal exchanges of genetic material, leading to insertions or deletions in the chromosome.
- Consequences
- Unequal Exchanges: The majority of non-homologous crossovers involve the unequal exchange of genetic material. This can result in significant genetic mutations, such as insertions or deletions, which affect multiple loci simultaneously.
- Genetic Mutations: Non-homologous crossover is a major source of mutations in the genome. These mutations can cause gene duplications, which might have implications for gene function and genome stability.
- Significance
- Impact on Genetic Variation: While less common than homologous recombination, non-homologous crossover events are crucial for generating genetic diversity. They can introduce variations that may affect gene expression and function.
- Implications for Genome Stability: The disruptions caused by non-homologous crossover can lead to genomic instability. This instability may contribute to various genetic disorders and diseases.
- Comparative Rarity
- Frequency and Effects: Although non-homologous crossover events are rarer compared to homologous recombination, their impact is profound. These events are associated with substantial alterations in the genetic material and can influence numerous loci within the genome.
Significance of Crossing over
Crossing over is a fundamental genetic process observed across a wide array of organisms, including bacteria, yeast, fungi, higher plants, and animals. Its significance can be outlined as follows:
- Genetic Variation:
- New Gene Combinations: Crossing over results in the exchange of genetic material between homologous chromosomes. This exchange creates new combinations of genes, which is crucial for generating genetic diversity within a population. Increased genetic variation provides the raw material for natural selection and evolution.
- Evolutionary Impact: By facilitating the creation of novel gene combinations, crossing over plays a pivotal role in the evolutionary process. It allows populations to adapt to changing environments and can lead to the development of new traits that may be beneficial for survival.
- Chromosomal Mapping:
- Linear Gene Arrangement: Studies of crossing over provide evidence that genes are arranged in a linear sequence along chromosomes. This linear organization is essential for understanding how genes are linked and how they interact.
- Genetic Maps: The frequency of crossing over between genetic loci is used to construct genetic maps. These maps illustrate the relative positions of genes on chromosomes and are instrumental in studying genetic linkage and inheritance patterns.
- Understanding Gene Function:
- Mechanism of Gene Action: The process of crossing over helps elucidate the nature and mechanisms of gene action. By observing how genes are exchanged and recombined, researchers can gain insights into gene function and regulation.
- Research Applications: Knowledge of crossing over enhances the ability to study genetic disorders and traits, as well as to investigate the effects of genetic modifications.
- Practical Applications:
- Plant Breeding: Crossing over can result in the formation of advantageous gene combinations that may be beneficial for agriculture. These new combinations can be utilized in plant breeding programs to develop crops with desirable traits, such as improved yield, resistance to diseases, or better nutritional quality.
- Universal Occurrence:
- Wide Distribution: Crossing over is a universal phenomenon observed in a diverse range of organisms, including viruses, bacteria, fungi, plants, and animals. This universality underscores its fundamental role in genetic processes across different life forms.
Example of Crossing Over
To illustrate the concept of crossing over, consider the well-known example involving the fruit fly Drosophila melanogaster. This example highlights how genetic recombination occurs during meiosis and its effects on phenotypic outcomes.
- Experimental Setup
- Parental Generation (P Generation): In this example, a mutant Drosophila with recessive traits for pink eyes (r) and curled wings (s) is crossed with a wild-type Drosophila displaying dominant traits for red eyes (R) and straight wings (S).
- F1 Generation: The resulting F1 progeny all exhibit the dominant phenotypes, displaying red eyes and straight wings with the genotype RrSs. This indicates that the dominant alleles mask the recessive traits in this generation.
- Test Cross
- Crossing F1 Female with Double Recessive Male: A female from the F1 generation (RrSs) is crossed with a double recessive male (rrss) from the parental generation. This type of cross is known as a test cross and is used to determine the linkage and recombination of alleles.
- Gamete Production: The F1 female will produce four types of gametes: RS, Rs, rS, and rs, based on the possible combinations of her alleles.
- F2 Generation Results
- Phenotypic Ratios: Upon mating the F1 female gametes with the single type of male gametes (rrss), the F2 generation displays a phenotypic ratio as follows:
- 49% flies with red eyes and straight wings
- 49% flies with pink eyes and curled wings
- 1% flies with red eyes and curled wings
- 1% flies with pink eyes and straight wings
- Phenotypic Ratios: Upon mating the F1 female gametes with the single type of male gametes (rrss), the F2 generation displays a phenotypic ratio as follows:
- Analysis of Results
- Non-Crossover Types: The majority (98%) of the F2 progeny exhibit the phenotypes corresponding to the original parental types (red eyes and straight wings; pink eyes and curled wings). This indicates that these types result from non-crossover events, where the alleles did not exchange between chromatids.
- Crossing Over Types: The remaining 2% of the progeny show new combinations of traits (red eyes with curled wings and pink eyes with straight wings). These novel combinations result from crossing over, where segments of chromatids from homologous chromosomes have exchanged genetic material.
- Conclusion
- Linkage and Recombination: The results from this experiment demonstrate that the genes for eye color and wing shape are located on the same chromosome. In the majority of gametes (98%), the genes are inherited together without crossing over. However, in the remaining 2% of gametes, crossing over occurs, leading to new genetic combinations. This recombination illustrates the physical exchange of chromatid segments between homologous chromosomes during meiosis, thus providing evidence for the linkage of genes and the occurrence of genetic recombination.
Fun facts on Crossing over
- Did you know that crossing over is a key mechanism during meiosis that leads to genetic diversity by exchanging segments between homologous chromosomes?
- Have you heard that crossing over typically occurs during prophase I of meiosis, specifically during the pachytene stage?
- Are you aware that crossing over involves the exchange of genetic material between non-sister chromatids of homologous chromosomes, resulting in new allele combinations?
- Did you know that the physical sites where crossing over occurs are called chiasmata, which are visible under a microscope during meiosis?
- Can you believe that crossing over is responsible for creating recombinant chromosomes, which contribute to genetic variation in offspring?
- Have you ever heard that the frequency of crossing over between two genes can be used to estimate their relative distances on a chromosome, helping to create genetic maps?
- Did you know that crossing over is a universal process observed in a wide range of organisms, from bacteria to humans?
- Are you aware that the number of chiasmata formed during meiosis can vary depending on the length of the chromosomes and the specific genetic context?
- Did you know that crossing over can occur at multiple points along a single chromosome, potentially leading to multiple chiasmata and increased genetic diversity?
- Have you heard that crossing over is essential for the proper segregation of chromosomes during meiosis, reducing the risk of aneuploidy?
- Can you believe that crossing over helps ensure that offspring inherit a mix of genetic material from both parents, contributing to evolutionary processes?
- Did you know that the process of crossing over involves breaking and rejoining chromatids, facilitated by specific enzymes like endonucleases and ligases?
- Are you aware that crossing over can lead to gene duplications and deletions if unequal exchanges occur between chromatids?
- Have you ever heard that the rate of crossing over can be influenced by genetic factors, environmental conditions, and even age?
- Did you know that crossing over plays a crucial role in generating genetic variation in populations, which is vital for natural selection and evolution?
- Can you believe that the study of crossing over has helped scientists understand the linear arrangement of genes on chromosomes and the formation of genetic linkage maps?
- Are you aware that some genetic disorders and abnormalities can result from improper crossing over, leading to chromosomal rearrangements?
- Did you know that crossing over is more frequent in certain regions of chromosomes known as recombination hotspots, where the exchange of genetic material is more likely to occur?
- Have you heard that crossing over can sometimes lead to gene conversions, where one allele is converted into another during the recombination process?
- Can you believe that crossing over is a fundamental process in genetics that not only increases genetic diversity but also provides valuable insights into the mechanisms of inheritance?
- James German, Cytological Evidence for Crossing-Over in vitro in Human Lymphoid Cells.Science144,298-301(1964).DOI:10.1126/science.144.3616.298
- http://courseware.cutm.ac.in/wp-content/uploads/2020/05/Cytological-basis-of-crossing-over-1.pdf
- https://www.rlsycollegebettiah.ac.in/wp-content/uploads/2023/02/file_63e39fa98b8a4.pdf
- https://dhingcollegeonline.co.in/attendence/classnotes/files/1605551586.pdf
- https://uomustansiriyah.edu.iq/media/lectures/6/6_2021_09_15!11_21_24_PM.pdf
- https://www.notesonzoology.com/genetics/linkage-and-crossing-over-of-genes-with-diagram-genetics/6511
- https://www.biologydiscussion.com/genetics/crossing-over-meaning-mechanism-and-significance/35505
- https://www.brainkart.com/article/Crossing-Over_38222/
- https://www.geeksforgeeks.org/crossing-over/
- https://biology.homeomagnet.com/crossing-over/
- https://studymind.co.uk/notes/how-meiosis-produces-variation/
- https://old-ib.bioninja.com.au/standard-level/topic-3-genetics/33-meiosis/crossing-over.html
- http://www.lscollege.ac.in/sites/default/files/e-content/CROSSING-%20OVER_1.pdf
- https://tacs.dypvp.edu.in/documents/Study-Material/Microbiology-TYBSc/Crossing-Over.pdf
- https://kvmwai.edu.in/upload/StudyMaterial/Crossing_over.pdf