What is Cell Differentiation?
- Cell differentiation is a fundamental biological process in which unspecialized cells, known as stem cells, transform into specialized cells with unique structures and functions. This transformation is essential in the development of a multicellular organism, from a simple zygote (fertilized egg) to a complex system of tissues and cell types. Differentiation occurs through the systematic expression and regulation of specific genes, which guide a cell to adopt particular traits needed for specialized roles.
- During early human development, differentiation begins with the zygote, which divides and progressively forms cells specialized in distinct roles such as muscle, neuron, or blood cells. These early-stage stem cells, located in embryos, have high potency, meaning they can differentiate into a wide variety of cell types. Even in adulthood, certain stem cells retain this ability, such as those involved in tissue repair, where they divide to replace damaged or aging cells. While animal cells generally lose the capacity for differentiation after maturation, many plant cells maintain this ability throughout their lifespan.
- Differentiation modifies numerous cell characteristics, including size, shape, membrane potential, metabolic activity, and responsiveness to signals. These changes are driven by complex regulatory mechanisms involving gene expression, often without altering the cell’s DNA sequence. This selective gene expression and the resulting cellular modifications are the focus of epigenetics. Differences in gene expression can lead to significant variations in metabolic makeup and physical properties, allowing cells with identical genomes to develop distinct identities and functions.
- The differentiation process also includes specialized stages, such as terminal differentiation, where certain precursor cells exit the cell cycle permanently and adopt specific roles, such as those in the nervous system or muscle tissue. After reaching this terminal state, some cells may undergo further functional changes depending on their environmental cues or physiological needs.
- Levels of cellular potency vary, dictating a cell’s potential to differentiate. Totipotent cells, like those in the early embryo, can become any cell type, including placental cells. Pluripotent cells, such as embryonic stem cells, can develop into nearly all cell types within the organism. Multipotent cells are more limited, differentiating only into closely related cell types, while oligopotent cells can only develop into a few specific types, and unipotent cells are restricted to a single cell type but maintain the capacity for self-renewal.
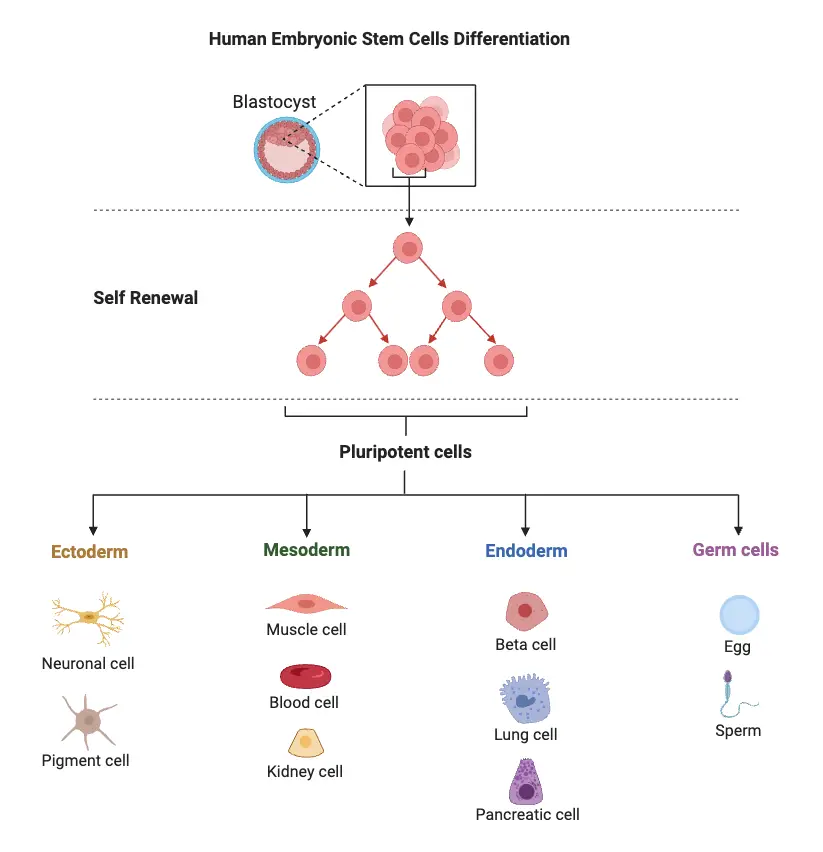
Definition of Cell Differentiation
Cell differentiation is the process by which unspecialized cells, like stem cells, transform into specialized cells with distinct structures and functions, enabling the development and maintenance of complex tissues and organs in multicellular organisms.
Factors Influencing Cell Differentiation
In multicellular organisms, several key factors influence cell differentiation, the process through which cells become specialized for distinct roles. Differentiation is triggered from the earliest stages of life, starting with a fertilized egg that divides and diversifies into cells with unique functions.
Key Factors Affecting Cell Differentiation:
- Gene Structure and Expression
- Gene Structure plays a central role, as each gene carries instructions for the specific characteristics of a cell.
- Every cell in an organism has the same set of genes, inherited from the fertilized egg.
- However, cells activate only the genes necessary for their particular function, “switching off” the rest.
- Mistakes in gene structure or expression can disrupt differentiation, leading to issues in cell function and organism development.
- Environmental Determinants
- External factors like temperature, oxygen levels, and nutrient availability impact differentiation.
- These factors influence hormone production and protein function, which in turn affect cell signaling pathways.
- Changes in these environmental conditions can alter gene expression, impacting the differentiation and growth processes.
- Cell Signaling and Interactions
- Cells communicate with each other through signaling molecules, guiding differentiation decisions.
- This cell-to-cell communication helps synchronize the development of tissues and ensures that cells take on roles appropriate to their location and function.
- Signaling is essential during the repair of damaged tissues, where it directs undifferentiated cells to become the cell types needed for repair.
- Influence of Cytoplasmic Components
- The cytoplasm—the fluid within a cell that contains organelles and molecules—also contributes to differentiation.
- Differences in the concentration of molecules within the cytoplasm can influence gene expression.
- This variation can help direct cells toward specific types during early stages of development.
- Hormonal Influences
- Hormones act as chemical messengers that play a critical role in regulating differentiation, especially during growth and repair.
- Hormonal signals can activate specific gene expressions, guiding cells to develop into specialized types, such as muscle or nerve cells.
Examples of Cell Differentiation Occurrences:
- Routine Cell Turnover
- In adults, many cells (e.g., blood cells) undergo regular turnover, requiring stem cells to differentiate and replace old or damaged cells.
- Growth and Maturation
- As an immature organism grows, differentiation occurs continuously, guiding cells to form tissues, organs, and complex systems.
- Tissue Repair
- When tissues are damaged, specialized cells are often needed to replace those lost to injury.
- During this repair, cell signaling helps undifferentiated cells become the necessary cell types.
In both animals and plants, differentiated cells retain all the genes needed for complex development. The specific patterns of gene expression, however, vary across cell types, guiding each one into its unique, functional form.
Mechanism of Cell Differentiation
Cell differentiation transforms a cell from an unspecialized state to a specialized one, relying on complex processes involving transcription factors, gene regulation, and cell signaling. Each cell type emerges from a unique pattern of gene expression, shaped by multiple mechanisms and interactions.
Key Mechanisms of Cell Differentiation:
- Transcription Factors and Gene Expression
- Transcription factors are proteins that bind to specific DNA sequences, influencing the transcription of DNA to RNA.
- These factors guide gene expression by regulating which genes are active, effectively dictating the cell’s identity.
- The specific gene expression pattern of each cell type is maintained through networks of regulatory genes and modules.
- Gene Regulatory Networks
- Gene regulatory networks consist of interconnected genes that act together to govern cell behavior.
- These networks create predictable patterns by managing which genes are activated or suppressed in response to signals.
- Each node within this network processes input and sends output to control differentiation, helping cells follow distinct developmental pathways.
- Signal Transduction Pathways
- Signal transduction pathways involve cell signaling molecules like growth factors that control cell differentiation.
- Ligands produced by one cell bind to receptors on neighboring cells, initiating a cascade of reactions.
- This binding changes the receptor’s shape, activating its enzymatic function, which phosphorylates downstream proteins.
- These phosphorylation reactions trigger dormant transcription factors, stimulating changes that drive the cell toward its specialized state.
- Competence and Induction
- Cells vary in competence—their ability to respond to external signals.
- Signal induction is when a cell or tissue influences another cell’s developmental pathway through a series of signaling events.
- For instance, certain cells can induce eye development by signaling other cells to form the required structures, a process shown in studies with cave- and surface-dwelling fish.
- Asymmetric Cell Division
- Asymmetric cell divisions yield daughter cells with different developmental fates by distributing regulatory molecules unevenly.
- Maternal cytoplasmic determinants—molecules unevenly divided during cell division—create distinct differentiation patterns for each daughter cell.
- This mechanism is well-documented in species like Drosophila, where it establishes the body axis, and in Volvox, where cell size after division dictates whether a cell becomes germ or somatic.
- Role of RNA in Intracellular Control
- RNA molecules serve as important differentiation signals within cells.
- They help transmit regulatory instructions that guide the differentiation pathways of specific cell types.
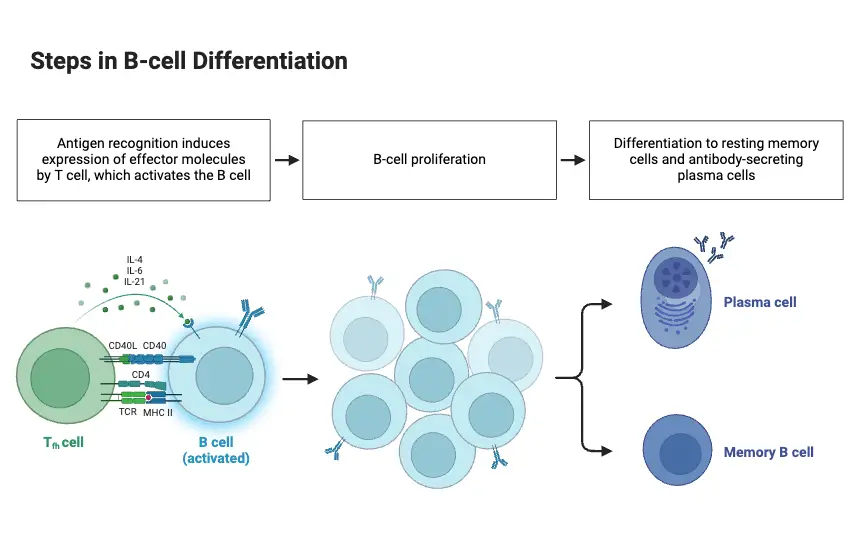
Examples of Cell Differentiation
Cell differentiation is fundamental in both plants and animals, where it transforms unspecialized cells into specialized types. Each organism starts from a single cell and, through differentiation, develops diverse cell types essential for growth and function.
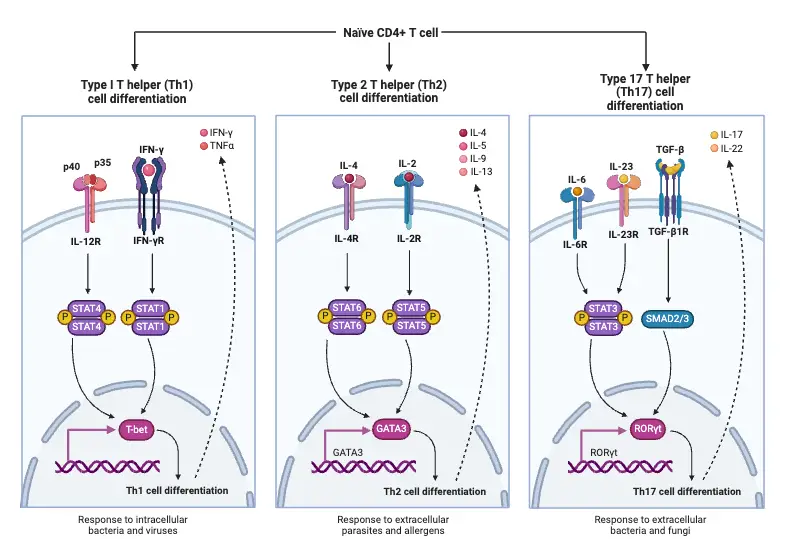
Examples in Plants
- Seed and Zygote Development
- Plant differentiation begins from a single fertilized cell within a seed, much like an animal egg.
- The seed nourishes and protects the zygote, which divides and forms an embryo.
- When a seed disperses, further development pauses until conditions are suitable for growth.
- Meristems and Stem Cells
- The embryo develops meristems, which are specialized stem cells responsible for plant growth.
- Meristems divide into new cells—some move outward to form external structures, while others contribute to internal growth.
- These regions are found in both root and shoot tips and generate cells for plant organs.
- Root Development and Root Cap
- In roots, meristematic cells form a root cap, a protective layer that sheds as roots grow through soil.
- The root cap constantly regenerates, shielding the root as it pushes through tough environments.
- Leaf and Stem Formation
- Meristematic cells at the surface differentiate into structures like leaves and stems, each with specialized roles.
- Inner meristem cells develop into vascular tissues for nutrient transport, creating a system similar to the organs found in animals.
Examples in Animals
- Totipotent Cells and Zygote Formation
- In animals, differentiation begins with fertilization, producing a totipotent zygote—a cell capable of forming any cell type.
- This single zygote divides and specializes to form all the complex tissues and organs in the animal body.
- Early Stages of Differentiation
- Differentiation initiates early in animal development, with cells gradually adopting roles and forming structures.
- Specialized cells in tissues and organs allow complex animals to function and adapt to various environments.
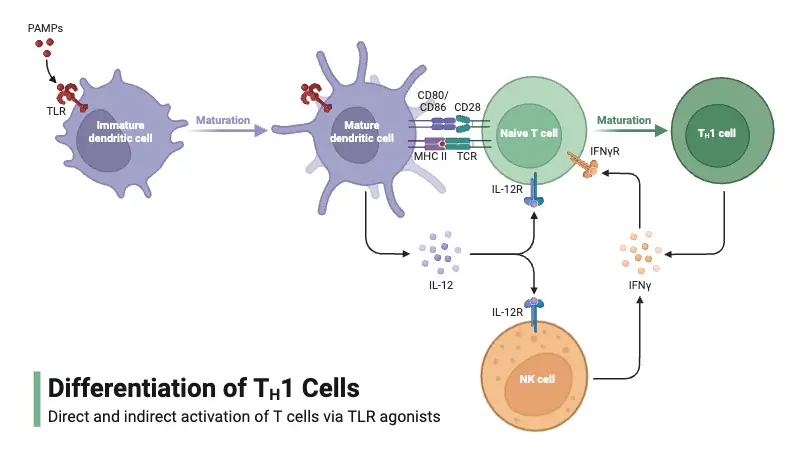
Cell Differentiation – Errors
Errors in cell differentiation lead to abnormalities that can disrupt normal development and function. These errors are classified into three categories: anaplasia, dysplasia, and metaplasia.
- Anaplasia
- Anaplasia is the complete loss of normal differentiation in cells, often occurring in the later stages of cancer.
- Initially, cancer cells resemble the tissue they originated from, maintaining some level of differentiation.
- As the tumor progresses, cellular features become more irregular, and the cells become increasingly unrecognizable.
- Eventually, in advanced cancer, the cells no longer resemble their parent tissue and exhibit a highly anaplastic growth pattern, indicating the loss of differentiation.
- Dysplasia
- Dysplasia refers to disordered cell arrangement, where the growth pattern of cells is disrupted.
- This abnormality arises due to stress or damage in the tissue, often leading to structural changes.
- Dysplastic cells can be precursors to cancer but not all cases lead to malignancy.
- In some instances, the abnormal cells may resolve and return to normal growth, such as in CIN (cervical intraepithelial neoplasia).
- Dysplasia is often an indicator of potential future problems, though it may not always be harmful in the short term.
- Metaplasia
- In metaplasia, one cell type is replaced by another, usually as a response to chronic damage or irritation.
- It typically occurs when a tissue undergoes significant regeneration after prolonged injury.
- Squamous metaplasia in the bronchi is a common example, where the normal epithelial cells are replaced by squamous cells in response to smoking or other irritants.
- Though metaplasia is reversible, it can sometimes lead to further complications if the underlying cause of the damage persists.
Stem Cell Differentiation
Stem cells are unique in their ability to self-renew and differentiate into a variety of mature somatic cells. This ability makes them vital for growth, healing, and regeneration.
- Embryonic Stem Cells
- These stem cells are found in embryos and come in two types: totipotent and pluripotent.
- Totipotent stem cells can differentiate into every type of cell in the body.
- They are capable of forming both embryonic tissues and extra-embryonic tissues like the placenta.
- Pluripotent stem cells can differentiate into most cell types, but not all.
- These are typically found in the blastocyst stage of human embryos and can develop into nearly any cell type in the body, but not the supporting tissues like placenta.
- Adult Stem Cells
- These are found in adult organisms and are multipotent, meaning they can only differentiate into a limited range of cell types.
- Unlike embryonic stem cells, they do not form all types of tissues but are still crucial for tissue repair and regeneration.
- Examples of multipotent stem cells include hematopoietic stem cells that form blood cells and mesenchymal stem cells that form bone and cartilage cells.
In short, stem cells can be categorized based on their potential for differentiation:
- Totipotent: capable of forming all cell types, including extra-embryonic tissues.
- Pluripotent: can form nearly every cell type, but not the extra-embryonic tissues.
- Multipotent: can only form a restricted number of cell types, typically related to the tissue from which they originate.
- https://byjus.com/neet/cell-differentiation/
- https://studymind.co.uk/notes/cell-differentiation/
- https://shiken.ai/biology/cell-differentiation
- https://en.wikipedia.org/wiki/Cellular_differentiation
- https://www.sciencedirect.com/topics/biochemistry-genetics-and-molecular-biology/cellular-differentiation