A cell is the basic building block of all living things, acting like a tiny, self-contained factory that keeps life running. Think of it as the smallest unit capable of performing the essential functions needed for survival—like converting nutrients into energy, growing, and reproducing. Every organism, from plants and animals to humans, is made up of cells, which come in different shapes and sizes depending on their role. For example, nerve cells transmit signals in the body, while plant cells have rigid walls to support structure. Cells work together to form tissues, organs, and complex systems, making them the foundation of biology. Understanding cells helps scientists unlock mysteries about health, disease, and how life itself functions. Simple yet incredibly sophisticated, these microscopic powerhouses are key to everything from healing a scraped knee to exploring groundbreaking medical treatments.
What is a cell?
Usually tiny and limited by a plasma membrane controlling passage of molecules, a cell is the smallest structural and functional unit of living entities.
Along with other organelles serving specific purposes, a cell consists of cytoplasm and genetic material arranged inside a nucleus or nucleoid area.
With unicellular animals made of one single cell and multicellular organisms made of specialized cells cooperating, cells are the building blocks of life.
From simple biological study to uses in biotechnology and medicine, where knowledge of cellular processes is essential for developments in agriculture, genetics, and disease treatment, the value of cells spans all spheres.
Robert Hooke’s discovery of cell walls in cork and Antoni van Leeuwenhoek’s microscopic views of live cells in pond water started the history of cell discovery in the 17th century.
Matthias Schleiden and Theodor Schwann first formalized cell theory in the 19th century when they said all creatures are made of cells and Rudolf Virchow subsequently said all cells originate from pre-existing cells.
Broadly speaking, prokaryotic cells—which lack a real nucleus and are typified by bacteria and archaea—are one kind of cell; eukaryotic cells are another type with defined nucleus and sophisticated organelles.
Important eukaryotic organelles are the nucleus, the genetic control center; mitochondria for energy generation; endoplasmic reticulum for protein and lipid synthesis; Golgi apparatus for molecular modification and sorting; lysosomes for waste processing.
Thanks to developments in cellular research like synthetic biology and cryo-electron microscopy, researchers are able to construct minimum cells for uses in targeted medicines and renewable energy generation and study cellular structures at atomic resolution.
Emerging multidisciplinary discipline linking molecular biology to tissue-level functions and disease pathology is seeing cells as material with special mechanical and physical characteristics.
What’s found inside a cell
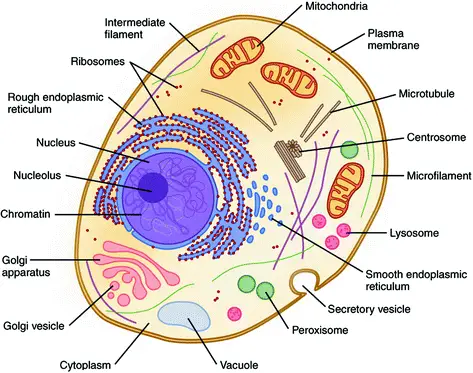
- Comprising a lipid bilayer, plasma membrane encloses the cell and controls waste products, ions, and nutrient flow.
- Comprising cytosol and organelles, cytoplasm is the gel-like matrix filling the cell and where most cellular activity occurs.
- The semifluid component of the cytoplasm surrounding organelles, cytosol helps to create molecular transit and reaction areas.
- Microtubules, microfilaments, and intermediate filaments make form the dynamic network of protein filaments known as cytoskeleton, which supports structural integrity, preserves cell shape, and facilitates intracellular movement and motility. .
- Found in eukaryotic cells, the membrane-bound organelle known as nucleus stores DNA and regulates gene expression and replication mechanisms.
- Within the nucleus, the dense area known as nucleolus hosts ribosomal RNA transcription and assembly of ribosome subunits.
- Double-membraned organelles in charge of generating ATP via oxidative phosphorylation serve both in metabolic control and apoptosis.
- Found free in the cytosol or connected to the rough endoplasmic reticulum, rRNA and proteins make up macromolecular machines called ribosomes that catalyze polypeptide synthesis.
- Rough ER, studded in ribosomes for protein synthesis and folding, and smooth ER engaged in lipid synthesis, detoxification, and calcium storage make up endoplasmic reticulum.
- A stack of membrane-bound cisternae, the Golgi apparatus sorts, bundles, and alters proteins and lipids for release or distribution to other organelles
- Membrane-bound vesicles with hydrolytic enzymes for macromolecules’ intracellular breakdown and cellular component recycling are lysosomes.
- Little, enzyme-filled organelles called peroxisomes accelerate oxidative events including neutralizing reactive oxygen species and fatty acid beta-oxidation.
- Common in plant cells, vacuoles are membrane-bound compartments that store waste products and nutrients, therefore supporting cell turgor and pH equilibrium.
- Found in photosynthetic eukaryotes, chloroplasts have chlorophyll and use photosynthesis to transform light energy into chemical energy kept in carbs.
- Present in plant cells, fungi, many prokaryotes, cell walls—a hard extracellular structure made of cellulose, chitin, or peptidoglycan—that offers mechanical support and protection
- Lacking a nuclear membrane, the circular DNA chromosome dwells and interacts with proteins in the nucleoid area of prokaryotic cells for transcription and replication.
- Found in animal cells close to the nucleus, centrioles are cylindrical organelles made of tubulin microtubule triplets that arrange spindle fibers during cell division.
- During mitosis and interphase, the major microtubule organizing core is centrosome, composed of two centrioles and surrounding pericentriolar material.
Organelle | Function | Factory part |
---|---|---|
Nucleus | DNA Storage | Room where the blueprints are kept |
Mitochondrion | Energy production | Powerplant |
Smooth Endoplasmic Reticulum (SER) | Lipid production; Detoxification | Accessory production – makes decorations for the toy, etc. |
Rough Endoplasmic Reticulum (RER) | Protein production; in particular for export out of the cell | Primary production line – makes the toys |
Golgi apparatus | Protein modification and export | Shipping department |
Peroxisome | Lipid Destruction; contains oxidative enzymes | Security and waste removal |
Lysosome | Protein destruction | Recycling and security |
What are Cell Organelles?
- Within a multicellular organism, cell organelles are specialized structures within cells that have different purposes similar to those of organs.
- Organelle literally means little organ reflecting their function as functional elements within the cell.
- Within eukaryotic cells, compartmentalization by organelles helps to separate metabolic activities therefore minimizing interference and improving efficiency.
- Early compound microscopes and staining methods produced subcellular features seen by Hooke studying cork cells in 1655 and Leeuwenhoek detecting protozoa and bacteria in the 1670s.
- The Cell Theory was presented by Theodor Schwann and Matthias Schleiden in 1838; Rudolf Virchow subsequently proved that all cells originate from already existing cells.
- Endosymbiosis of primitive prokaryotic cells is thought to have produced eukaryotic organelles like mitochondria and chloroplasts, hence laying a foundation for cellular complexity.
- Membranous and non-membranous forms of organelles are distinguished; membranous examples include endoplasmic reticulum and non-membranous examples comprising ribosomes.
- A double membrane limits the nucleus, which also encloses genetic material and the nucleolus where ribosomal RNA is produced.
- Acting as the cell’s power sources, mitochondria create ATP by oxidative phosphorylation and have an outside and folded inner membrane generating cristae.
- Non-membranous complexes of RNA and protein, ribosomes transform mRNA into polypeptides, hence promoting protein synthesis.
- Originally rough, the endoplasmic reticulum is a network of flattened sacs that synthesizes lipids and detoxifies toxins and contains ribosomes for protein synthesis.
- Stacked cisternae in the Golgi apparatus change, sort and bundle proteins and lipids into vesicles for transport to their targets.
- Peroxisomes comprise oxidases that break down fatty acids and detoxify hydrogen peroxide; lysosomes are single-membrane vesicles carrying hydrolytic enzymes for intracellular digestion.
- Double-membrane organelles with internal thylakoid stacks where photosynthesis turns light energy into chemical energy, chloroplasts found in plant cells
- While the cytoskeleton made of microtubules and filaments gives structural support and intracellular transport, central vacuoles in plant cells preserve turgor pressure and store nutrients.
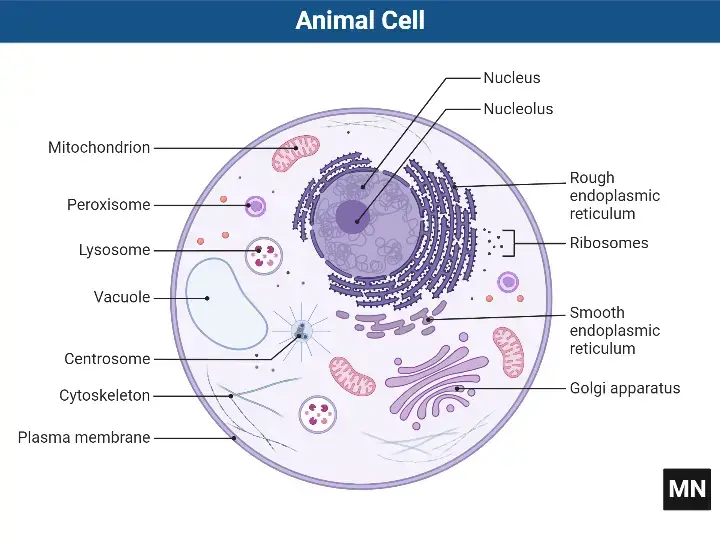
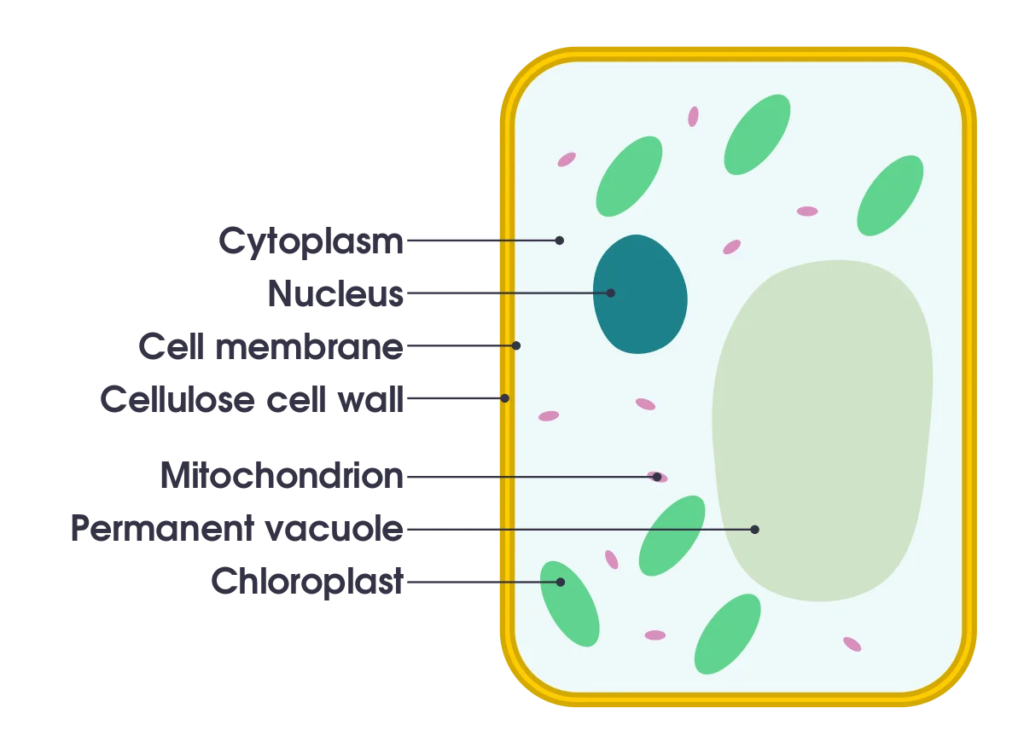
Examples of Cell Organelles
Major eukaryotic organelles
Organelle | Main function | Organisms |
---|---|---|
cell membrane | separates the interior of all cells from the outside environment (the extracellular space) which protects the cell from its environment. | all eukaryotes |
cell wall | The cell wall is a rigid structure composed of cellulose that provides shape to the cell, helps keep the organelles inside the cell, and does not let the cell burst from osmotic pressure. | plants, protists, rare kleptoplastic organisms |
chloroplast (plastid) | photosynthesis, traps energy from sunlight | plants, protists, rare kleptoplastic organisms |
endoplasmic reticulum | translation and folding of new proteins (rough endoplasmic reticulum), expression of lipids (smooth endoplasmic reticulum) | all eukaryotes |
flagellum | locomotion, sensory | some eukaryotes |
Golgi apparatus | sorting, packaging, processing and modification of proteins | all eukaryotes |
mitochondrion | energy production from the oxidation of glucose substances and the release of adenosine triphosphate | most eukaryotes |
nucleus | DNA maintenance, controls all activities of the cell, RNA transcription | all eukaryotes |
vacuole | storage, transportation, helps maintain homeostasis | eukaryotes |
Minor eukaryotic organelles and cell components
Organelle/Macromolecule | Main function | Organisms |
---|---|---|
acrosome | helps spermatozoa fuse with ovum | most animals |
autophagosome | vesicle that sequesters cytoplasmic material and organelles for degradation | all eukaryotes |
centriole | anchor for cytoskeleton, organizes cell division by forming spindle fibers | animals |
cilium | movement in or of external medium; “critical developmental signaling pathway”. | animals, protists, few plants |
cnidocyst | stinging | cnidarians |
eyespot apparatus | detects light, allowing phototaxis to take place | green algae and other unicellular photosynthetic organisms such as euglenids |
glycosome | carries out glycolysis | Some protozoa, such as Trypanosomes. |
glyoxysome | conversion of fat into sugars | plants |
hydrogenosome | energy & hydrogen production | a few unicellular eukaryotes |
lysosome | breakdown of large molecules (e.g., proteins + polysaccharides) | animals |
melanosome | pigment storage | animals |
mitosome | probably plays a role in Iron-sulfur cluster (Fe-S) assembly | a few unicellular eukaryotes that lack mitochondria |
myofibril | myocyte contraction | animals |
nucleolus | pre-ribosome production | most eukaryotes |
ocelloid | detects light and possibly shapes, allowing phototaxis to take place | members of the family Warnowiaceae |
parenthesome | not characterized | fungi |
peroxisome | breakdown of metabolic hydrogen peroxide | all eukaryotes |
porosome | secretory portal | all eukaryotes |
proteasome | degradation of unneeded or damaged proteins by proteolysis | all eukaryotes, all archaea, and some bacteria |
ribosome (80S) | translation of RNA into proteins | all eukaryotes |
stress granule | mRNA storage | most eukaryotes |
TIGER domain | mRNA encoding proteins | most organisms |
vesicle | material transport | all eukaryotes |
Prokaryotic organelles and cell components
Organelle/macromolecule | Main function | Organisms |
---|---|---|
anammoxosome | anaerobic ammonium oxidation | “Candidatus” bacteria within Planctomycetes |
carboxysome | carbon fixation | some bacteria |
chlorosome | photosynthesis | green sulfur bacteria |
flagellum | movement in external medium | some prokaryotes |
magnetosome | magnetic orientation | magnetotactic bacteria |
nucleoid | DNA maintenance, transcription to RNA | prokaryotes |
pilus | Adhesion to other cells for conjugation or to a solid substrate to create motile forces. | prokaryotic cells |
plasmid | DNA exchange | some bacteria |
ribosome (70S) | translation of RNA into proteins | bacteria and archaea |
thylakoid membranes | photosynthesis | mostly cyanobacteria |
1. The cell membrane (Plasma membrane/ Plasmalemma)
The plasma membrane is made up of proteins and lipids. It can fluctuate depending on fluidity and external environment.
Structure of Cell membrane
- Comprising the biological membrane that encloses a cell’s cytoplasm and isolates it from the outside world, the cell membrane—also called the plasma membrane—separates
- It offers a semipermeable barrier that controls waste products, nutrients, and ions, thereby preserving cellular homeostasis.
- In cell communication, adhesion, signal transduction, and energy transduction it is fundamental.
- Red blood cell membrane investigations led Gorter and Grendel in 1925 to suggest a lipid bilayer, which started the study of cell membrane structure.
- Davson and Danielli first presented the trilaminar sandwich concept in 1935 using a phospholipid bilayer bordered by two protein layers.
- Adding protein layers to the lipid bilayer helped to clarify surface tension and permeability characteristics.
- It could not explain the dynamic fluidity and particular transport roles of membranes.
- Based on electron microscopy displaying a trilaminar pattern with dark‑light‑dark layers, Robertson suggested the unit membrane hypothesis in the late 1950s.
- It proposed a universal membrane architecture on both sides wherein a lipid bilayer is sandwiched between protein sheets.
- Based on osmiophilic and osmiophobic layers, it gauged the membrane thickness as between 6.5 and 8.5 nm.
- Singer and Nicolson’s 1972 fluid mosaic model called the membrane a dynamic fluid lipid bilayer with proteins floating inside it.
- It underlined homogeneous mosaic layout and lateral mobility of lipids and proteins.
- Drawing on their interaction with the lipid bilayer, it categorized membrane proteins as integral and peripheral.
- Comprising amphipathic molecules with hydrophilic heads facing aquatic surroundings and hydrophobic tails building the membrane interior, the phospholipid bilayer is the basic structural framework.
- Every phospholipid contains two fatty acid tails coupled to a glycerol phosphate head.
- Interspersed among phospholipids, cholesterol molecules alter membrane fluidity and stability at different temperatures.
- Glycoproteins and glycolipids on the extracellular surface help cells to recognize and communicate by means of carbohydrates.
- Membrane proteins comprise extrinsic peripheral proteins bound to membrane surfaces and intrinsic integral proteins crossing the bilayer.
- Integral proteins are channels, transporters, receptors, or enzymes.
- Participating in cytoskeletal attachments and signal transmission, peripheral proteins
- The two leaflets of the lipid bilayer show asymmetry in both protein distribution and lipid composition.
- Lateral diffusion of lipids and proteins, bending of fatty acid tails, and sporadic flip-flop help to define membrane fluidity and dynamics.
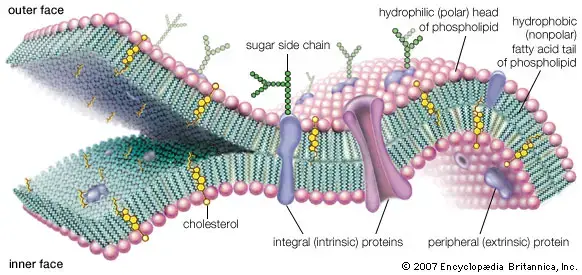
Functions of Cell membrane
- The cell membrane preserves interior components by mechanical support that keeps cell form.
- To maintain cellular homeostasis, it serves as a selectively permeable barrier controlling waste materials, nutrients, and ion entrance and departure.
- It helps to move molecules.
- passive diffusion by osmosis, channel and carrier protein assisted diffusion,
- active movement of ions and molecules against their concentration gradient propelled by ATP-driven pumps
- It includes receptor proteins that start signal transduction paths by sensing extracellular signals like hormones and neurotransmitters.
- On its surface, it has enzymes that, in specialized membranes, catalyze metabolic events including early stages of photosynthesis and respiration.
- Through glycoproteins and glycolipids on the extracellular surface, it contributes in cell identification and adhesion, therefore facilitating cell–cell interactions and immunological responses.
- It grounds the extracellular matrix and cytoskeleton to preserve tissue integrity and offer structural stability.
- By preserving electrochemical gradients utilized in ATP generation in organelles such as mitochondria and chloroplasts, it helps to transduct energy.
- It preserves dynamic fluid mosaic architecture thereby enabling lateral diffusion of lipids and proteins for membrane flexibility, remodeling, and protein interactions.
- It improves the effectiveness of signaling and metabolic pathways by grouping certain lipids and proteins in microdomains, hence separating biological activities.
- It controls membrane fluidity and permeability by means of interspersed cholesterol molecules that control stiffness and phase behavior across temperature fluctuations.
2. Cell Wall
The cell wall is an additional layer that exists outside of the cell membrane. It provides structure, protection, and filtering mechanisms to the cells.
Structure of Cell Wall
- The cell wall is a rigid, semi-permeable structural layer external to the plasma membrane in plants, fungi, bacteria, algae, and some archaea, providing mechanical support and regulating osmotic balance
- In plants, it consists of three layers:
- Middle lamella: outermost layer rich in pectin, cements adjacent cells via calcium cross-links
- Primary cell wall: composed of cellulose microfibrils (linear β-1,4-glucan chains) embedded in a matrix of hemicellulose (e.g., xyloglucan) and pectin, enabling flexibility during cell growth
- Secondary cell wall: formed post-growth, contains cellulose, hemicellulose, and lignin (a phenolic polymer), providing rigidity and hydrophobicity in specialized cells (e.g., xylem)
- Cellulose microfibrils are synthesized by cellulose synthase complexes (rosettes) in the plasma membrane, arranged in a plywood-like network for tensile strength 28
- In plants, it consists of three layers:
- Bacterial cell walls are classified into gram-positive and gram-negative based on peptidoglycan structure
- Gram-positive: thick peptidoglycan (90% of wall) with teichoic acids (polyol-phosphate polymers) covalently linked, contributing to cell shape and antibiotic resistance
- Gram-negative: thin peptidoglycan (5–10%) surrounded by an outer membrane containing lipopolysaccharides (LPS) with lipid A (endotoxin), core polysaccharide, and O-antigen; periplasmic space houses degradative enzymes
- Peptidoglycan structure: alternating N-acetylglucosamine (NAG) and N-acetylmuramic acid (NAM) cross-linked by tetrapeptide bridges (e.g., L-Ala-D-Glu-L-Lys-D-Ala) via transpeptidases targeted by β-lactam antibiotics
- Fungal cell walls are composed of chitin (β-1,4-N-acetylglucosamine), β-glucans (β-1,3 and β-1,6 linkages), and glycoproteins
- Inner layer: chitin and β-1,3-glucan form a rigid scaffold; outer layer contains mannoproteins (glycosylated proteins) for adhesion and immune evasion 410
- Candida albicans: cell wall composition varies between yeast (low chitin) and hyphal forms (high chitin), influencing pathogenicity
- Cryptococcus neoformans: melanin and α-1,3-glucan layers protect against host oxidative stress and phagocytosis
- Algal cell walls differ by species, containing cellulose, glycoproteins, or polysaccharides like carrageenan (red algae) and agar
- Diatoms: cell walls (frustules) composed of biogenic silica (SiO₂·nH₂O) with intricate nanopatterns for structural integrity
- Green algae: cellulose and sulfated polysaccharides (e.g., ulvan) provide flexibility in aquatic environments
- Archaeal cell walls lack peptidoglycan and instead use pseudopeptidoglycan (N-acetyltalosaminuronic acid), glycoprotein S-layers, or polysaccharides for stability
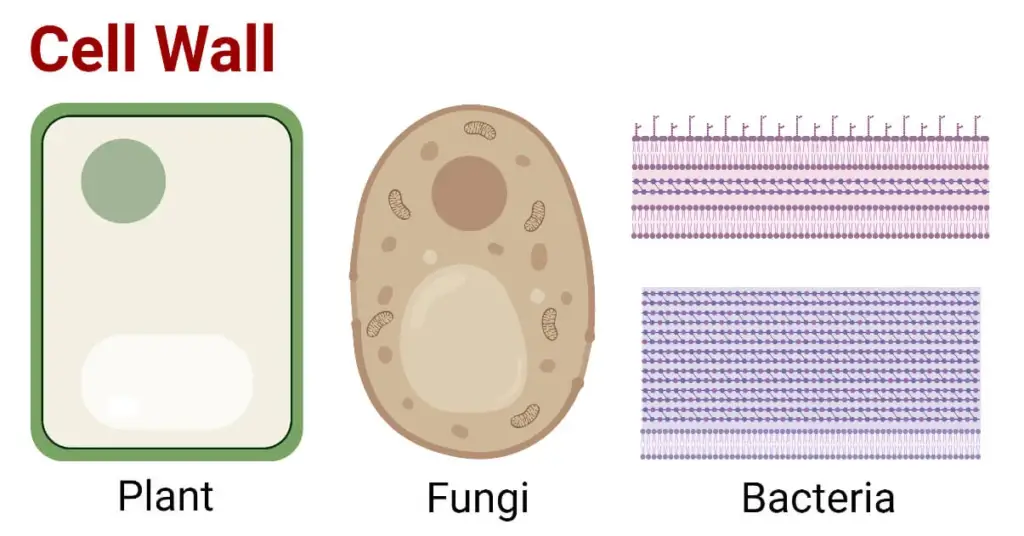
Functions of Cell Wall
- The cell wall is a stiff, extracellular component present in plant, fungal, and bacterial cells. In plants, it predominantly consists of cellulose, hemicellulose, and pectin, fulfilling several vital roles required for cellular viability and plant physiology.
- It imparts mechanical strength and structural integrity, allowing plants to preserve their form and withstand mechanical stress.
- The cell wall modulates turgor pressure by opposing the osmotic pressure of the cell’s contents, therefore averting cell lysis in hypotonic conditions.
- It regulates the orientation of cellular development and expansion, impacting the overall shape of plant tissues.
- The cell wall functions as a selective barrier, regulating the diffusion of substances into and out of the cell, while permitting the transit of vital molecules.
- It enables intercellular communication via plasmodesmata, which are channels linking neighbouring plant cells, for the passage of signalling chemicals and nutrients.
- The cell wall functions as a defensive barrier against diseases, offering physical protection and housing antimicrobial substances that impede pathogen infiltration.
- It inhibits excessive water loss by preserving cellular stiffness and reducing transpiration rates.
- The cell wall is involved in signalling pathways that govern cell division and differentiation, influencing the plant’s development and reaction to external stimuli.
3. Centriole
Centrioles, which are tubular structures found mostly in eukaryotic cells, are mainly composed of the protein tubulin.
Structure of Centriole
- Most animal cells and certain lower plant species have cylindrical organelles called centrioles around their nucleus. Functioning as microtubule-organizing centres (MTOCs), they are essential parts of the centrosome and greatly influence cell division as well as cilia and flagella development.
- Though variants occur with lengths ranging from 0.16 μm to as long as 8 μm, each centriole is around 0.15–0.25 μm in diameter and 0.3–0.7 μm in length.
- A centriole’s structural arrangement consists in nine sets of microtubule triplets stacked cylindrical fashion. Essential for its function in cellular processes, this arrangement gives the centriole both flexibility and strength.
- Centrioles include a core cartwheel structure, made of a central hub with nine spokes extending outward. Every one of them links to a microtubule triplet by a pinhead, which helps the centriole to be stable and orderly.
- Usually located in pairs and orientated at right angles to one another within the centrosome, centrioles Their function during cell division depends critically on this orthogonal configuration.
- Surrounded by the centrioles, the pericentriolar material (PCM) is high in proteins required for microtubule nucleation and anchoring, therefore helping to produce the mitotic spindle during cell division.
- Though they lack DNA and a surrounding membrane, centrioles may multiply on their own during the cell cycle. Tight control of this replication guarantees appropriate cell division and function.
- Apart from their part in cell division, centrioles can develop into basal bodies, which are necessary for the development and operation of cilia and flagella, therefore supporting cell movement and sensory activities.
- Most higher plant cells, some fungus, and some protists lack centrioles, suggesting that other systems for microtubule organisation and cell division must exist in these animals.
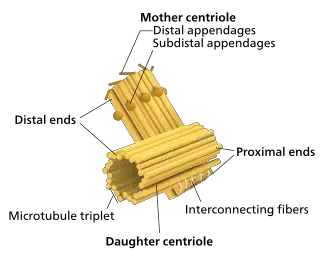
Functions of Centriole
- Found mostly in animal cells, centrioles are cylindrical organelles made of microtubules that are essential for many different kinds of cellular activity.
- They are crucial for arranging microtubules during cell division and for helping the mitotic spindle to develop, therefore guaranteeing correct chromosomal separation.
- Basal bodies, centrioles anchor and start the cilia and flagella assembly, structures essential for cell movement and sensory activities.
- Their spatial arrangement and orientation help other organelles inside the cell to be positioned, therefore affecting cellular structure and function.
- Independent of DNA replication, centrioles make sure each daughter cell inherits a pair during cell division.
- They help to define the beating pattern of cilia and flagella, therefore affecting the capacity of the cell to react to external stimuli.
- Crucially for sperm movement, the distal centriole forms the axial filament or tail in sperm cells.
- During cell division, centrioles help to properly orient and separate chromosomes, therefore facilitating the development of the aster.
- Most higher plant cells lack them, suggesting other means of microtubule arrangement in these species.
4. Cilia and Flagella
Flagella and Cilia are microtubule-based projections of the cell that look like hairs. They are covered by the plasma membrane.
Structure of Cilia and Flagella
- Mostly used in movement and sensory purposes, cilia and flagella are hair-like appendages projecting from the surface of eukaryotic cells. Their fundamental basis is shared even if their lengths and numbers differ. ,
- The axoneme, which has a “9+2” configuration of microtubules—nine peripheral doublets around two central singlets—defines the basic structure of both cilia and flagella.
- Every peripheral microtubule doublet comprises one full microtubule (A-tubule) and one imperfect microtubule (B-tubule).
- Attached to the A-tubules, dynein arms act as motor proteins breaking ATP to create sliding forces between neighboring microtubule doublets, producing cilia and flagella’s bending motion.
- Radial spokes, which help to control dynein arm activity and preserve axoneme structural integrity, stretch from each peripheral doublet toward the center pair.
- Nexin joins neighboring microtubule doublets to provide elastic resistance to the sliding forces produced by dynein, hence transforming sliding into bending.
- The plasma membrane ensheathes the whole axoneme, creating flagella and cilia extensions of the cell surface.
- Comprising nine triplet microtubules, each cilium or flagellum’s basal body is physically identical to a centriole. The axoneme is first assembled by the basal body, which also binds it to the cell.
- Lacking the middle pair of microtubules and dynein arms, primary (non-motile) cilia have a “9+0” axonemal structure and are mostly involved in sensory processes.
- Effective locomotion and fluid movement across cellular surfaces are made possible by the coordinated activity of dynein arms, radial spokes, and nexin linkages facilitating the unique beating patterns of cilia and flagella.
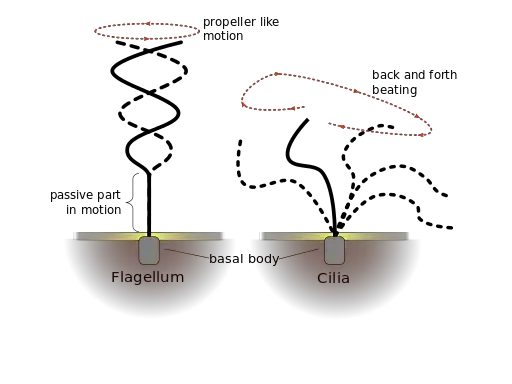
Functions of Cilia and Flagella
- Cilia and flagella perform diverse biological roles categorized into motile, sensory, and developmental functions
- Motility and fluid dynamics
- Propel cells through liquid environments (e.g., sperm flagella enable swimming toward the egg)
- Move extracellular fluids via coordinated beating (e.g., respiratory cilia clear mucus and pathogens; fallopian tube cilia transport ova)
- Generate unidirectional flow of cerebrospinal fluid in brain ventricles (ependymal cell cilia)
- Sensory signal transduction
- Detect chemical signals (e.g., olfactory cilia bind odorants; primary cilia in kidneys sense urinary flow and solute concentrations)
- Mediate mechanosensation (e.g., hair cells in the inner ear detect sound vibrations via stereocilia, a specialized cilium subtype)
- Facilitate phototransduction in retinal photoreceptors (modified cilia house light-sensitive proteins like rhodopsin)
- Regulate developmental signaling pathways (e.g., Hedgehog signaling in primary cilia controls tissue patterning; PDGFRα signaling in fibroblasts)
- Developmental roles
- Establish left-right body asymmetry during embryogenesis (nodal cilia create leftward fluid flow, activating genetic cascades)
- Guide cell migration in tissue morphogenesis (e.g., cilia direct neural crest cell movement)
- Motility and fluid dynamics
- Specialized motility patterns
- Planar beating of motile cilia (e.g., metachronal waves in tracheal epithelia for mucus escalator function)
- Helical undulation of eukaryotic flagella (e.g., sperm flagella generate whip-like thrust)
- Rotary motion in prokaryotic flagella (distinct from eukaryotic flagella; spin like propellers to drive bacterial movement)
- Cell cycle regulation
- Primary cilia disassemble before mitosis to free centrioles for spindle formation
- Ciliary resorption linked to cell cycle checkpoints (prevents conflicting signaling during division)
- Immune and homeostatic functions
- Respiratory cilia trap and expel pathogens, contributing to innate immunity
- Renal primary cilia monitor urine composition, regulating electrolyte balance and blood pressure
- Clinical functional impairments
- Primary ciliary dyskinesia (motility defects cause chronic sinusitis, bronchiectasis, and situs inversus in Kartagener syndrome)
- Polycystic kidney disease (loss of flow sensing in renal cilia leads to uncontrolled cyst growth)
- Retinal degeneration (mutations in ciliary proteins disrupt photoreceptor maintenance, causing retinitis pigmentosa)
- Evolutionary adaptations
- Conservation of sensory functions across eukaryotes (e.g., Chlamydomonas flagella detect light and chemicals)
- Protozoan predation and environmental navigation (e.g., Paramecium cilia mediate feeding and escape responses)
- Energy utilization
- Dynein ATPase activity consumes ATP for microtubule sliding in motile structures
- Sensory cilia maintain low energy demands, relying on ion gradients for signal amplification
- Research and diagnostic relevance
- Sperm flagellar motility assays assess male fertility
- Ciliary beat frequency analysis diagnoses respiratory disorders
- Primary cilia studies inform therapies for cystic diseases and cancer (targeting Hedgehog signaling pathways)
- Ecological interactions
- Algal flagella enable phototaxis (movement toward light for photosynthesis)
- Ciliated planktonic organisms influence aquatic nutrient cycles through motility and feeding behaviors
5. Chloroplast
A chloroplast, a type of plastic, is involved in photosynthesis in plants or algae. Chloroplast has a vital pigment called chlorophyll that traps sunlight to produce glucose.
Structure of Chloroplast
- Chloroplasts are double-membrane-bound organelles found in plant cells and algae, responsible for photosynthesis and other metabolic processes
- Outer membrane: permeable to small molecules due to porin proteins
- Inner membrane: selectively permeable, contains transport proteins for metabolite exchange
- Intermembrane space: narrow region between the two membranes
- Stroma: the fluid-filled space inside the inner membrane
- Contains enzymes for the Calvin cycle (light-independent reactions of photosynthesis)
- Houses chloroplast DNA, ribosomes (70S type), starch granules, and lipid droplets
- Site for synthesis of fatty acids, amino acids, and other metabolites
- Thylakoid system: membrane-bound compartments where light-dependent reactions occur
- Thylakoids: flattened, disc-like sacs containing photosynthetic pigments
- Grana (singular: granum): stacks of 10-20 thylakoids
- Stroma lamellae: tubular connections between grana
- Thylakoid lumen: aqueous space inside thylakoids where proton accumulation occurs
- Photosynthetic pigments and protein complexes:
- Chlorophyll a and b: primary pigments that absorb light energy
- Carotenoids (beta-carotene, xanthophylls): accessory pigments that protect against photo-oxidation
- Photosystem I and II: protein-pigment complexes that capture and transfer light energy
- Cytochrome b6f complex: mediates electron transport between photosystems
- ATP synthase: enzyme complex that generates ATP using proton gradient
- Chloroplast DNA and ribosomes:
- Circular DNA (120-170 kb) encoding approximately 120 genes
- Genes mainly code for components of the photosynthetic apparatus and protein synthesis machinery
- 70S ribosomes (similar to bacterial ribosomes) for protein synthesis
- Plastoglobuli: lipid droplets in the stroma
- Store lipids, carotenoids, and tocopherols (vitamin E)
- Involved in chloroplast membrane maintenance and stress response
- Starch grains: temporary storage of photosynthetic products
- Formed in the stroma during daylight hours
- Broken down at night to provide energy for cellular processes
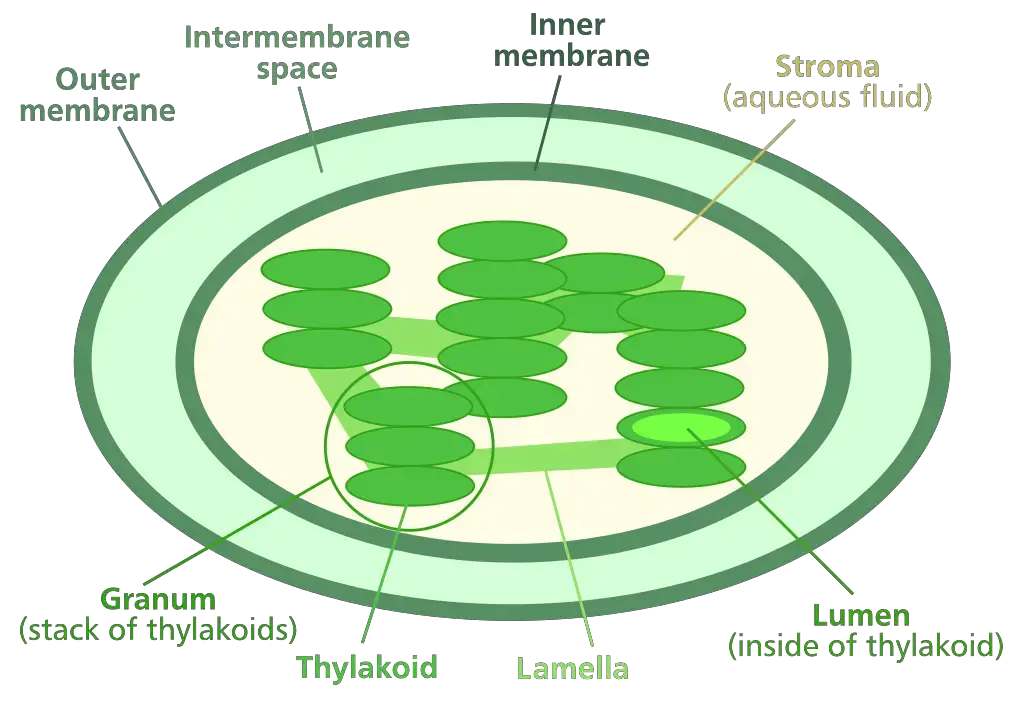
Functions of Chloroplast
- Conducts photosynthesis to convert light energy into chemical energy (ATP and NADPH)
- Light-dependent reactions occur in thylakoid membranes
- Photosystem II splits water (photolysis) to release oxygen, protons, and electrons
- Photosystem I produces NADPH using electrons and hydrogen ions
- ATP synthase generates ATP via proton gradient across thylakoid membrane
- Light-independent reactions (Calvin cycle) occur in the stroma
- Fixes atmospheric CO₂ into glucose using ATP and NADPH
- Rubisco enzyme catalyzes CO₂ fixation with ribulose bisphosphate (RuBP)
- Light-dependent reactions occur in thylakoid membranes
- Synthesizes and stores photosynthetic pigments
- Chlorophyll a and b in thylakoid membranes absorb blue and red light wavelengths
- Carotenoids (e.g., beta-carotene, xanthophylls) protect against photodamage by dissipating excess light energy
- Produces oxygen as a byproduct of water photolysis in photosystem II
- Critical for aerobic respiration in plants and most organisms
- Regulates carbon metabolism and starch synthesis
- Temporary storage of starch granules in the stroma during the day for nighttime energy use
- Converts excess glucose into starch to maintain osmotic balance
- Participates in nitrogen assimilation
- Enzymes in the stroma reduce nitrate (NO₃⁻) to ammonium (NH₄⁺) for amino acid synthesis
- Generates lipid precursors for membrane synthesis
- Fatty acids and glycerolipids produced in the stroma contribute to thylakoid and chloroplast membrane formation
- Facilitates plant immune responses
- Produces reactive oxygen species (ROS) as signaling molecules during pathogen attacks
- Synthesizes defense-related compounds like salicylic acid
- Maintains chloroplast autonomy through semi-independent replication
- Contains circular DNA encoding rRNA, tRNA, and proteins essential for photosynthesis
- Divides via binary fission-like processes regulated by nuclear and chloroplast genomes
- Supports evolutionary adaptations in plants
- Chloroplast genome structure (e.g., inverted repeats) preserves gene order and function across plant species
- Endosymbiotic origin explains shared features with cyanobacteria (e.g., prokaryotic ribosomes, unstacked DNA)
- Regulates cellular redox state
- Balances ATP/NADPH ratios to prevent oxidative stress during fluctuating light conditions
- Thioredoxin system in stroma activates Calvin cycle enzymes via redox signaling
- Interacts with mitochondria and peroxisomes in photorespiration
- Recycles phosphoglycolate into usable metabolites during high O₂/low CO₂ conditions
6. Cytoplasm
The cytoplasm is everything inside a cell, except for the nucleus. The cytoplasm is found in animal and plant cells. They are jelly-like compounds, located between the cell membrane and the nucleus. They’re mostly made up of organic and inorganic substances. The cytoplasm is among the fundamental cells in which all organelles within the cell reside. Cell organelles are home to enzymes that are responsible for controlling the metabolic activity that takes in the cell. They are the location for the majority reaction reactions that occur within cells.
Structure of Cytoplasm
- Cytoplasm is a viscous, translucent substance filling the cell interior between the plasma membrane and the nuclear envelope
- Comprises three primary components: cytosol (liquid matrix), organelles, and cytoplasmic inclusions
- Acts as the medium for biochemical reactions, intracellular transport, and structural support
- Cytosol (cytoplasmic matrix) constitutes ~70% of the cell volume
- Composed primarily of water (70-80%), ions (K⁺, Na⁺, Cl⁻), enzymes, carbohydrates, lipids, and proteins
- Contains high concentrations of macromolecules (150-300 mg/mL), creating macromolecular crowding that influences reaction kinetics
- Maintains pH 7.0-7.4 through buffering systems involving bicarbonate and phosphate ions
- Cytoplasmic organelles are membrane-bound or non-membrane-bound structures with specialized functions
- Mitochondria
- Double-membrane structure with cristae (inner membrane folds) and matrix containing mitochondrial DNA
- Site of aerobic respiration and ATP synthesis via the citric acid cycle and electron transport chain
- Endoplasmic reticulum (ER)
- Rough ER: Ribosome-studded membranes for protein synthesis and initial post-translational modifications
- Smooth ER: Lipid synthesis, detoxification, and calcium ion storage
- Ribosomes
- 70S in prokaryotes (50S + 30S subunits), 80S in eukaryotes (60S + 40S subunits)
- Composed of rRNA and proteins; synthesize polypeptides via mRNA translation
- Golgi apparatus
- Stacked cisternae modifying, sorting, and packaging proteins for secretion or lysosomal creation
- Lysosomes
- Contain 40+ hydrolytic enzymes (optimal pH 4.5-5.0) for macromolecule degradation and autophagy
- Cytoskeleton
- Microfilaments (7 nm): Actin polymers enabling cell motility and cytokinesis
- Intermediate filaments (10 nm): Keratin/lamins providing mechanical strength
- Microtubules (25 nm): Tubulin dimers facilitating intracellular transport and mitotic spindle formation
- Mitochondria
- Cytoplasmic inclusions are non-membrane-bound transient structures
- Nutrient reserves: Glycogen granules (hepatocytes), lipid droplets (adipocytes)
- Pigments: Melanin in melanocytes, lipofuscin in aging cells
- Crystals: Cholesteryl esters in adrenal cortex cells
- Physical properties demonstrate dynamic behavior
- Undergoes sol-gel transitions regulated by calcium concentrations and ATP availability
- Exhibits cytoplasmic streaming (cyclosis) in plant cells (e.g., Chara spp.) at speeds up to 100 μm/sec
- Viscosity ranges from 1-3 cP (similar to water) in sol state to >10,000 cP in gel state
- Functional roles extend beyond passive substance containment
- Facilitates signal transduction through controlled ion gradients (Ca²⁺ waves)
- Enables metabolic channeling via enzyme complexes (e.g., pyruvate dehydrogenase complex)
- Maintains cellular homeostasis through selective molecular sequestration in phase-separated condensates
- Prokaryotic cytoplasm differs structurally from eukaryotic cytoplasm
- Lacks membrane-bound organelles but contains ribosomes (70S) and proteinaceous microcompartments
- Nucleoid region contains supercoiled chromosomal DNA attached to the plasma membrane
- Carboxysomes in cyanobacteria concentrate CO₂ for RuBisCO activity in photosynthesis
- Experimental techniques for cytoplasmic analysis
- Differential centrifugation isolates cytoplasmic components based on density/size
- Fluorescence recovery after photobleaching (FRAP) measures macromolecule mobility
- Cryo-electron tomography reveals native-state organelle interactions at 4 nm resolution
- Pathological alterations reflect cytoplasmic sensitivity to stressors
- Hypoxia induces cytoplasmic vacuolization through ER and mitochondrial swelling
- Viral infections often create viral factories (e.g., poxvirus factories) disrupting normal organelle distribution
- Protein aggregation in cytoplasm characterizes neurodegenerative diseases (e.g., ALS-linked TDP-43 inclusions)
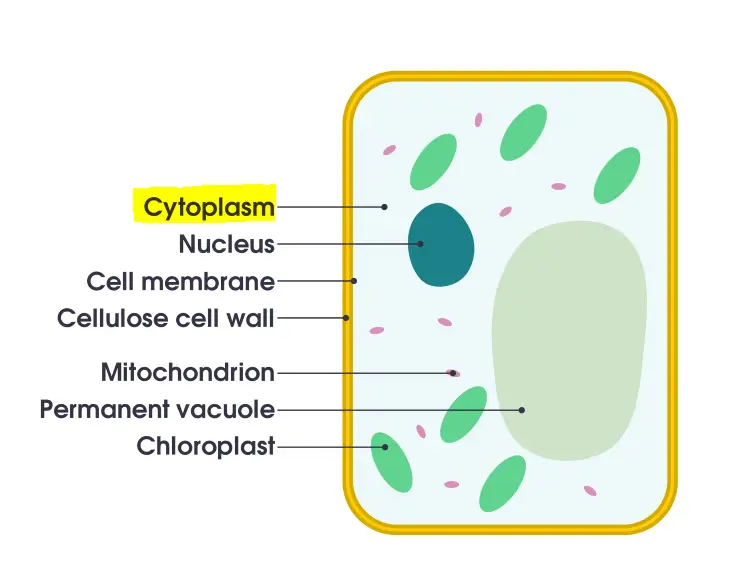
Functions of Cytoplasm
- Serves as the primary site for metabolic reactions essential to cellular survival
- Glycolysis occurs in the cytosol, breaking glucose into pyruvate (yielding 2 ATP per molecule)
- Fatty acid synthesis and the pentose phosphate pathway occur in the cytoplasmic matrix
- Enzymes in the cytosol catalyze amino acid activation for protein synthesis
- Facilitates intracellular transport and molecular trafficking
- Cytoskeletal networks (microtubules, actin filaments) guide vesicle movement via motor proteins (kinesin, dynein, myosin)
- Facilitated diffusion and active transport mechanisms maintain ion gradients (e.g., Na⁺/K⁺ ATPase pumps)
- Endoplasmic reticulum-to-Golgi vesicle shuttling relies on cytoplasmic motor-protein interactions
- Maintains cellular structure and mechanical integrity
- Cytoskeleton provides tensile strength through intermediate filaments (e.g., vimentin in mesenchymal cells)
- Actin-myosin interactions enable cell motility (lamellipodia formation) and cytokinesis via contractile ring assembly
- Turgor pressure in plant cells is regulated by cytoplasmic water content and vacuolar osmolytes
- Stores reserves and critical molecules for cellular operations
- Glycogen granules in hepatocytes and muscle cells provide rapid glucose mobilization
- Lipid droplets store triglycerides and cholesterol esters for membrane synthesis and energy production
- Calcium ion reservoirs in the ER lumen (up to 10⁻³ M) enable rapid signaling during muscle contraction
- Enables signal transduction and regulatory processes
- Secondary messengers (cAMP, IP₃) diffuse through the cytosol to amplify extracellular signals
- Scaffolding proteins organize kinase cascades (e.g., MAPK pathways) in spatially defined cytoplasmic domains
- Calcium waves propagate through the cytoplasm to synchronize cellular responses (e.g., fertilization in oocytes)
- Supports cell division and genetic material segregation
- Cytoplasmic factors regulate mitotic spindle assembly and chromosome separation
- Asymmetric organelle distribution during cytokinesis ensures daughter cell viability
- Midbody formation in the cytoplasm mediates abscission during telophase
- Participates in waste management and detoxification
- Peroxisomes in the cytoplasm oxidize fatty acids and neutralize reactive oxygen species (ROS) via catalase
- Proteasomes degrade ubiquitin-tagged misfolded proteins to prevent cytotoxic aggregation
- Xenobiotic metabolism in hepatocyte cytoplasm involves cytochrome P450 enzymes (phase I reactions)
- Mediates environmental adaptation and stress responses
- Heat shock proteins (HSP70) in the cytoplasm refold denatured proteins during thermal stress
- Phase-separated condensates (stress granules) sequester untranslated mRNA during nutrient deprivation
- Cryoprotectant synthesis (e.g., trehalose in yeast) prevents cytoplasmic ice crystal formation during freezing
- Facilitates interorganelle communication and membrane contact sites
- Mitochondria-associated ER membranes (MAMs) enable lipid transfer and calcium signaling
- Lysosome-ER contacts regulate autophagosome formation during nutrient stress
- Lipid droplets interact with peroxisomes and mitochondria for β-oxidation coordination
- Prokaryotic cytoplasmic functions differ in organization but retain core metabolic roles
- Transcription-translation coupling occurs in the nucleoid-adjacent cytoplasm due to absent nuclear compartmentalization
- Plasmid replication and partitioning rely on cytoplasmic ParA-ParB ATPase systems
- Gas vesicles in aquatic prokaryotes regulate buoyancy via cytoplasmic protein assembly
- Pathological disruptions highlight critical functional dependencies
- Amyotrophic lateral sclerosis (ALS) involves cytoplasmic TDP-43 aggregates impairing RNA metabolism
- Viral replication complexes (e.g., SARS-CoV-2) hijack cytoplasmic machinery for viral RNA synthesis
- Ischemia-induced cytoplasmic acidosis (pH <6.5) disrupts enzyme activity and induces necrotic cell death
- Experimental insights into cytoplasmic functions
- Green fluorescent protein (GFP) tagging reveals real-time organelle dynamics and protein localization
- Atomic force microscopy measures cytoplasmic elasticity changes during metastatic transformation
- Metabolomic profiling identifies cytoplasmic metabolic flux alterations in cancer (Warburg effect)
7. Cytoskeleton
The cytosol contains a number of fibrous structures that help give shape to cells and support cellular transport. It is a continuous system of filamentous proteinsaceous structures that extend throughout the cytoplasm starting from the nucleus and ending at the plasma membrane. It is present in all living cells, and is particularly present in eukaryotes.
The cytoskeleton matrix comprises of a variety of proteins that are able to divide quickly or break down depending on the needs of cells. The main function of the cytoskeleton matrix is providing the form and resistance of cells against deformation. The contractile nature of the filaments aids in cytokinesis as well as in motility.
Structure of Cytoskeleton
- The cytoskeleton is a dynamic network of protein filaments maintaining cell shape, enabling motility, and organizing intracellular components
- Comprises three primary filament systems: microfilaments (actin filaments), intermediate filaments, and microtubules
- Each filament type differs in composition, mechanical properties, and functional specialization
- Microfilaments (actin filaments)
- Diameter: 6-7 nm, composed of globular actin (G-actin) subunits forming helical F-actin polymers
- Exhibit structural polarity with fast-growing (+) and slow-growing (-) ends regulated by ATP hydrolysis
- Form cortical networks beneath plasma membrane (cell cortex) and specialized structures:
- Stress fibers: Contractile actomyosin bundles in adherent cells
- Lamellipodia/filopodia: Membrane protrusions driving cell migration
- Dynamic assembly/disassembly enables rapid structural remodeling during cytokinesis and phagocytosis
- Intermediate filaments
- Diameter: 10 nm, composed of tissue-specific proteins (e.g., keratins, vimentin, lamins, neurofilaments)
- Non-polar rope-like structure with high tensile strength due to staggered tetrameric assembly
- Mechanical stabilization roles:
- Nuclear lamina (lamin filaments) reinforcing nuclear envelope
- Keratin networks in epithelial cells resisting shear stress
- Neurofilaments in axons maintaining neuronal caliber
- More stable than other filaments, with half-lives exceeding 24 hours
- Microtubules
- Diameter: 25 nm, hollow tubes composed of α/β-tubulin heterodimers arranged inprotofilaments
- Exhibit dynamic instability: Stochastic growth/shrinkage phases regulated by GTP hydrolysis
- Structural and functional polarity:
- Plus end (+): Rapid tubulin addition, oriented toward cell periphery
- Minus end (-): Anchored at microtubule-organizing centers (MTOCs) like centrosomes
- Key organizational roles:
- Mitotic spindle formation during cell division
- Axonal transport via motor proteins (kinesin/dynein)
- Cilia/flagella structure with 9+2 microtubule arrangement
- Accessory proteins regulate cytoskeletal dynamics
- Motor proteins:
- Myosins (actin-based): Drive muscle contraction and vesicle transport
- Kinesins/dyneins (microtubule-based): Transport organelles/chromosomes
- Crosslinking proteins:
- Filamin (actin): Forms orthogonal networks
- MAPs (microtubule-associated proteins): Stabilize microtubules
- Severing proteins:
- Cofilin (actin) and katanin (microtubules) promote filament disassembly
- Motor proteins:
- Supramolecular organization
- Centrosomes: MTOCs containing γ-tubulin ring complexes for microtubule nucleation
- Focal adhesions: Integrin-mediated actin connections to extracellular matrix
- Desmosomes/hemidesmosomes: Intermediate filament anchoring at cell-cell/cell-matrix junctions
- Evolutionary conservation and diversity
- Prokaryotic homologs:
- MreB (actin-like) maintains rod-shaped bacteria
- FtsZ (tubulin-like) mediates cell division
- Eukaryotic specialization:
- Muscle-specific actin-myosin arrays
- Neuron-specific microtubule-associated tau proteins
- Prokaryotic homologs:
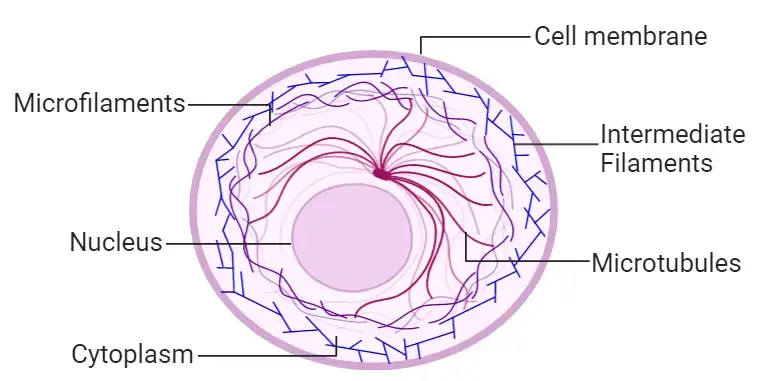
Functions of Cytoskeleton
- Preserves structural integrity and cell form
- Actin filaments withstand deformation by forming cortical networks under plasma membrane.
- Tensile strength—that which intermediate filaments provide—that of keratins in epithelial cells
- Microtubules oppose compression forces, therefore preserving axon structure in neurones.
- Promotes cell motility and contractility.
- Actin polymerisation promotes lamellipodia/filopodia membrane protrusions during migration.
- Actin is interacted with by myosin motor proteins to allow muscle contraction and cytokinesis.
- Movement of cilia or flagella by dynein-mediated microtubule sliding (9+2 axoneme structure)
- Promotes intracellular mobility
- Motor proteins (kinesins/dyneins) use microtubules as rails to move vesicles/organelles
- Actin filaments direct short-range vesicle migration close to the plasma membrane
- Axon neurofilament control of organelle location and axonal width
- Arranges cell components in space.
- Centrosomes place organelles (e.g., Golgi apparatus) by anchoring microtubule minus ends.
- Actin networks separate cytoplasmic elements during oocyte polarisation.
- Interactive filaments link nuclear envelope to cell-matrix adhesions.
- Governs cell division.
- During mitosis, mitotic spindle—microtubules—separates chromosomes.
- Daughter cells are cleaved during cytokinesis by contractile ring (actin-myosin).
- Duplication of centrosomes guarantees correct spindle development.
- helps signal transduction.
- Mechanotransduction: LINC complexes lets cytoskeleton send mechanical signals to nucleus.
- Scaffolds signalling molecules (e.g., MAPK cascades attached to microtubules)
- Extensive matrix signals are transformed into biochemical reactions via integrin-actin interactions.
- facilitates specific cell activities.
- Actin shapes membrane to capture particles in phagocytosis.
- T-cell microtubules polarise secretory machinery towards target cells in immune synapses.
- Cortical microtubules direct cellulose synthase placement in plant cell walls.
- Preserves nuclear architecture.
- Nuclear lamina, sometimes known as lamin intermediate filaments, stabilises nuclear envelope.
- Nesprin proteins connect cytoplasmic cytoskeleton to nucleoskeleton.
- controls chromosome alignment during gene expression.
- reacts under injury and stress.
- Under mechanical tension, intermediate filaments reorganise to prevent rupture.
- Mechanisms of membrane repair motivated by actin seal lesions on a plasma membrane
- Microtubules help autophagy machinery move broken organelles.
- Adaptations for evolution
- Plant cells reposition dynamic microtubule arrays in response to phototropism.
- Maintaining cell form and guiding peptidoglycan production, bacterial actin homologs (MreB)
- Apicomplexan parasites host invasion using actin-based gliding motility.
- Pathological ties
- Tau protein aggregation alters microtubule stability (Alzheimer’s).
- Keratin mutations induce skin blistering in epidermolysis bullosa.
- Altered actin dynamics improve cell motility across tissues, hence promoting cancer metastases.
- Experimental analysis instruments
- Dynamic rearrangements are shown by live-cell imaging with fluorescent tags (e.g., GFP-actin).
- Cytochalasin D (actin depolymerizer), Nocodazole (microtubule disruptor) pharmacological inhibitors
- Force of traction Microscope measures mechanical forces produced by cytoskeleton
8. Endoplasmic Reticulum (ER)
Endoplasmic means inside (endo) the cytoplasm(plasm). The Latin word for the net is reticulum. An endoplasmic reticulum is a plasma membrane that forms inside a cell. It folds inwards to create an inner space called the lumen. The lumen is actually continuous and is connected to the perinuclear area.
The Endoplasmic Reticulum, a network membranous canals that are filled with fluid, is the largest of these. They act as the transport system for the cell and transport materials within the cell.
- Rough Endoplasmic Reticulum – These are made up of cisternae and tubules and vesicles that are present throughout the cell and involved in protein production.
- Smooth Endoplasmic Reticulum – These are the storage organelles, which are associated with the production and use of steroids, lipids, and detoxification.
Structure of Endoplasmic Reticulum (ER)
- From the outer nuclear membrane throughout the cytoplasm, the endoplasmic reticulum is a continuous membranous network of linked flattened sacs, vesicles, and tubules.
- About 5–6 nm thick, the phospholipid bilayer membrane of the ER has a fluid-mosaic arrangement.
- Embedded in ER membranes are stearases, NADH-cytochrome reductase, and glucose-6‑phosphatase needed for different synthesis processes.
- A continuous internal compartment with a diameter ranging from 30 to 50 nm, the ER lumen provides a channel for protein and lipid movement.
- Three primary morphological substructures called cisternae, vesicles, and tubules are formed by the ER.
- Usually 40–50 nm in width, cisternae are long, flattened sac-like unbranched membranes stacked in parallel.
- Small, approximately spherical membrane-bound objects (~25–50 nm radius) called vesicles bud from ER membranes to carry cargo.
- Highly curved, linked cylindrical membranes called tubules generate a vast three-dimensional network.
- Rough ER appears granular and helps co-translational protein translocation by being composed of flattened sheets studded with ribosomes on the cytosolic surface.
- Temporary binding of ribosomes to the RER translocon complex converts proteins straight into the ER lumen.
- Continuous with the outside nuclear envelope, rough ER sheets are joined by helical Terasaki ramps like those of a parking garage.
- Smooth ER consists in a tubular network devoid of ribosomes engaged in detoxification, calcium storage, and lipid synthesis.
- About 30–50 nm in diameter, SER tubules are maintained by membrane-shaping proteins including REP and reticulons.
- Forming membrane contact sites with mitochondria and other organelles to enable lipid exchange, it is contiguous with RER and the nuclear envelope.
- ER membranes are fluid and dynamic, remodelled by fusion and fission processes under control of ER‑shaping proteins and the cytoskeleton.
- Reticulons and DP1/Yop1 family proteins generate tubules and membrane curvature.
- Interactions with actin and microtubules help to distribute ER around the cell, hence driving ER network dynamics.
- Key component of the endomembrane system, the ER is continuous with the nuclear envelope and functionally connected to the Golgi apparatus by COPII‑ and COPI‑coated vesicle trafficking.
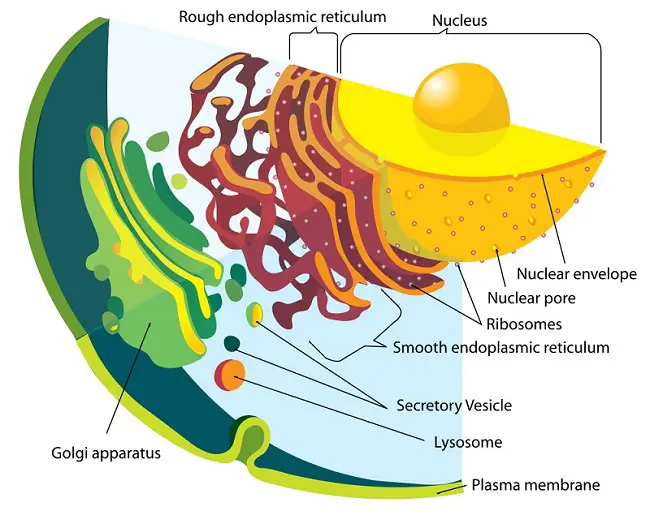
Functions of Endoplasmic Reticulum (ER)
- Rough ER activities comprise the synthesis, folding, modification, and transport of proteins intended for secretion, the plasma membrane, or organelles like lysosomes.
- Rough ER helps transmembrane proteins to be inserted and phospholipids to be synthesized needed for membrane biogenesis in situ.
- Resident chaperones like as BiP govern the quality of newly produced polypeptides by guaranteeing correct folding and thereby avoiding aggregation.
- Smooth ER activities cover the production of lipids, including steroid hormones, cholesterol, and phospholipids.
- Crucially important in carbohydrate metabolism and blood sugar control, the enzyme glucose‑6‑phosphatase in the smooth ER transforms glucose‑6‑phosphatase to glucose.
- Cytochrome P450 enzymes buried in the smooth ER membrane mediate the detoxification of xenobiotics and pharmaceuticals, hence improving solubility of toxins for excretion.
- Specialized smooth ER or sarcoplasmic reticulum stores and releases calcium ions in muscle cells to control contraction cycles.
- The ER’s overall roles include serving as a continuous conduit between the cytoplasm and the nuclear envelope to enable effective protein and lipid movement.
- COPII‑coated vesicles that convey cargo to the Golgi apparatus find budding sites at transional ER exit sites.
- Non-vesicular lipid transport and inter-organelle signaling are facilitated by ER with mitochondria, Golgi, and plasma membrane contact sites.
- Supporting cell development and organelle maintenance, the ER membrane is the key location for the production of transmembrane proteins and lipids destined for all endomembrane compartments.
9. Endosomes
Endosomes are membrane-bound cells within cells that are derived from the Golgi network
Structure of Endosomes
- In eukaryotic cells, endosomes are membrane-bound organelles used as sorting centers in the endocytic pathway, gathering cargo from the plasma membrane and Golgi apparatus.
- Early endosomes, late endosomes (multivesicular bodies/MVBs), and recycling endosomes include three main forms.
- Early endosomes: tubular-vesicular structures surrounded by the plasma membrane distinguished by phosphatidylinositol-3-phosphate (PI3P). Rab5
- Rab7 and phosphatidylinositol-3,5-bisphosphate (PI(3,5)P₂) define spherical organelles containing intraluminal vesicles (ILVs), late endosomes/MVBs
- Recycling endosomes: characterized by Rab11, tubular structures returning goods to the plasma membrane
- Structural maturation consists of consecutive changes.
- Early endosomes develop from fusion of endocytic vesicles and mature into late endosomes by acidification (pH ~6.0 to ~5.0) and Rab5-to–Rab7 GTPase switching.
- Mediated by ESCRT complexes (ESCRT-0 to III), intraluminal vesicles (ILVs) develop inwards from inward budding of the endosomal membrane
- Maturation involves ILV buildup and loss of tubular extensions that results in multivescular morphology.
- Key lipid composition and molecular indicators
- PI3P, clathrin-coated areas, and Rab5/EEA1 proteins—early endosomes
- PI(3,5)P₂, lysobisphosphatidic acid (LBPA), and Rab7 late endosomes
- Recycling endosomes: cargo return 59 from Rab11 and tubular extensions
- structural elements tailored for a plant
- In plants, the early endosome is the trans-golgi network (TGN), which lacks typical tubular-vesicular form.
- Plant MVBs sort ubiquitinated cargo via different ways but lack ESCRT-0.
- Ultrastructural features shown via electron microscopy
- Early endosomes: electron-lucent vacuoles devoid of clathrin-coated areas and few ILVs
- Dense vesicles (250–1000 nm diameter) containing 30–60 nm ILVs are late endosomes or MVBs.
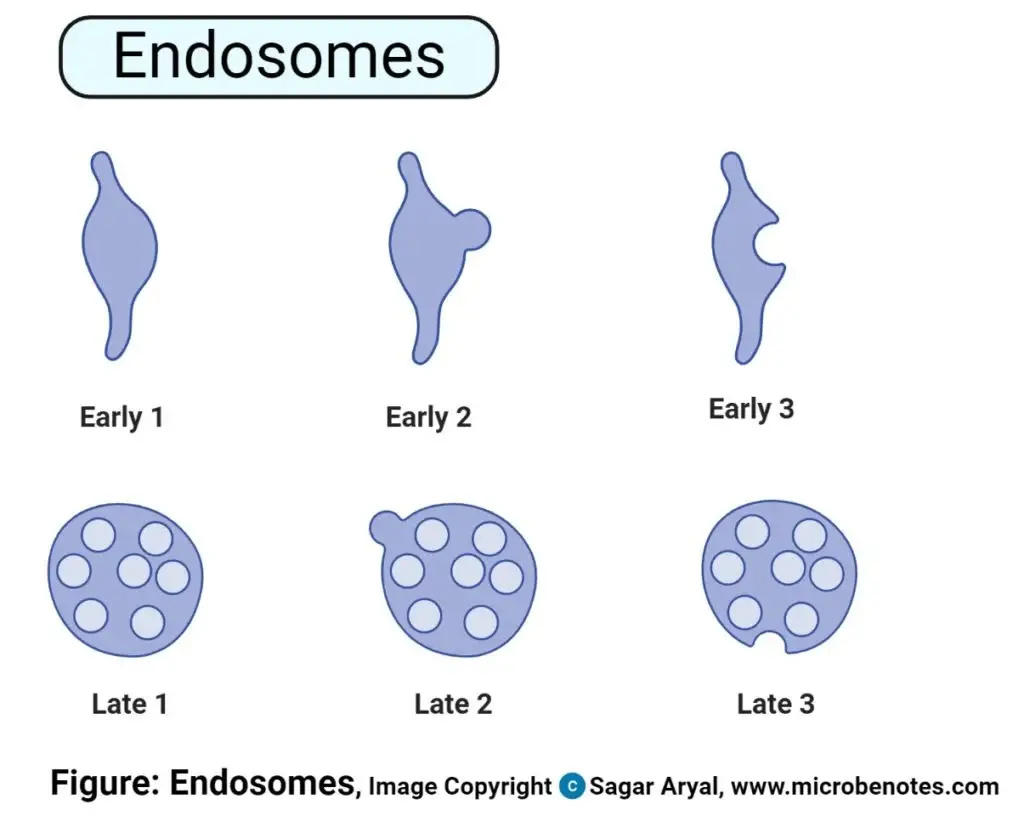
Functions of Endosomes
- Organizes and guides internalized molecules toward certain targets.
- Early endosomes send cargo to degradative routes or recycling and act as sorting stations.
- Oversees ligand and membrane receptor recycling.
- Dissociated receptors are brought back to the plasma membrane to control receptor density.
- promotes macromolecules’ breakdown.
- Late endosomes breakdown absorbed proteins, lipids, and other macromolecules by fusing with lysosomes.
- controls expression of transmembranesle receptors.
- Reversals of receptor recycling and degradation help to sustain proper cell surface levels.
- Provides signaling platforms.
- Activating receptors and signaling complexes, hosts let cells know about stress reactions and nutritional state.
- allows retrograde movement to the Golgi system.
- Choose goods to be transported back to the trans Golgi network for processing and reusing.
- Combines secretory and endocytic channels.
- Bridges move from lysosomes, Golgi complex, plasma membrane,
- helps digest antigens for immunological responses.
- Antigens in endosomal compartments precede loading onto MHC class II molecules.
- preserves vacuolar activities in plant cells.
- controls vacuumuole generation, maintenance, and expansion for cell development.
- temporarily locks vesicles for freight sorting
- acts as temporary vesicular compartments before target delivery.
- helps extracellular compounds to be absorbed via endocytic routes.
- incorporates drugs into endosomal vesicles for intracellular movement and processing.
- Promotes endosomal maturation and clathrin mediated endocytosis.
- To start cargo sorting and maturation into late endosomes, uncoated vesicles fuse with early endosomes.
- supports cell dynamics, control of growth, and defense.
- Coordinating endocytic events influences cell motility, nutrition absorption, and immunological protection.
10. Golgi Apparatus/ Golgi Complex/ Golgi Body
The Golgi Apparatus is the main organelle in cells of eukaryotrd that helps in the packing of macromolecules into vesicles that can be transported out to the site of action.
Golgi Apparatus is also known by its name Golgi Complex. This is an elastomeric organelle made up of a set of pouches flattened, stacked and known as Cisternae. The cell organelle is principally involved in transporting, changing and packaging proteins and lipids to specific locations. Golgi Apparatus is located within the cell’s cytoplasm and is present in animal and plant cells.
Structure of Golgi Apparatus/ Golgi Complex/ Golgi Body
- Usually stacked four to eight cisternae per stack in animal cells, Golgi apparatus made of flattened membrane‑bound sacs termed cisternae is supported by several related vesicles.
- With a convex cis face facing the endoplasmic reticulum and a concave trans face pointed toward the plasma membrane, Golgi body shows clear polarity.
- On the cis face, a network of tubules and vesicles called cis Golgi network absorbs freshly produced proteins from the ER via COPII‑coated vesicles.
- The entrance compartments where first glycosylation modifications and protein folding quality control take place are cis cisternae.
- Enzymes found in medial cisternae help to further process and modify proteins, including sugar trimming and adding processes.
- The exit compartments where last protein changes take place and cargo is arranged for future movement are trans cisternae.
- Adjacent to the trans face, a tubular-vesicular network formed by trans Golgi network sorts proteins and lipids into vesicles targeted to either lysosomes, secretory granules or plasma membrane.
- Associated transport vesicles include clathrin‑coated vesicles guiding cargo toward endosomes and the plasma membrane and COPI‑coated vesicles controlling retrograde trafficking to early Golgi compartments.
- Among eukaryotes, Golgi shape ranges from an interconnected ribbon of stacks in mammalian cells to isolated ministacks in plant cells to unstacked cisternae in yeast.
- Anchoring the Golgi close to the centrosome, cytoskeletal microtubules preserve its juxtanuclear localization and facilitate directed vesicle trafficking.
- Golgi matrix proteins include golgins and GRASP proteins create a structural framework preserving general Golgi integrity and cisternial stacking.
- Dynamic existent in forms of cisternae, tubules, and vesicles that remodel during cell division and cargo processing, Golgi structure is pleomorphic.
- Contributing to Golgi formation and maintenance of stack number, vesicular clusters bud off the ER and merge to generate new cisternae.
- Golgi stack quantity, size, and organization match cellular secretory activity; highly secretory cells have clearly visible Golgi structures.
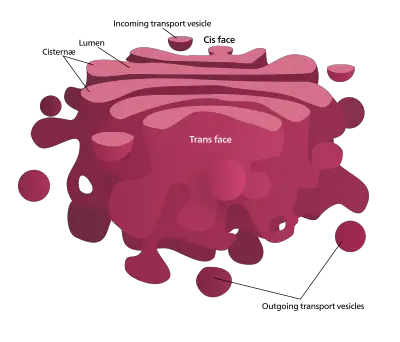
Functions of Golgi Apparatus/ Golgi Complex/ Golgi Body
- Protein and lipid sorting and packing into vesicles – Gets goods from endoplasmic reticulum at the cis face and guides vesicles into lysosomes, secretory granules, or the plasma membrane.
- Post-translational changes of proteins including sulfation and glycosylation – Ciszenae trim Medial and trans cisternae as well as n‑linked oligosaccharides add sulfate groups and complex sugars.
- Lipid metabolism and glycolipid production include sphingolipids – lysosome formation and trafficking tagged hydrolases with mannose‑6‑phosphate
- Specialized secretory cell mediation of the secretory pathway – Sort and pack insulin in pancreatic beta cells, digestive enzymes in acinar cells, extracellular matrix components in fibroblasts.
- Reversing transport and receptor recycling using COPI-coated vesicles – Returns membrane receptors back to previous Golgi cisternae or the endoplasmic reticulum escaped ER-resident proteins.
- Integration of endocytic and secretory processes functioning as a central trafficking center
- Platform for signaling controlling cellular stress reactions and innate immunity.
- In plant cells, synthesis of cell wall polysaccharides and pectins
- Protein folding and retention quality control for destruction of misfolded proteins
- Golgi structure and morphology maintained by golgins and GRASP scaffold proteins
11. Intermediate filaments
The third filament class that makes up the cytoskeleton is the intermediate filaments. They are classified as intermediate filaments due to the diameter intermediate of the filaments compared to myosin and microfilaments.
Structure of Intermediate filaments
- About 10 nm in average diameter, intermediate filaments are rope-like cytoskeletal fibers between actin microfilaments (7 nm) and microtubules (25 nm).
- Comprising a varied family of related fibrous proteins categorized into six categories (I–VI), cytoplasmic keratins, vimentin, desmin and nuclear lamins
- Every monomer consists of a core α‑helical “rod” domain flanked by non‑helical N‑terminal “head” and C‑terminal “tail”.
- Monomers couple in parallel to create a coiled-coil dimer.
- Two rod domains form a stable coiled coil by hydrophobic contacts wrapping around each other.
- Dimers staggered in a staggered pattern generate non-polar tetramers by aligning antiparallel.
- Eight tetramers laterally combine to produce a unit-length filament, which anneals end-to- end producing lengthy protofilsaments.
- Tensile strength is conferred by a flexible, non-polar rope of protofilaments called mature intermediate filament without clear “plus” or “minus” ends.
- Anchoring to cell–cell junctions and organelles, filaments create vast cytoplasmic networks called the nuclear lamina.
- Expression tailored to a tissue results in cell type diversity:
- Keratins in cells of epithelial nature
- Neurofilament in neurons
- Desmin in muscular cells.
- Dynamic assembly/disassembly under control by phosphorylation:
- Filament disintegration in mitosis results from phosphorylation.
- Reassembly is promoted by dephosphorylation following mitosis.
- Plectin and other associated crosslinking proteins bind intermediate filaments to microtubules, actin and membrane complexes.
- Though their chemical makeup is less clear in plant cells, IF‑like proteins create networks supporting cell shape and mechanical stability.
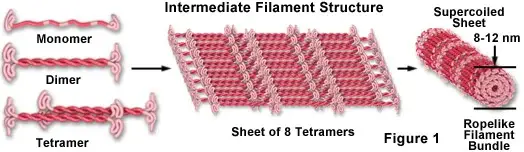
Functions of Intermediate filaments
- Provide structural support and mechanical stability to cells
- Bear tensile stress to maintain cell shape and prevent rupture under strain
- Anchor the nucleus and other organelles in their proper intracellular locations
- Link to cell–cell and cell–matrix junctions to maintain tissue integrity
- Serve as flexible intracellular tendons absorbing mechanical forces during deformation
- Facilitate cell motility by interacting with signaling molecules and pathways
- Provide a scaffold for organelle positioning and intracellular transport
- Form the nuclear lamina to support nuclear envelope structure and regulate gene expression
- Resist compressive forces in conjunction with microtubules and actin filaments
- Assemble into durable, long‑lived filaments that persist through dynamic cell processes
12. Lysozyme
Lysozymes, also known as lysosomes, are membrane-bound organelles found in the cytoplasm of animal cells. They play a crucial role in intracellular digestion and the breakdown of various macromolecules. Lysozymes contain a variety of hydrolytic enzymes, including lipases, amylases, proteases, and nucleases, which are involved in the degradation of lipids, carbohydrates, proteins, and nucleic acids, respectively.
Lysozymes are spherical vesicles enclosed by a single membrane called the lysosomal membrane. This membrane helps maintain the acidic pH inside the lysosome, which is necessary for the optimal activity of the hydrolytic enzymes. The lysosomal membrane also acts as a barrier, preventing the enzymes from leaking into the cytoplasm and ensuring their confined action within the lysosome.
Within the cell, there are no distinct types of lysozymes referred to as primary and secondary lysozymes. However, it is important to note that lysosomes can fuse with other vesicles or organelles, allowing the enzymes contained within the lysosome to degrade the engulfed materials. This fusion process helps in the breakdown and recycling of various cellular components, including damaged organelles and engulfed molecules or organelles.
The primary function of lysozymes is intracellular digestion and the recycling of cellular waste. They are involved in the degradation of worn-out organelles, the turnover of cellular components, and the clearance of foreign materials, such as bacteria or cellular debris. Lysozymes also play a role in various cellular processes, including autophagy, where they participate in the degradation of unwanted cellular components.
In summary, lysozymes are membrane-bound organelles found in animal cells. They contain hydrolytic enzymes involved in the degradation of macromolecules. Lysozymes function in intracellular digestion, the recycling of cellular waste, and the clearance of foreign materials.
- Primary lysosome, which contains hydrolytic enzymes such as lipases, proteases, amylases, and nucleases.
- Secondary lysozyme created by the combination of primary lysozymes that contain organelles or molecules that have been engulfed.
Structure of Lysozyme
- Lysozyme is a tiny globular enzyme made of one polypeptide chain of 129 amino acids joined end to end starting with a lysine residue.
- While around twelve percent of the lysozyme’s residues form beta sheet structures, forty percent adopt alpha helical conformations.
- Lysozyme’s secondary structure consists of five antiparallel beta strands linked by beta turns and random coil regions, four alpha helices including one 3₁₀ helix.
- Four disulphide bonds tying residues 6 and 127, 30 and 115, 64 and 80, and 76 and 94 stabilise lysozyme’s secondary and tertiary structure.
- Lysozyme has two domains: a C terminal domain formed of a bundle of alpha helices joined by a long central helix and a N terminal domain built of antiparallel beta sheets.
- An active region where catalytic residues glutamate 35 and aspartate 52 bind and hydroly the substrate produces a clear gap between the two domains.
- Lysozyme uses a compact globular design with hydrophobic amino acid side chains buried in the core and hydrophilic side chains orienting themselves towards the solvent interface.
- With a molecular mass of around fourteen thousand three hundred daltons, solution hen egg white lysozyme functions as a monomer rather than assembling into higher order complexes.
- Two domains linked by a hinge region enable domain motions for substrate access, thereby maintaining a single polypeptide chain in function even in bacteriophage T4 lysozyme.
Functions of Lysozyme
- Catalyzes hydrolysis of β(1→4) glycosidic bonds in bacterial peptidoglycan, leading to cell lysis
- Provides innate immune defense at mucosal surfaces (tears, saliva, mucus, breast milk) by destroying bacteria
- Released from neutrophil and macrophage granules to enhance phagocytic killing of pathogens
- Generates peptidoglycan fragments that activate host pattern recognition receptors, modulating immune response
- Disrupts and prevents bacterial biofilm formation, supporting wound‑healing applications
- Hydrolyzes chitin‑like substrates in fungal cell walls, contributing to antifungal defense
- Stimulates secretion of immunoglobulin A in the gut, strengthening mucosal immunity
- Facilitates digestion in herbivorous animals by breaking down bacterial cell walls in the gastrointestinal tract
- Binds bacterial surface carbohydrates through lectin‑like interactions, exerting non‑enzymatic bactericidal effects
13. Microfilaments
Microfilaments form part of the cytoskeleton in cells, made of actin proteins as parallel polymers. They are the smallest filaments in the cytoskeleton that have the highest rigidity and flexibility giving strength and flexibility to the cells.
Structure of Microfilaments
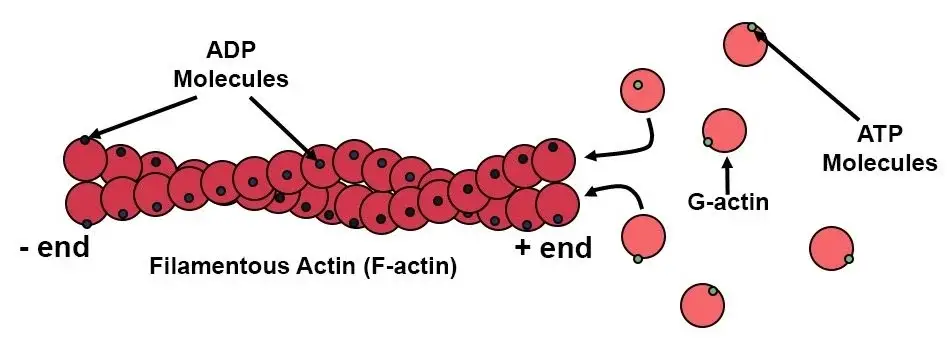
Functions of Microfilaments
- Microfilaments are polymers of globular actin proteins assembled head to tail into filamentous actin made of two interwoven helical threads.
- Each actin filament is a right handed double helix with a diameter of around seven nanometres and a helical repeat of roughly thirty seven nanometres equivalent to roughly thirteen actin subunits each crossing.
- Microfilaments have structural polarity with a barbed plus end that elongates ten times quicker than the pointed minus end.
- Microb Notes
- ATP binding and hydrolysis drive actin polymerisation by means of dynamic assembly and disassembly producing treadmilling behaviour at constant state.
- Formin family proteins and the Arp2/3 complex help cells to enhance the production of an actin trimer, which is the rate limiting nucleation phase of filament building.
- Alpha actinin filamin and fascin monomer sequestersing proteins are among the many actin binding proteins that control microfilament shape and organisation. Thymosin beta four barbed end capping proteins; profilin CapZ and CapG cutting proteins cofilin and gelsolin
- Tropomyosin stabilises F actin and controls access of other binding proteins like myosin in muscle and nonmuscle cells by binding along the long pitch of actin filaments encompassing seven successive subunits.
- Tropomyosin in muscle cells works with troponin to regulate myosin binding to actin, therefore permitting calcium dependent contraction control.
- Microfilaments arrange either branching networks as seen in lamellipodia and the cell cortex or parallel bundles as shown in filopodia stress fibres.
- Concentrated in the cell periphery, microfilament form a cortical network necessary for control of mechanical stability and cell shape mobility.
- In both muscle contraction and intracellular movement, myosin motor proteins find paths in actin filaments.
- Microfilament’s flexible yet strong construction helps cells to resist compressive and tensile stresses while fast remodelling to enable activities such amoeboid motility and cytokinesis endocytosis.
14. Microtubules
Microtubules also form a part of the cytoskeleton, which differs from microfilaments because of that they contain tubulin.
Structure of Microtubules
- Long hollow cylinders made of α‑ and β‑tubulin heterodimers, microtubules polymerise head‑to‑ tail into linear protofilaments.
- Usually aligning in parallel and laterally, thirteen protofilaments form the tubular wall of a microtubule with an inner lumen diameter roughly 15 nanometres and an outside diameter of around 25 nanometres by means of lateral interactions.
- Comprising two globular subunits α‑tubulin and β‑tubulin each with a molecular weight of around 50.0 Da and an isoelectric point between 5.2 and 5.8, each tubulin heterodimer is approximately 8.0 nanometres long.
- Microtubules have intrinsic structural polarity; a fast-growing plus end exposes β‑tubulin and a slower-growing minus end exposes α‑tubulin, which controls directional polymerisation dynamics.
- Heterotypic α‑β interactions violate the pseudo‑helical symmetry to produce a discontinuity in the B‑lattice that affects microtubule dynamics and interactions with microtubule‑associated proteins.
- γ‑tubulin ring complexes at microtubule‑organizing centres such centrosomes in mammalian cells or spindle pole bodies in fungus where the 13‑protofilament architecture stimulates microtubule nucleation.
- Lowering the kinetic barrier for nucleation, γ‑Tubulin ring complexes generate a template matching the symmetry of 13 protofilaments.
- Microtubule-associated proteins bind either along protofilaments or at ends to control stability and organisation.
- Structural MAPs include tau MAP2 and MAP4 bind along the microtubule surface to stabilise the lattice and regulate filament spacing.
- Motors proteins Kinesin and dynein carry cargo by walking along microtubules towards plus or minus ends respectively.
- AAA‑ATPase proteins, like katanin spastin and fidgetin, cleave microtubules into shorter filaments to reorganise networks.
- In motile cilia and flagella microtubules are organised in a 9+2 pattern with nine outer doublet microtubules (each including a full A‑tubule of 13 protofilaments and an incomplete B‑tubule of 10 protofilaments) surrounding two core singlet microtubules.
- Each triplet in the 9‑triplet microtubule cylinder of centrioles and basal bodies consists of one whole A‑tubule and two partial B and C tubules organised with a cartwheel scaffold at the core.
- Five‑protofilament “mini‑microtubules” made from some bacterial tubulin homologues BtubA/B show evolutionary modifications of the tubulin fold.
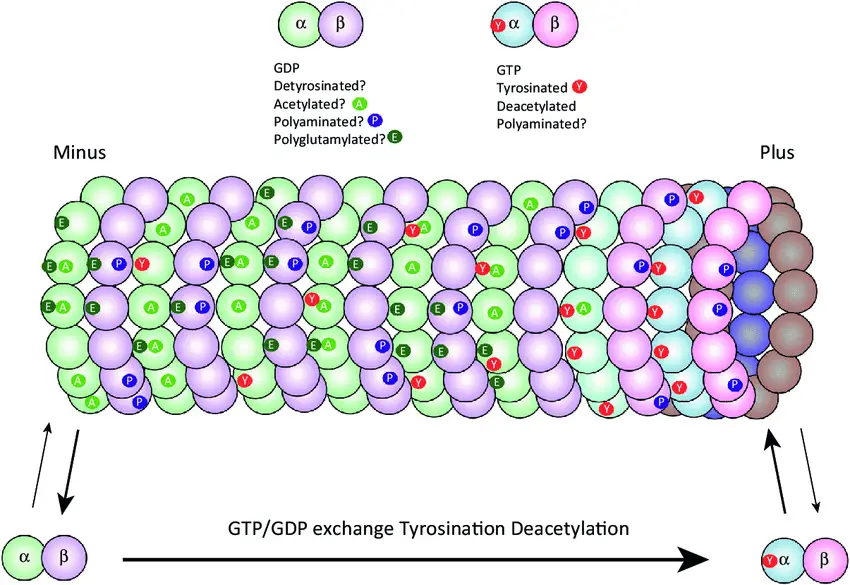
Functions of Microtubules
- Maintenance of cell form via stiff hollow rods resisting compression and defining cell architecture.
- Generation of intracellular transport highways acting as tracks for motor proteins kinesin and dynein to move organelles vesicles and macromolecules.
- Whereas dynein causes retrograde movement towards the minus ends, kinesin controls anterograde movement towards the plus ends.
- Development of the mitotic and meiotic spindle fibres causing precise chromosomal alignment and segregation during cell division
- Organisation and anchoring of organelles emanating from microtubule organising centres including centrosomes or basal bodies.
- building and running cilia and flagella systems that support extracellular fluid flow and cell motility.
- Facilitation of cytoplasmic streaming (cyclosis) to guarantee homogeneous distribution of nutrients and organelles inside big cells
- Participating in morphogenetic processes via regulating cell elongation and differentiation throughout developmental events
- Orienting microtubule plus ends towards the leading edge helps to regulate cell polarity and directed migration, hence guiding vescular trafficking and focal adhesion turnover.
- Cytoskeletal signalling pathways affected by microtubule dynamics and interactions with transcription factors modulates gene expression.
- Assembly of centrioles and basal bodies essential for spindle pole development and ciliogenesis commencement
- Organisation and maintenance of Golgi apparatus placement by nucleating Golgi derived microtubules and guarantees correct secretory pathway activity.
15. Microvilli
Microvilli are tiny structures resembling fingers that extend from or onto cells. They can be found on their alone or when they are in combination with villi.
Structure of Microvilli
- Mostly in the small intestine and kidney tubules, microvilli are minute, finger-like projections extending from the apical surface of epithelial cells, which greatly improve the cell’s absorptive capacity by increasing surface area.
- Comprising a core of 20–30 tightly packed actin filaments, which give structural support, each microvillus is surrounded by the plasma membrane. Bundling proteins like fimbrin (plastin-1), villin, and espin links these filaments such that the microvillus remains erect and firm.
- Often capped by proteins like capZ, the actin filaments of the microvillus are fixed at their plus ends near the tip and their minus ends stretch into the terminal web, a dense network of cytoskeletal components underneath the apical membrane. The terminal web consists of contractile proteins such myosin II and tropomyosin, which help microvilli to be dynamically spaced and organised, and actin filaments stabilised by spectrin.
- Myosin-1a and calmodulin not only stabilise the microvillus structure but also help to mediate lateral connections between the actin core and the plasma membrane, hence maintaining microvillar length and playing roles in membrane trafficking.
- Glycoproteins and glycolipids form a carbohydrate-rich layer called a glycocalyx that covers the outside surface of microvilli. Crucially for nutrition digestion at the epithelium surface, this layer protects, moderates cell-cell interactions, and stores digestive enzymes like disaccharidases and peptidases.
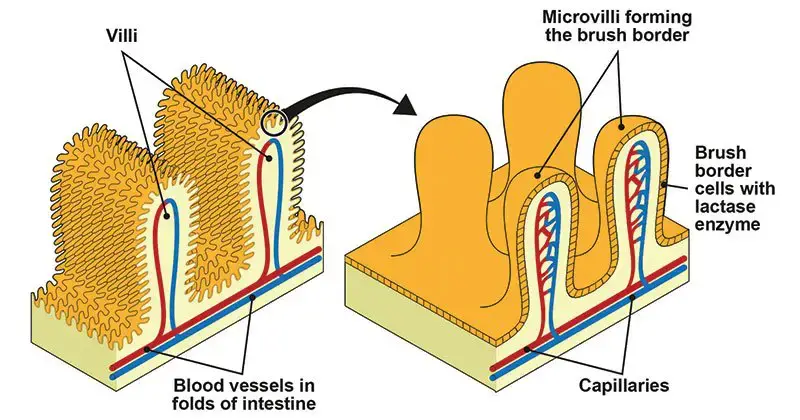
Functions of Microvilli
- Microscopic, finger-like projections called microvilli on the apical surface of epithelial cells greatly expand the surface area of the cell, therefore improving its functional capacity.
- Microvilli create the brush boundary of the small intestine, therefore augmenting the surface area for nutrition absorption. This structural modification helps nutrients—including amino acids, monosaccharides, and lipids—to be effectively absorbed.
- Essential for the latter stages of carbohydrate digestion, digestive enzymes like sucrase-isomaltase and lactase abound in the plasma membrane of microvilli.
- Microvilli help to mix luminal contents and improve interaction between digesting enzymes and substrates, therefore contributing to the motility of the brush border.
- Microvilli in the renal proximal tubules greatly help to maintain fluid and electrolyte balance by increasing the surface area available for the reabsorption of water, ions, and small molecules.
- By raising the apical surface area, microvilli help substances to be secreted, therefore improving the capacity of the cell to release enzymes and other molecules into the lumen.
- Stereocilia, specialised microvilli found in the inner ear, are involved in mechanotransduction—that is, they transform mechanical inputs into electrical impulses needed for balance and hearing.
- On immune cells, including lymphocytes, microvilli help in cell adherence and movement, therefore enabling immune surveillance and response. ,
- Microvilli are important in oocytes as they help sperm to adhere and fuse during fertilisation, hence facilitating reproduction.
- Motile behaviour of microvilli helps to eliminate undesired materials from the cell surface and supports the preservation of cellular homeostasis.
16. Mitochondria
Mitochondria are cells with two membranes that are responsible for the production as well as storage of fuel to the cell. The oxidation process of different substances in cells and the release of energy as a form ATP (Adenosine Triphosphate) is the main purpose of mitochondria.
Structure of Mitochondria
- Found in most eukaryotic cells’ cytoplasm, mitochondria are double-membrane-bound organelles ranging in size from 0.5 to 1.0 micrometres.
- Comprising porin proteins that provide smoothness and permeability to tiny molecules and ions, the outer mitochondrial membrane is
- Highly impermeable and greatly folded into structures known as cristae, the inner mitochondrial membrane expands the surface area for metabolic processes.
- The electron transport chain and the creation of the proton gradient required for ATP production depend critically on the intermembrane gap, which lies between the outer and inner membranes.
- Enzymes of the Krebs cycle, mitochondrial DNA (mtDNA), ribosomes, and other elements necessary for mitochondrial operation are found in the mitochondrial matrix, which is contained by the inner membrane. ,
- Supporting the endosymbiotic idea of their origin, mitochondria include their own circular DNA, which codes for some of the proteins needed for mitochondrial operation.
- Further underlining their semi-autonomous character, ribosomes found inside mitochondria allow the organelle to synthesis certain proteins autonomously.
- With the cristae of the inner membrane allowing a platform for the electron transport chain and ATP synthesis, the structural arrangement of mitochondria is closely related to their function in energy generation.
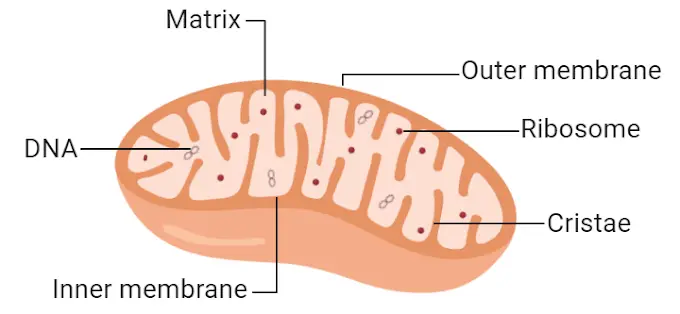
Functions of Mitochondria
- Mitochondria are double-membraned organelles present in the majority of eukaryotic cells, sometimes termed the “powerhouses of the cell” because of their function in energy generation.
- The principal role of mitochondria is to produce adenosine triphosphate (ATP) via oxidative phosphorylation, a mechanism that takes place in the inner mitochondrial membrane. This encompasses the electron transport chain and ATP synthase, which collectively generate the bulk of the cell’s ATP.
- Mitochondria are essential in modulating apoptosis, or programmed cell death, by releasing cytochrome c and other pro-apoptotic substances into the cytoplasm, which activates caspases that result in cell death.
- They participate in calcium homeostasis by buffering and storing calcium ions, which are crucial for several physiological activities, including muscle contraction and signal transmission.
- Mitochondria facilitate thermogenesis, particularly in brown adipose tissue, by producing heat via proton leak across the inner mitochondrial membrane, regulated by uncoupling proteins.
- They participate in the metabolism of amino acids and lipids, encompassing the β-oxidation of fatty acids, which produces acetyl-CoA for the citric acid cycle.
- Mitochondria possess their own circular DNA (mtDNA), which encodes vital components of the respiratory chain. This enables them to synthesise some proteins independently and bolsters the endosymbiotic notion of their genesis.
- They participate in the manufacture of certain hormones, including steroid hormones, by supplying the requisite enzymes and conditions for steroidogenesis.
- Mitochondria are involved in regulating the cell cycle and growth by engaging with signalling pathways that govern cell proliferation and differentiation.
- They contribute to innate immunity by generating reactive oxygen species (ROS) and additional signalling molecules that initiate immune responses against pathogens.
17. Nucleus
Nucleus is a dual membrane-bound structure that is responsible for controlling every cell’s activity and is also a central point for genetic materials and also for transferring. It is among the cell organelles that are large, taking up 10% of the total cell space. It is commonly referred to as”the “brain of the cell” because it is the one that gives commands to the functioning of other organelles within the cell. The term “nucleus” is defined when it comes to cells that are eukaryotic, but it is not present in prokaryotic cells that have the genetic material that is distributed within the cytoplasm.
Structure of Nucleus
- Found in eukaryotic cells, the membrane-bound organelle known as the nucleus coordinates cellular functions including growth, metabolism, and reproduction and stores genetic information.
- Comprising a double-membrane construction, the nuclear envelope encloses the nucleus and divides its contents from the cytoplasm. Whereas the inner membrane is bordered by the nuclear lamina, a thick fibrillar network that gives structural support, the outer membrane is continuous with the endoplasmic reticulum and is studded with ribosomes. By means of their perforation of the envelope, nuclear pores control the bidirectional molecular flow between the nucleus and cytoplasm.
- Enclosed by the nuclear membrane, the viscous fluid known as nucleoplasm—also called nuclear sap—is It is used in the transportation of components required for nuclear metabolism and gene expression as well as for the suspension of nuclear elements.
- Found within the nucleoplasm, chromatin is a combination of DNA and histone proteins. It appears in two forms: heterochromatin, which is more condensed and transcriptionally inactive; euchromatin, which is less condensed and transcriptionally active. Stability of the genome and control of genes depend critically on the arrangement of chromatine.
- Not encircled by a membrane, the dense, spherical structure known as the nucleolus forms within the nucleus. Synthetic ribosomal RNA (rRNA) is produced here, and first assembly of ribosomal subunits takes place here before being moved to the cytoplasm for ultimate assembly into functioning ribosomes.
- A fibrous network, the nuclear matrix supports the nucleus structurally and could help to organise chromatin and control gene expression.
- Comprising intermediate filament proteins known as lamins, the nuclear lamina lines the inside surface of the nuclear envelope. It participates in fundamental activities like DNA replication, transcription, and chromatin organisation and offers mechanical support.
- Important subnuclear structures involved in the processing and assembly of RNA and ribonucleoprotein particles abound in the nucleus as well: Cajal bodies, PML bodies, and nuclear speckles.
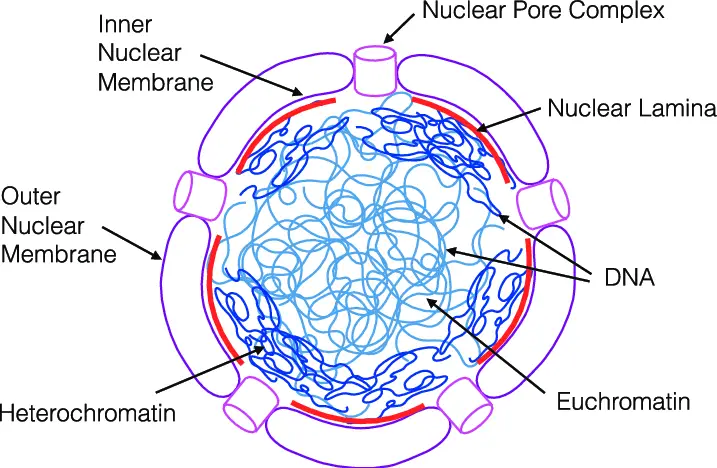
Functions of Nucleus
- In eukaryotic cells, the nucleus is a membrane-bound organelle whose housing of the cell’s genetic material coordinates gene expression and replication processes acts as the control centre for cellular activity.
- Essential for the passage of genetic information during cell division, it preserves the hereditary material of the cell in DNA form arranged into chromatin.
- By directing the transcription of DNA into messenger RNA (mRNA), the nucleus controls gene expression and thereby determines which proteins the cell synthesises.
- During the S phase of the cell cycle, it controls DNA replication such that daughter cells have precise duplication of genetic material.
- To generate mature mRNA molecules suitable for translation, the nucleus helps pre-mRNA be processed in capping, polyadenylation, and splicing.
- It helps the ribosomal subunits in the nucleolus to assemble and subsequently be exported to the cytoplasm for protein synthesis. ω
- By separating DNA away from cytoplasmic events, the nucleus preserves the integrity of genetic information and guards it from harm.
- It is involved in the control of genes linked to cellular metabolism and growth, therefore regulating these processes.
- Through control of gene expression unique to many cell types, the nucleus helps to define cellular differentiation.
- It modulates gene expression in response to different stimuli, therefore influencing the response of the cell to stress and outside signals.
18. Peroxisomes
Peroxisomes are membrane-bound organelles that undergo oxidation that are found in the cell cytoplasm of all Eukaryotes. Their name is attributed to their hydrogen peroxide-generating and eliminating activities.
Structure of Peroxisomes
- Usually measuring 0.1 to 1.5 micrometres in diameter, peroxisomes are membrane-bound organelles present in eukaryotic cells’ cytoplasm.
- Their single phospholipid bilayer membrane, which has many membrane-bound proteins, encloses them.
- Granular interior matrix of peroxisomes comprises of oxidative reaction-related enzymes.
- Because peroxisomes lack their own DNA and ribosomes, all proteins are produced in the cytoplasm and imported into the peroxisome.
- They include catalase, which breaks down hydrogen peroxide into water and oxygen, therefore shielding the cell from oxidative harm.
- To coordinate metabolic activity, peroxisomes interact with other organelles like mitochondria and the endoplasmic reticulum.
- They help to synthesise plasmalogens, which are crucial for the regular operation of lungs and mammalian brains.
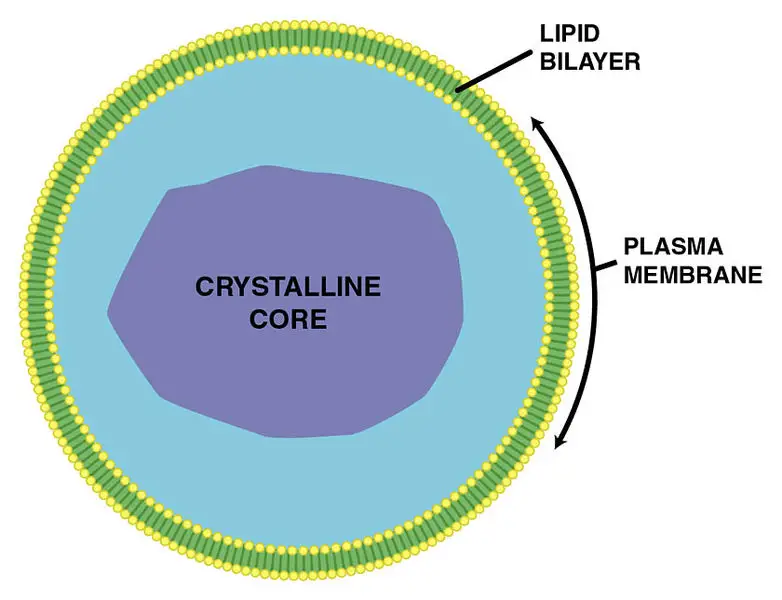
Functions of Peroxisomes
- Peroxisomes help β-oxidation of extremely long-chain fatty acids, therefore transforming them into shorter chains ready for further breakdown in mitochondria. Particularly in organs like the kidney and liver, this mechanism is absolutely vital for energy generation.
- Catalase, among other enzymes found in peroxisomes, breaks down hydrogen peroxide (H₂O₂), a reactive oxygen species, into water and oxygen, therefore shielding the cell from oxidative damage.
- Peroxisomes help to biosynthesise plasmalogens, ether phospholipids necessary for the correct operation of mammalian lungs and brains.
- In the liver, peroxisomes help to manufacture bile acids from cholesterol, which are essential for the breakdown and absorption of dietary lipids. ”
- Detoxification of many drugs is accomplished by peroxisomes. In liver cells, for example, they aid in the breakdown of poisons including alcohol.
- Peroxisomes help in photorespiration, a process in which carbon from phosphoglycolate is recycled during photosynthesis, therefore preserving energy and carbon in plant cells.
- In sprouting seeds, peroxisomes—also known as glyoxysomes—help to convert fatty acids to carbohydrates, therefore supplying the energy the developing plant needs.
- In certain yeasts, peroxisomes are engaged in the oxidation and absorption of methanol and amines, activities fundamental for cellular metabolism.
- Urate oxidase and D-amino acid oxidase are two of the many enzymes found in peroxisomes that help in oxidation events generating hydrogen peroxide.
- Reactive oxygen species generated by peroxisomes—especially hydrogen peroxide—have functions in cellular signalling pathways, therefore affecting processes like gene expression and cell development.
- Instead of having their own DNA, peroxisomes import cytoplasmic synthesised proteins. They split to create new peroxisomes and expand by include proteins and lipids.
19. Plasmodesmata
Plasmodesmata are tiny channels or passages which allow for the transfer of materials and communications between cells.
Structure of Plasmodesmata
- Plasmodesmata are microscopic channels that traverse the cell walls of plant cells
- They facilitate communication and transport between adjacent plant cells
- Each plasmodesma is lined with the plasma membrane, continuous between connected cells
- The central structure within a plasmodesma is the desmotubule, a modified strand of endoplasmic reticulum (ER)
- The desmotubule connects the ER of neighboring cells
- Surrounded by cytoplasmic sleeve, a space allowing molecule movement
- Cytoplasmic sleeve contains proteins and cytoskeletal elements
- Actin filaments and myosin proteins may assist in intracellular transport
- Spoke-like structures connect the desmotubule to the plasma membrane, regulating channel size
- Cell wall surrounding plasmodesmata contains additional carbohydrates like callose, which modulates permeability
- Plasmodesmata allow passive and active transport of water, ions, proteins, RNA, and signaling molecules
- Two types of plasmodesmata exist
- Primary plasmodesmata form during cell division during cell plate formation
- Secondary plasmodesmata develop in mature cell walls to connect cells post-division
- Size exclusion limit (SEL) determines the maximum size of molecules that can pass through
- SEL can be dynamically regulated in response to developmental or environmental cues
- Plasmodesmata often cluster in pit fields, regions of the cell wall with numerous channels
- Their structure and function differ from animal gap junctions, which lack ER components
- Plasmodesmata play critical roles in plant development, defense responses, and systemic signaling
- Viral movement proteins exploit plasmodesmata to spread infections between plant cells by dilating the channels
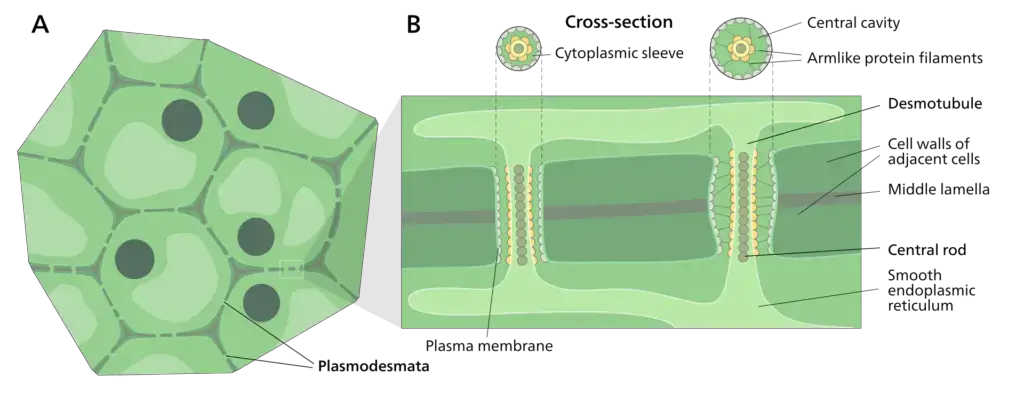
Functions of Plasmodesmata
- Plasmodesmata enable intercellular transport of water, ions, and small metabolites
- Facilitate cell-to-cell communication by allowing movement of signaling molecules like hormones (e.g., auxin) and transcription factors
- Transport RNA molecules between cells, including mRNA and siRNA, for genetic regulation and defense
- siRNA movement helps in systemic gene silencing during viral infections
- Mediate symplastic transport, creating a continuous cytoplasmic network across plant tissues
- Critical for distributing nutrients and photosynthetic products (e.g., sugars) from source to sink tissues
- Support plant development by coordinating cellular activities during growth and differentiation
- Enable synchronized tissue formation in roots, leaves, and floral organs
- Play a role in systemic acquired resistance (SAR) by transmitting defense signals to distant cells during pathogen attacks
- Allow electrical signaling through ion flux, aiding rapid responses to environmental stimuli (e.g., herbivory)
- Regulate molecular trafficking via dynamic changes in size exclusion limit (SEL)
- Callose deposition at plasmodesmata reduces permeability under stress or during SEL adjustment
- Serve as pathways for viral spread, as pathogens use movement proteins to dilate plasmodesmata and infect neighboring cells
- Maintain symplastic continuity in tissues, ensuring functional integration of cells in organs like phloem
- Differ from animal gap junctions by transporting larger molecules and including ER-derived components (desmotubule)
- Contribute to wound response by enabling resource redistribution and signaling for tissue repair
20. Plastids
Plastids are membrane-bound double structures found in plants as well as other eukaryotes involved with the production and storage of nutrients. Based on the kind of pigments the plastids come in three kinds:
- Chloroplasts – Chloroplasts are organelles with two membranes that typically vary in their shape – ranging from disc-like shapes to discoid-spherical, spherical oval, and ribbon. They are found in mesophyll cells found in leaves, where they contain chloroplasts as well as the other pigments of carotenoid. They help in capturing sunlight energy to enable photosynthesis. The membrane’s interior is enclosed by the stroma, a space. Flattened disc-like chlorophyll-containing structures known as thylakoids are arranged in a stacked manner like a pile of coins. Each pile is known as the granum (plural: the word grana) as well as the thylakoids from various grana are linked by membranous tubules that are flat, referred to as lamella stromal. Similar to mitochondrial matrix, the chloroplast’s stroma also has the double-stranded circular DNA 70S ribosomes and enzymes that are require the synthesis of proteins and carbohydrates.
- Chromoplasts – The chromoplasts contain carotenoid pigments, fat-soluble like carotene, xanthophylls, etc. that provide plants with their unique color, namely red, yellow, orange, etc.
- Leucoplasts – Leucoplasts are plastids that have no color and store nutrients. Amyloplasts are able to store carbohydrate (like the starch found in potatoes) and aleuroplasts store proteins and elaioplasts store fats and oils.
Structure of Plastids
- Plastids are double-membrane-bound organelles found in plant cells and certain protists
- The outer and inner membranes form the envelope, regulating molecule transport
- Inner membrane folds into lamellae or thylakoid structures in chloroplasts
- Stroma, the fluid matrix inside plastids, contains enzymes, DNA, ribosomes, and metabolites
- Site of the Calvin cycle in chloroplasts and starch synthesis in amyloplasts
- Chloroplasts contain thylakoids stacked into grana
- Thylakoid membranes house chlorophyll and proteins for light-dependent reactions
- Chromoplasts lack chlorophyll but contain carotenoid pigments stored in plastoglobuli
- Plastoglobuli are lipid-protein particles for pigment and lipid storage
- Leucoplasts are non-pigmented plastids involved in nutrient storage
- Amyloplasts store starch
- Elaioplasts store lipids
- Proteinoplasts store proteins
- All plastids originate from undifferentiated proplastids in meristematic cells
- Plastid DNA (ptDNA) and ribosomes enable limited autonomous protein synthesis
- Genome is circular, similar to cyanobacterial ancestors
- Plastids can interconvert based on tissue and environmental needs
- Chloroplasts transform into chromoplasts during fruit ripening
- Leucoplasts develop into chloroplasts in illuminated potato tubers
- Inner membrane systems (e.g., thylakoids) vary depending on plastid type and function
- Plastid division involves fission machinery resembling bacterial binary fission
- Symbiotic origin via endosymbiosis of cyanobacteria in eukaryotic cells
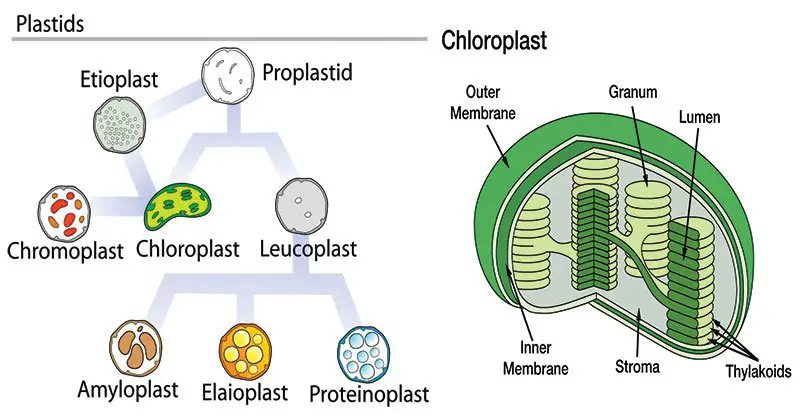
Functions of Plastids
- Plastids perform specialized functions depending on their type and cellular context
- Chloroplasts conduct photosynthesis
- Light-dependent reactions in thylakoids produce ATP and NADPH
- Calvin cycle in stroma fixes CO₂ into sugars using ATP and NADPH
- Chromoplasts synthesize and store pigments (e.g., carotenoids, anthocyanins)
- Provide color to flowers and fruits to attract pollinators and seed dispersers
- Leucoplasts store nutrients
- Amyloplasts store starch in roots and tubers
- Elaioplasts synthesize and store lipids
- Proteinoplasts accumulate proteins
- Plastids produce essential metabolites
- Synthesize fatty acids, amino acids, and terpenoids
- Generate precursors for plant hormones (e.g., gibberellins, abscisic acid)
- Chloroplasts contribute to nitrogen assimilation by converting nitrates into amino acids
- Participate in plant immune responses
- Produce reactive oxygen species (ROS) and defense-related signaling molecules
- Enable photoprotection in chloroplasts via non-photochemical quenching (NPQ) to dissipate excess light energy
- Plastids interact with mitochondria for energy and metabolite exchange
- Chloroplasts regulate stomatal opening by producing malate during light reactions
- Plastid genome (ptDNA) encodes components for photosynthesis, transcription, and translation
- Retain bacterial-like ribosomes for limited autonomous protein synthesis
- Plastids undergo interconversion to adapt to developmental or environmental changes
- Chloroplasts transform into chromoplasts during fruit ripening
- Etioplasts develop into chloroplasts upon exposure to light
- Serve as sensors for environmental stressors (e.g., light intensity, pathogens) and trigger adaptive responses
- Symbiotic origin from cyanobacteria enables evolutionary conservation of prokaryotic-like processes (e.g., fission)
21. Ribosomes
Ribosomes are ribonucleoproteins that contain equal amounts of proteins and RNA together with a variety of other components essential to synthesize proteins. In prokaryotes they can be found freely and in eukaryotes they are either found in the form of on their own or in the endoplasmic retina.
Structure of Ribosomes
- Found in all living cells, ribosomes are sophisticated molecular machinery that synthesise proteins by translating messenger RNA (mRNA) into amino acid sequences.
- Comprising ribosomal RNA (rRNA) and ribosomal proteins, they create ribonucleoprotein complexes.
- Two subunits make up ribosomes: a smaller subunit bound to mRNA and a bigger subunit in which amino acids are coupled to generate proteins.
- With bacterial ribosomes having a 70S unit made of 30S and 50S subunits and eukaryotic ribosomes having an 80S unit made of 40S and 60S, the sedimentation coefficient (S) of ribosomes differs throughout species.
- Whereas in eukaryotes they can be free or attached to the endoplasmic reticulum, creating the rough ER, in prokaryotes ribosomes are free in the cytoplasma.
- Prokaryotes and eukaryotes have different ribosome structures; eukaryotic ribosomes reflect their evolutionary difference by being bigger and more sophisticated.
- Essential for protein synthesis, ribosomes’ shape closely corresponds to their purpose in converting genetic information into useful proteins.
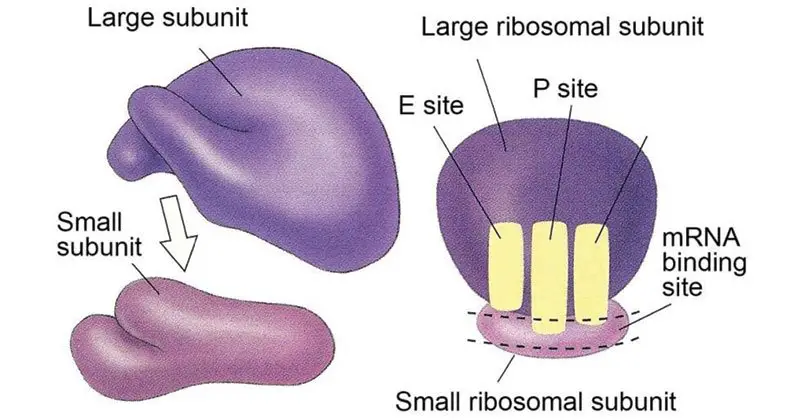
Functions of Ribosomes
- Essential molecular machinery in synthesising proteins by translating genetic information contained in Messenger RNA (mRNA), ribosomes
- Ribosomes mostly help to enable the process of translation, in which the mRNA genetic code is translated to build amino acids into polypeptide chains, therefore producing functional proteins.
- Two subunits make up ribosomes: the tiny subunit hooks itself to the mRNA and the bigger subunit catalyses the synthesis of peptide bonds between amino acids.
- Ribosomes travel along the mRNA reading its codons and organising the binding of transfer RNA (tRNA) molecules containing certain amino acids during translation.
- Producing proteins with the correct structure and function depends on the ribosome’s ability to connect amino acids in the right order.
- While in eukaryotes the cytoplasm or on the rough endoplasmic reticulum, where ribosomes are bound, in prokaryotes translation takes place in the cytoplasm.
- Polysomes, complexes whereby many ribosomes concurrently translate a single mRNA molecule, improve the efficiency of protein synthesis.
- Translation consists in three main phases: initiation, elongation, and termination, each under control by different elements to guarantee proper synthesis of proteins.
- In co-translational protein folding, ribosomes also help to properly fold newly produced polypeptides as they are synthesised.
- Insights into gene expression and the development of treatment approaches aiming at protein synthesis in disorders including cancer and bacterial infections depend on an awareness of ribosome activity.
22. Storage granules
Storage granules, which are membrane-bound organelles also known as zymogen granules that store cells’ energy reserve as well as other compounds.
Structure of Storage granules
- Specialised intracellular structures called storage granules help cells to store different compounds, including metabolic intermediates, energy reserves, and nutrients, within of cells.
- Usually tiny, membrane-bound organelles with a lipid bilayer membrane encapsulating the stored contents, these granules are made of
- The kind of cell and its metabolic demands will affect the stored compounds found in these granules.
- Storage granules in prokaryotic cells generally comprise polymers including polyphosphate, poly-β-hydroxybutyrate (PHB), or elemental sulphur, which act as substrates or energy stores for metabolic activities.
- Essential for energy metabolism and cellular homeostasis, storage granules in eukaryotic cells may store glycogen, lipids, or other metabolites.
- Tight control over the generation and use of storage granules guarantees that cells may effectively control their internal resources in reaction to metabolic demands and environmental changes.
- Storage granules’ arrangement and makeup can reveal information about the metabolic capacity and adaptive tactics of many species.
Functions of Storage granules
- Found in both prokaryotic and eukaryotic cells, storage granules are specialised intracellular structures that act as repositories for several molecules essential to cellular metabolism and survival.
- Depending on the organism and its metabolic needs, these granules—usually membrane-bound vesicles storing molecules including glycogen, polyphosphate, sulphur, and lipids—are either.
- In prokaryotic cells, storage granules sometimes feature:
- Comprising glucose units, glycogen is a polysaccharide used as an energy store.
- Chains of phosphate groups employed in energy metabolism and control make up polyphosphate.
- Elemental sulphur kept for use as an energy source for sulfur-oxidizing bacteria is known as sulphur grains.
- One carbon storage polymer used by bacteria such as Cupriavidus necator is poly-β-hydroxybutyrate (PHB).
- In eukaryotic cells, storage granules might consist:
- Found in muscle and liver cells, glycogen granules offer easily available energy source.
- Storing neutral lipids like triglycerides, lipid droplets act as structural elements and energy stores.
- Storage granules serve among other purposes:
- Energy storage is supplying a source of energy in times of metabolic demand or food shortage.
- Maintaining cellular homeostasis by control of important metabolite availability is metabolic regulation.
- Detoxification is the process of sequesters possibly toxic compounds, like extra phosphate or sulphur, so preventing cellular damage.
- Sometimes conserving necessary components helps to maintain the structural integrity of cells.
- Insights into cellular energy management, metabolic control, and environmental adaptability depend on an awareness of storage granule composition and function.
23. Vacuole
Vacuoles are membrane-bound cells that vary in size within cells of various species of organisms.
Structure of Vacuole
- Found mostly in plant, fungal, and certain protist cells, a vacuole is a membrane-bound organelle found in the cytoplasm of eukaryotic cells; some animal cells also include vacuoles.
- Usually big, fluid-filled vesicles with several purposes including storage, waste disposal, and preserving cellular homeostasis, vacuoles are
- The tonoplast, the membrane around the vacuole, controls the flow of ions and other molecules into and out of the vacuole.
- A major component of plant cells, the central vacuole fills a large fraction of their volume and helps to generate turgor pressure, therefore preserving structural support and stiffness of the cell.
- Cell sap, or vacuoles, can have water, ions, carbohydrates, amino acids, pigments, and waste materials among their contents.
- By regulating the water to solute balance inside the cell, vacuoles can also be involved in osmoregulation.
- Vacuoles are engaged in several unicellular organisms in activities including digestion, excretion, and ejection of extra water.
- The kind of cell and organism will affect the shape and purpose of vacuoles, therefore reflecting their adaptation to various physiological demands.
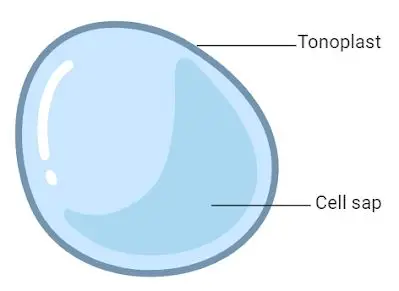
Functions of Vacuole
- Storage of Nutrients and Metabolites
- Vacuoles store essential substances such as sugars, amino acids, ions, and secondary metabolites like alkaloids and anthocyanins.
- In seeds, they serve as storage sites for proteins and other nutrients necessary for germination and early growth.
- They also store waste products and harmful substances, isolating them from the rest of the cell to prevent damage.
- Regulation of Turgor Pressure
- Vacuoles maintain turgor pressure by absorbing water through osmosis, which helps keep the cell rigid and supports plant structure.
- This pressure is vital for maintaining cell shape and enabling growth without the need for additional cytoplasm expansion.
- pH and Ionic Balance
- Vacuoles help regulate the pH of the cell by pumping protons (H⁺ ions) into their lumen, maintaining an acidic environment that is essential for enzyme activity and cellular processes.
- They also play a role in maintaining ionic balance by sequestering excess ions, thereby protecting the cell from potential toxicity.
- Degradation and Detoxification
- Vacuoles contain hydrolytic enzymes that break down macromolecules and cellular debris, functioning similarly to lysosomes in animal cells.
- They sequester and neutralize harmful compounds, including xenobiotics and metabolic by-products, contributing to cellular detoxification.
- Defense Mechanisms
- In plants, vacuoles store compounds like protease inhibitors that protect against herbivory by deterring or inhibiting the digestion of plant proteins by herbivores.
- They can also store antimicrobial compounds that protect against pathogens.
- Facilitation of Cell Growth
- Vacuoles contribute to cell growth by absorbing water, leading to an increase in cell size without the need for new cytoplasm.
- This process is particularly important in non-woody plants where growth is primarily driven by vacuolar expansion.
- Involvement in Endocytosis and Exocytosis
- In animal cells, vacuoles participate in endocytosis by engulfing extracellular material, which is then transported into the cell.
- They also facilitate exocytosis by transporting waste or secretory products to the cell surface for removal or secretion.
- Signaling and Homeostasis
- Vacuoles participate in cellular signaling pathways by storing and releasing ions and other molecules that act as secondary messengers.
- They help maintain cellular homeostasis by regulating the internal environment in response to external changes.
- Contribution to Plant Pigmentation
- Vacuoles contain pigments like anthocyanins that contribute to the coloration of plant tissues, which can attract pollinators or deter herbivores.
- Adaptation to Environmental Stress
- Vacuoles help plants adapt to environmental stresses such as drought or salinity by adjusting their osmotic potential and storing compatible solutes.
24. Vesicles
Vesicles are the structures in cells. They can be formed naturally through processes such as exocytosis, endocytosis or transport of material within the cell, or they may be created artificially, they are referred to as liposomes. There are different kinds of vesicles such as vacuoles, transport and secretory vesicles, based on their functions.
Structure of Vesicles
- Comprising fluid or cytoplasm contained by a lipid bilayer, a vesicle is a tiny, membrane-bound structure either inside or outside of a cell.
- Amphipathic phospholipids make up the lipid bilayer of vesicles; a semi-permeable barrier is created by hydrophilic heads facing outward and hydrophobic tails facing inside.
- Because its inside is chemically different from cytosol, a vesicle can separate molecules for digestion, storage, or transportation.
- As in the case of liposomes, vesicles can arise spontaneously by activities including secretion (exocytosis), absorption (endocytosis), and intracellular transport, or artificially.
- By fusing with other membranes inside the cell system, the membrane of a vesicle helps materials be transferred to the plasma membrane or between organelles.
- Among the several cellular processes, including waste disposal, nutrition absorption, and intercellular communication, vesicles are absolutely important.
- Types of vesicles include:
- Transport vesicles: Involved in moving materials between organelles.
- Secretory vesicles: Carry substances to be secreted outside the cell.
- Lysosomes: Contain enzymes for intracellular digestion.
- Peroxisomes: Contain enzymes that oxidize certain molecules.
- Vacuoles: Larger vesicles primarily involved in storage and maintaining cell turgor.
- Multivescular bodies (MVBs), which are vital for protein sorting and degradation within the cell, are produced by the endosomal sorting complexes needed for transport (ESCRT).
- Multiple protein complexes (ESCRT-0, I, II, III) make up the ESCRT system and help membrane budding and scission during events like viral budding and cytokinesis.
- Damage of ESCRT function is linked to neurodegenerative illnesses including Alzheimer’s and Parkinson’s and can cause buildup of defective proteins.
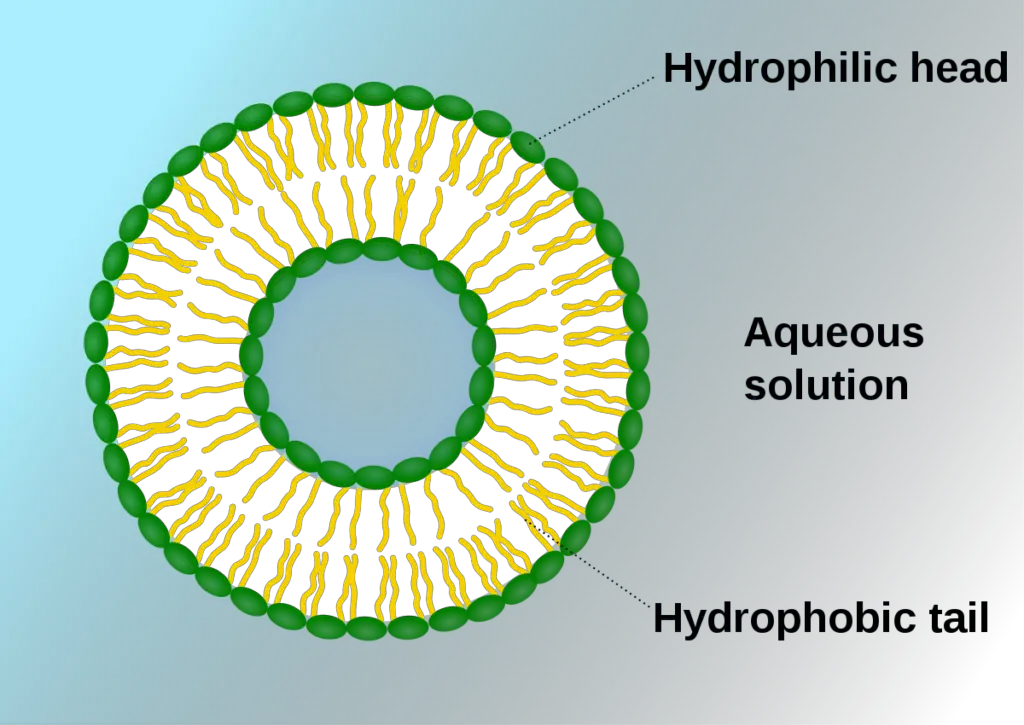
Functions of Vesicles
- Essential components of cellular operations like transport, storage, digesting, and signalling, vesicles are membrane-bound structures.
- Transportation Vesicles:
- Help molecules migrate between several cell compartments.
- From the endoplasmic reticulum, carry proteins and lipids to the Golgi apparatus and from the Golgi to various sites either inside or outside of the cell.
- Choose cargo and direct vesicle trafficking using coat proteins including COPI, COPII, and clathrin.
- Vesicles of secretory nature:
- Stow and move molecules like hormones, neurotransmitters, and enzymes to be released from the cell.
- Exocytosis responds to particular signals releases their contents into the extracellular area.
- Help to preserve homeostasis and participate in intercellular communication.
- Lysosomes are:
- Hold hydrolytic enzymes for breaking down and recycling foreign materials, damaged organelles, and cellular trash.
- Maintaining cellular health requires avoiding the buildup of harmful toxins.
- Peroxisumes:
- Include detoxifying agents like hydrogen peroxide and enzymes that break down fatty acids.
- Help processes of cellular detoxification and lipid metabolism.
- Vacuocytes:
- Present as nutrient, waste product, and other chemical storage spaces.
- The central vacuole of plant cells contains poisons and pigment as well as helps to preserve turgor pressure.
- Exosodes:
- Little vesicles moving proteins, lipids, and RNA between cells in intercellular communication.
- Take part in immunological reactions and could help to explain the course of diseases.
- Multivesicular bodies (MVBs):
- Developed from endosomal membranes’ inward budding to form vesicles.
- Participated in protein breakdown and sorting to help cells to remain homeostasis.
- Proteins from SNARE:
- Recognising and binding complementary SNAREs on the vesicle and target membranes will help you to mediate the fusing of these structures.
- Guarantee of specificity in vesicle targeting and fusing is crucial for appropriate intracellular movement.
- GTPases related to Rab:
- Interacting with SNARE proteins and other effectors, operate as molecular switches controlling vesicle transit.
- Guide vesicles to their proper sites to guarantee precise intracellular movement.
A Brief Summary on Cell Organelles
Cell Organelles | Structure | Functions |
Cell membrane | A double membrane composed of lipids and proteins. Present both in plant and animal cell. | Provides shape, protects the inner organelle of the cell and acts as a selectively permeable membrane. |
Centrosomes | Composed of Centrioles and found only in the animal cells. | It plays a major role in organizing the microtubule and Cell division. |
Chloroplasts | Present only in plant cells and contains a green-coloured pigment known as chlorophyll. | Sites of photosynthesis. |
Cytoplasm | A jelly-like substance, which consists of water, dissolved nutrients and waste products of the cell. | Responsible for the cell’s metabolic activities. |
Endoplasmic Reticulum | A network of membranous tubules, present within the cytoplasm of a cell. | Forms the skeletal framework of the cell, involved in the Detoxification, production of Lipids and proteins. |
Golgi apparatus | Membrane-bound, sac-like organelles, present within the cytoplasm of the eukaryotic cells. | It is mainly involved in secretion and intracellular transport. |
Lysosomes | A tiny, circular-shaped, single membrane-bound organelles, filled with digestive enzymes. | Helps in the digestion and removes wastes and digests dead and damaged cells. Therefore, it is also called as the “suicidal bags”. |
Mitochondria | An oval-shaped, membrane-bound organelle, also called as the “Power House of The Cell”. | The main sites of cellular respiration and also involved in storing energy in the form of ATP molecules. |
Nucleus | A largest, double membrane-bound organelles, which contains all the cell’s genetic information. | Controls the activity of the cell, helps in cell division and controls the hereditary characters. |
Peroxisome | A membrane-bound cellular organelle present in the cytoplasm, which contains the reducing enzyme. | Involved in the metabolism of lipids and catabolism of long-chain fatty acids. |
Plastids | Double membrane-bound organelles. There are 3 types of plastids:Leucoplast –Colourless plastids.Chromoplast–Blue, Red, and Yellow colour plastids.Chloroplast – Green coloured plastids. | Helps in the process of photosynthesis and pollination, Imparts colour for leaves, flowers and fruits and stores starch, proteins and fats. |
Ribosomes | Non-membrane organelles, found floating freely in the cell’s cytoplasm or embedded within the endoplasmic reticulum. | Involved in the Synthesis of Proteins. |
Vacuoles | A membrane-bound, fluid-filled organelle found within the cytoplasm. | Provide shape and rigidity to the plant cell and helps in digestion, excretion, and storage of substances. |
FAQ
What is a cell organelle?
A cell organelle is a specialized structure within a cell that performs a specific function.
What are some examples of cell organelles?
Some examples of cell organelles include the nucleus, mitochondria, ribosomes, endoplasmic reticulum, Golgi apparatus, lysosomes, and peroxisomes.
What is the function of the mitochondria?
The mitochondria are responsible for producing energy for the cell through the process of cellular respiration.
What is the function of the endoplasmic reticulum?
The endoplasmic reticulum is responsible for protein synthesis and lipid metabolism.
What is the function of the Golgi apparatus?
The Golgi apparatus is responsible for modifying, sorting, and packaging proteins and lipids for transport within or outside the cell.
What is the function of lysosomes?
Lysosomes are responsible for the breakdown of cellular waste and debris.
What is the function of peroxisomes?
Peroxisomes are responsible for breaking down toxic substances within the cell.
What is the function of ribosomes?
Ribosomes are responsible for protein synthesis.
How do cells maintain the proper functioning of their organelles?
Cells maintain the proper functioning of their organelles through a variety of mechanisms, including regulating gene expression, recycling damaged organelles, and targeting dysfunctional organelles for degradation.
What happens when organelles malfunction or are damaged?
When organelles malfunction or are damaged, it can lead to cellular dysfunction and disease. Cells have various mechanisms for repairing or replacing damaged organelles, but severe or chronic damage can lead to irreversible cellular damage or death.