What is Cardiac Cycle?
- Cell lines are essential tools in biological research and biomedical applications, serving as in vitro models to study cellular processes, drug responses, and disease mechanisms. The characterization of these cell lines is a critical step in ensuring the reliability and relevance of experimental results.
- To begin, cell lines are typically derived from primary cells through a process of immortalization, which allows them to proliferate indefinitely. This transformation can occur naturally or through manipulation techniques, such as viral infection or genetic modification. The resulting immortalized cell lines can be categorized based on their tissue of origin, such as epithelial, fibroblast, or hematopoietic cells. Each category exhibits unique growth characteristics and functional properties, influencing their suitability for specific research applications.
- One fundamental aspect of cell line characterization is the assessment of their morphological properties. Morphology can provide valuable insights into the health and differentiation state of the cells. For instance, changes in cell shape, size, and arrangement can indicate cellular stress or transformation. Techniques such as microscopy allow researchers to observe these morphological features directly, providing a visual context for further analyses.
- In addition to morphology, the characterization process includes evaluating the cell lines’ genetic and phenotypic profiles. This entails analyzing the expression of specific markers, which can be done through techniques such as flow cytometry or immunocytochemistry. By identifying surface antigens and other markers, researchers can confirm the identity of the cell line and ascertain its characteristics. For example, the expression of certain proteins may suggest the lineage of the cells or indicate their potential response to therapeutic agents.
- Another critical dimension of cell line characterization is assessing growth kinetics and culture conditions. This includes evaluating parameters such as doubling time, saturation density, and optimal growth media. Understanding these factors is vital for optimizing experimental setups and ensuring reproducibility. Furthermore, researchers must consider the impact of external stimuli, such as cytokines or growth factors, which can significantly influence cell behavior.
- The functional assays performed on cell lines are equally important for characterization. These assays may include evaluating cellular responses to drugs, assessing migratory behavior, or measuring metabolic activity. For instance, cancer cell lines might be subjected to chemotherapeutic agents to determine their sensitivity or resistance. These functional studies help establish the relevance of the cell lines as models for human disease.
- Additionally, genetic stability is a key consideration in the characterization of cell lines. Over time, continuous passage of cells can lead to genetic drift, which may alter their characteristics. Therefore, it is crucial to monitor genomic integrity through techniques such as karyotyping or next-generation sequencing. These assessments can reveal chromosomal abnormalities or mutations that could affect the outcome of experiments.
- Moreover, ethical considerations also play a role in cell line characterization. Many cell lines are derived from human tissues, necessitating compliance with ethical standards regarding consent and use. It is imperative for researchers to be aware of and adhere to these ethical guidelines when utilizing cell lines in their studies.
Cardiac Cycle Phases
The cardiac cycle refers to the sequence of events that occurs from the beginning of one heartbeat to the beginning of the next. This cycle consists of two primary phases: ventricular contraction, known as systole, and ventricular relaxation, referred to as diastole. Each of these phases can be further subdivided into distinct stages characterized by specific pressure and volume changes.
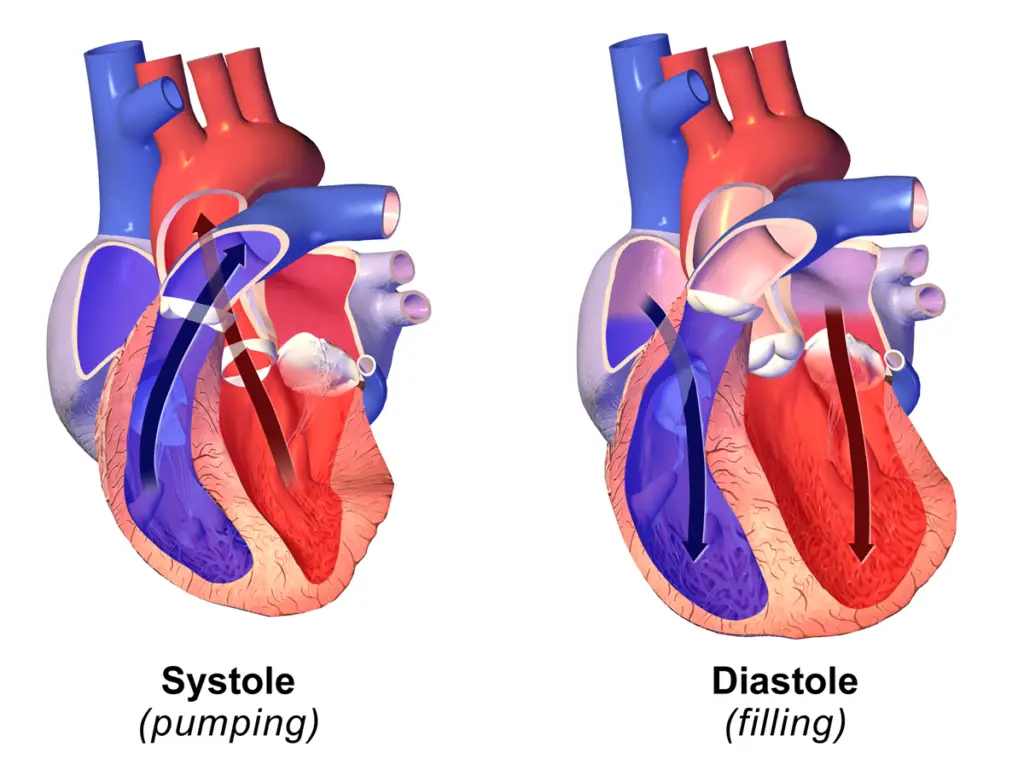
- Systole: This phase encompasses the contraction of the ventricles and is crucial for the ejection of blood from the heart.
- Isovolumic Contraction: This initial phase of systole occurs when the ventricles begin to contract. During this period, the volume of blood within the ventricles remains constant, despite the increasing pressure as the ventricular muscle contracts. The atrioventricular (AV) valves close at this time, preventing backflow into the atria, while the aortic and pulmonary valves remain closed, setting the stage for effective blood ejection.
- Ejection Phase: Following isovolumic contraction, the pressure within the ventricles rises sufficiently to exceed that in the aorta and pulmonary artery. This leads to the opening of the aortic and pulmonary valves, allowing blood to be ejected from the ventricles into the systemic and pulmonary circulation. This phase is characterized by a significant decrease in ventricular volume as blood is expelled from the heart.
- Diastole: This phase involves the relaxation of the ventricles and the filling of the heart chambers.
- Isovolumic Relaxation: At the beginning of diastole, the ventricles relax, causing a drop in pressure. Both the aortic and pulmonary valves close to prevent backflow of blood. During this phase, the volume of blood in the ventricles remains unchanged as the heart prepares for the next filling cycle.
- Rapid Ventricular Filling: Following isovolumic relaxation, the pressure in the ventricles decreases further, leading to the opening of the AV valves. Blood flows rapidly from the atria into the ventricles, filling them quickly. This phase is crucial for ensuring that the ventricles have adequate blood volume for the next contraction.
- Slow Ventricular Filling (Diastasis): As the ventricles fill, the rate of filling slows down. This phase, also known as diastasis, allows for the gradual filling of the ventricles as the pressure in the atria remains higher than in the ventricles. This stage is essential for optimizing the preload on the ventricles.
- Atrial Contraction: The final stage of diastole is characterized by atrial contraction. The atria contract, pushing additional blood into the ventricles, ensuring they are optimally filled before the next ventricular contraction begins. This phase enhances ventricular filling and is critical for maintaining effective cardiac output.
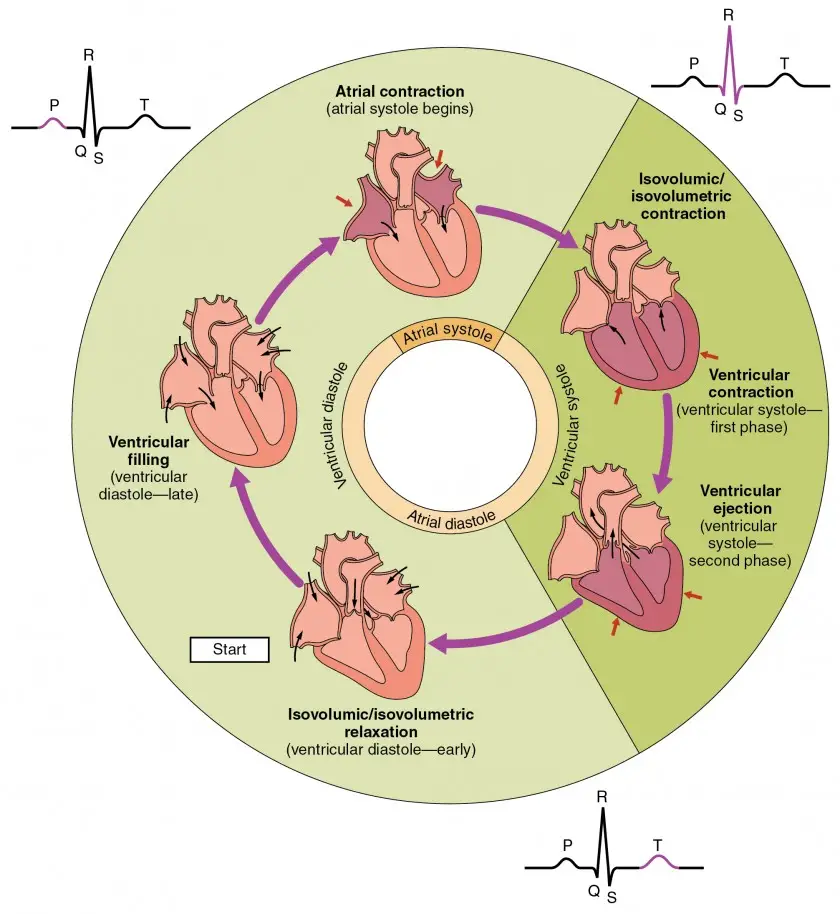
1. Isovolumic Contraction
Isovolumic contraction is a critical phase of the cardiac cycle, occurring immediately after the ventricles begin to contract. This phase is marked by significant physiological changes that set the stage for effective blood ejection into the systemic and pulmonary circulations.
- Ventricular Depolarization: The onset of isovolumic contraction is initiated by ventricular depolarization, which results in the rapid increase in pressure within the ventricles. As the ventricles contract, the pressure rises above that in the atria, leading to the closure of the atrioventricular (AV) valves. Consequently, this prevents blood from flowing back into the atria during contraction.
- Pressure and Volume Dynamics: During this phase, the ventricles experience a marked increase in pressure while the blood volume remains unchanged, hence the term “isovolumic.” At this point, all heart valves are closed, meaning that no blood is ejected from the ventricles. The ventricular pressure rises significantly, reaching levels necessary for the subsequent opening of the semilunar valves. The blood volume within the ventricles at this stage is equivalent to the end-diastolic volume, which is approximately 130 mL.
- Atrial Response: As the pressure within the ventricles increases, it causes the AV valves to bulge backward into the atria. This mechanical event is responsible for the “c wave” observed in the venous pulse, indicating the effect of increasing ventricular pressure on the atrial structures.
- Arterial Pressure Changes: During isovolumic contraction, the pressures within the systemic and pulmonary arteries gradually decrease. This decrease is a reflection of the dynamic interplay between ventricular contraction and arterial compliance.
- Electrocardiogram (ECG) Changes: The electrical events accompanying isovolumic contraction can be observed on an electrocardiogram. Ventricular depolarization, which is responsible for this contraction, spreads from the atrioventricular node through the bundle of His and the Purkinje fibers, resulting in the QRS complex on the ECG. At the same time, the atria are repolarized, producing the atrial T wave (Ta). However, this wave is typically obscured by the more prominent QRS complex in standard ECG readings.
- Heart Sounds: The isovolumic contraction phase is also characterized by the production of the first heart sound, often denoted as S1. This sound arises from the vibrations of the AV valves, surrounding myocardium, and blood, all of which are affected by the closure of the AV valves at the start of this phase.
Therefore, isovolumic contraction plays a vital role in preparing the heart for effective blood ejection during the next phase of the cardiac cycle.
2. Ejection Phase
The ejection phase of the cardiac cycle is a critical period during which blood is expelled from the ventricles into the arterial system. This phase follows isovolumic contraction and is characterized by significant changes in pressure, volume, and electrical activity of the heart.
- Initiation of Ejection: The ejection phase begins when the left ventricular pressure surpasses the pressure in the aorta, and the right ventricular pressure exceeds that in the pulmonary artery. This pressure differential causes the semilunar valves to open, allowing blood to be forcefully ejected from both the left and right ventricles into the aorta and pulmonary artery, respectively. During this time, the atrioventricular valves remain closed to prevent backflow into the atria.
- Pressure and Volume Dynamics:
- During the initial part of the ejection phase, known as rapid ejection, the ventricular pressure increases significantly, facilitating the swift movement of blood into the arteries. As blood is ejected, the volume within the ventricles decreases.
- Subsequently, the pressure in the ventricles begins to decline during the second part of the ejection, referred to as slow ejection. This decline in pressure occurs as the pressure gradient between the ventricles and the arteries lessens, resulting in a slower rate of blood flow.
- The maximum ventricular pressures achieved during this phase are approximately 120 mmHg in the left ventricle and 25 mmHg in the right ventricle, known as systolic pressure.
- Stroke Volume and End-Systolic Volume: Under resting conditions, approximately 70 mL of blood is ejected from each ventricle during the ejection phase, termed the stroke volume. At the conclusion of systole, about 60 mL of blood remains in each ventricle, referred to as the end-systolic volume. The ejection fraction, defined as the ratio of stroke volume to end-diastolic volume, indicates the percentage of blood ejected from the ventricles during systole, typically around 60%.
- Atrial Dynamics: As the ventricles contract, they also shorten, leading to elongation of the atria and major veins. This action results in a decrease in pressure within the atria, which is reflected as an x wave in the venous pulse, indicating the impact of ventricular contraction on the atrial pressure dynamics.
- Arterial Pressure Changes: The blood pressure in the major arteries rises rapidly during the ejection phase, reaching systolic pressures of 120 mmHg in the aorta and 25 mmHg in the pulmonary artery. As the ejection transitions to slow ejection, the blood pressure in both the systemic and pulmonary circulations begins to decrease progressively.
- Electrocardiogram (ECG) Changes: The electrocardiogram provides insights into the electrical events that coincide with the ejection phase. At the onset of ejection, the ventricles are fully depolarized, marked by the ST segment on the ECG. As the phase progresses into the latter half, the appearance of the T wave signifies the repolarization of the ventricles, reflecting the end of the ejection process.
3. Isovolumic Relaxation
Isovolumic relaxation is a pivotal phase of the cardiac cycle that occurs immediately after systole. This period is characterized by a series of physiological changes that facilitate the transition from ventricular contraction to the filling phase. The events during isovolumic relaxation are essential for preparing the heart for the next cycle of blood ejection.
- Mechanics of the Heart: At the end of systole, the ventricles undergo relaxation, leading to a rapid decrease in ventricular pressure. During this phase, the inertia of the blood allows a small amount of blood to flow out of the ventricles, even when the pressure in the large arteries exceeds that of the ventricles. However, once the inertia is overcome, the elevated pressures in the aorta and pulmonary artery push blood back toward the ventricles, effectively closing the semilunar valves. The atrioventricular valves remain closed because the pressure in the atria is lower than that in the ventricles.
- Pressure and Volume Changes:
- Ventricles: During isovolumic relaxation, the ventricles relax without any change in their blood volume, which corresponds to the end-systolic volume, approximately 60 mL. This relaxation results in a significant drop in ventricular pressure, approaching near zero at the end of this phase.
- Atria: As the ventricles relax, blood continues to flow from the veins into the atria, even though the atrioventricular valves are still closed. This influx of blood causes the pressure in the atria to rise, leading to the formation of a v wave in the venous pulse, indicative of increasing atrial pressure.
- Arteries: The arterial pressure experiences a decrease; however, this decline is briefly interrupted by the appearance of a dicrotic notch in the aortic pulse. The dicrotic notch represents a momentary pressure increase caused by a brief period of backward blood flow just before the semilunar valves close.
- Electrocardiogram (ECG) Changes: During isovolumic relaxation, the process of ventricular repolarization is nearing completion, marked by the end of the T wave on the ECG. This electrical activity correlates with the mechanical relaxation of the ventricles, reflecting the heart’s transition toward the filling phase.
- Heart Sounds: The second heart sound, often referred to as “dub,” is generated during isovolumic relaxation. This sound arises from the vibrations of the semilunar valves, adjacent myocardium, and blood as the semilunar valves close. The sound provides an auditory indication of the transition between the phases of the cardiac cycle.
4. Rapid Ventricular Filling
The rapid ventricular filling phase is a critical component of the cardiac cycle that facilitates the efficient filling of the ventricles with blood following systole. This phase is marked by the opening of the atrioventricular valves, allowing blood to flow from the atria into the ventricles. Understanding this phase is essential for grasping the dynamics of cardiac function and hemodynamics.
- Mechanics of the Heart: As soon as the pressure within the ventricles decreases below that in the atria, the atrioventricular valves open, allowing blood to flow rapidly into the ventricles. During this time, the semilunar valves remain closed, preventing blood from returning to the ventricles from the aorta and pulmonary artery.
- Pressure and Volume Changes:
- Ventricles: This phase accounts for a significant portion of the total ventricular filling. Blood that had accumulated in the atria flows swiftly into the ventricles, resulting in an increase in ventricular volume. However, due to the ongoing relaxation of the ventricles, the ventricular pressure does not change significantly during this filling. This dynamic ensures that the ventricles can accommodate the incoming blood efficiently.
- Atria: The evacuation of blood from the atria to the ventricles produces a negative y wave in the venous pulse. This wave reflects the decrease in atrial volume as blood is drawn into the ventricles.
- Arteries: Following the closure of the semilunar valves, arterial pressure begins to decline gradually. Unlike the pressure fluctuations within the ventricles, the arterial pressure does not reach zero due to the elastic properties of the arterial walls. The minimum pressure observed during this phase, termed diastolic pressure, is approximately 80 mmHg in systemic circulation and 8 mmHg in pulmonary circulation.
- Electrocardiogram (ECG) Changes: During the rapid ventricular filling phase, no electrical activity is generated by the cardiac cells, leading to the presence of an isoelectric line on the ECG. This indicates a period of electrical inactivity while the ventricles are being filled.
- Heart Sounds: The third heart sound, though infrequent, may occur during this phase. It is thought to arise from the rapid influx of blood into the ventricles, which causes vibrations in the cardiac structures.
5. Slow Ventricular Filling
The slow ventricular filling phase is an essential part of the cardiac cycle that occurs after the rapid filling phase, contributing to the overall volume of blood in the ventricles. This phase is characterized by the gradual influx of blood from the atria into the ventricles, setting the stage for the next phase of ventricular contraction.
- Mechanics of the Heart: During this phase, the atrioventricular valves remain open, while the semilunar valves are closed. This configuration ensures that blood flows freely from the atria into the ventricles without any resistance from the arterial system.
- Pressure and Volume Changes:
- Ventricles: As diastole progresses, a small volume of blood begins to flow into the ventricles. This blood comes from the veins and passes through the atria, gradually filling the ventricles. During this phase, the pressure in both ventricles is close to zero, reflecting their relaxed state. This low pressure allows for efficient filling without the need for significant muscular contraction.
- Arteries: Concurrently, the pressures in the arteries of both the systemic and pulmonary circulations continue to decrease. This reduction in arterial pressure is vital as it enables the blood to flow from the higher-pressure atria into the lower-pressure ventricles.
- Electrocardiogram (ECG) Changes: At the conclusion of the slow ventricular filling phase, electrical depolarization spreads from the sinoatrial node throughout the atria. This activity is represented as the P wave on the ECG, indicating atrial depolarization. The appearance of the P wave signals the transition to the next phase of the cardiac cycle.
6. Atrial Systole
Atrial systole is the concluding phase of diastole in the cardiac cycle, marking the completion of ventricular filling. During this phase, the atria contract to propel blood into the ventricles, ensuring that they are adequately filled in preparation for the subsequent ventricular contraction.
- Mechanics of the Heart: During atrial systole, the atrioventricular valves are open, allowing blood to flow from the atria into the ventricles. Simultaneously, the semilunar valves remain closed to prevent backflow into the arterial system. The contraction of the atria is a critical mechanism that facilitates the final phase of filling the ventricles with blood.
- Pressure and Volume Changes:
- Ventricles: Approximately 25% of the total ventricular filling volume is ejected from the atria into the ventricles during atrial systole. Despite the influx of blood, the relaxed state of the ventricular myocardium results in negligible changes in ventricular pressure, which remains almost zero. By the end of atrial systole, each ventricle contains about 130 ml of blood, a volume referred to as the end-diastolic volume.
- Atria: The contraction of the atria leads to a rise in atrial pressure, which is represented by the a wave in the venous pulse. This increase in pressure is essential for efficiently pushing blood into the ventricles.
- Arteries: The arterial pressure in both systemic and pulmonary circulations continues to decrease throughout this phase, reflecting the overall changes in the cardiovascular system as blood is directed towards the ventricles.
- Electrocardiogram (ECG) Changes: The completion of atrial depolarization coincides with the end of the P wave on the ECG, signaling the onset of atrial systole. Following this, depolarization spreads from the atria to the atrioventricular node, resulting in the visibility of the PR segment on the ECG. This electrical activity provides a clear indication of the heart’s electrical conduction during atrial contraction.
- Heart Sounds: The fourth heart sound, a soft sound, may be produced due to the increase in ventricular pressure following atrial systole. This sound is uncommon in healthy individuals but may be audible under pathological conditions. It can occur due to elevated intra-atrial pressure or reduced compliance of the ventricle, as seen in cases of ventricular hypertrophy.
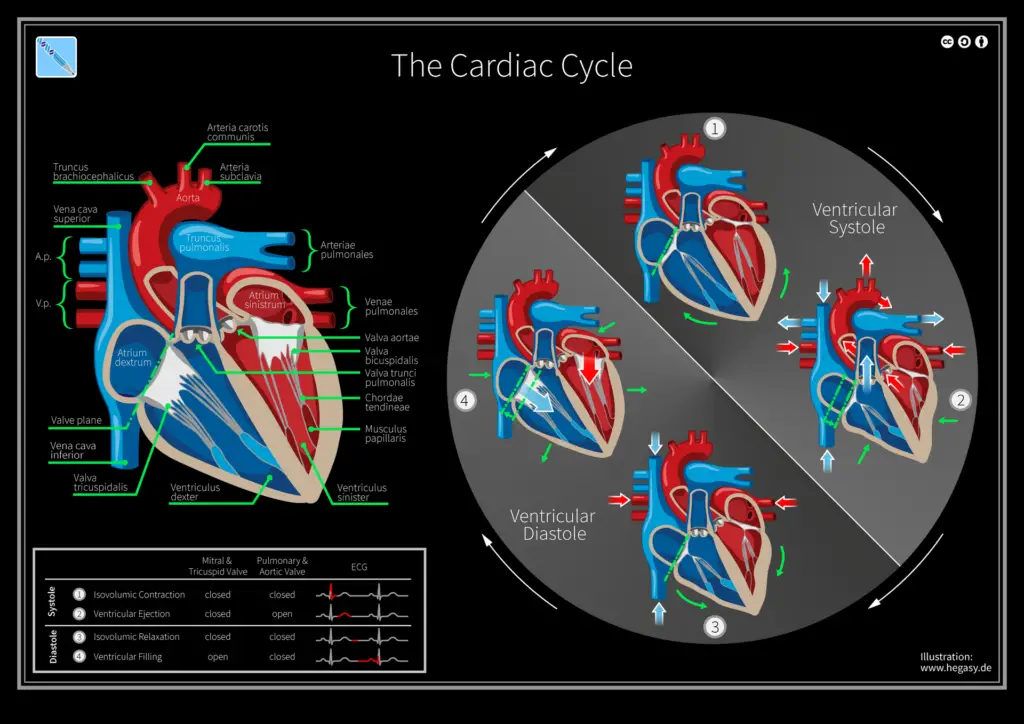
Cardiac Cycle Phases
The cardiac cycle consists of a series of phases that facilitate the effective pumping of blood through the heart and the circulatory system. Each phase is characterized by specific mechanical and electrical activities that ensure optimal function. The following phases outline the progression of the cardiac cycle:
- Atrial Diastole: During this initial phase, the chambers of the heart are in a relaxed state. The aortic valve and pulmonary artery are closed, while the atrioventricular valves are open. This allows the heart chambers to fill with blood from the veins, preparing them for the next contraction. The relaxation of the atria during this time is crucial for maintaining an efficient flow of blood into the ventricles.
- Atrial Systole: Following atrial diastole, the atria contract to propel blood into the ventricles. This phase ensures that the remaining blood in the atria is effectively transferred to the ventricles. The contraction of the atria increases atrial pressure, which facilitates the movement of blood into the ventricles, thereby completing the filling process.
- Isovolumic Contraction: In this phase, the ventricles begin to contract, causing a rise in ventricular pressure. The atrioventricular valves and the semilunar valves (including the pulmonary artery and aortic valves) close to prevent backflow. However, there is no change in the volume of blood within the ventricles during this stage, as the pressure builds up without any ejection of blood. This phase is essential for generating the pressure required for effective ventricular ejection.
- Ventricular Ejection: During ventricular ejection, the pressure in the ventricles exceeds that in the pulmonary artery and aorta, leading to the opening of the semilunar valves. Blood is forcefully ejected from the ventricles into the pulmonary artery and aorta. The ejection phase is critical for delivering oxygenated blood to the body and deoxygenated blood to the lungs for reoxygenation. At the conclusion of this phase, the pulmonary artery and aortic valves close to prevent backflow.
- Isovolumic Relaxation: In this phase, the ventricles stop contracting and begin to relax. No blood enters the ventricles during this time, leading to a decrease in ventricular pressure. The closure of the aortic and pulmonary valves occurs due to the pressure differences between the ventricles and the great arteries. This phase prepares the ventricles for the next filling cycle by allowing them to return to their resting state.
- Ventricular Filling Stage: The ventricular filling stage encompasses the rapid filling of the ventricles with blood from the atria. This phase includes both rapid and slow filling, ensuring that the ventricles are adequately filled before the next contraction. The filling process is essential for maintaining continuous blood flow throughout the cardiovascular system.
Duration of Cardiac Cycle
The duration of the cardiac cycle is a crucial aspect of cardiovascular physiology, reflecting the time taken for the heart to complete one full sequence of contraction and relaxation. In a typical individual, the heart beats approximately 72 times per minute. This frequency allows for the calculation of the duration of each cardiac cycle, which can be expressed in both minutes and seconds.
- Basic Calculation:
- With a heartbeat of 72 beats per minute, the duration of one cardiac cycle can be computed as follows:
- Duration of Cardiac Cycle Phases: The overall duration of the cardiac cycle can be further broken down into its constituent phases, each contributing to the cycle’s total time. The approximate durations of these phases are as follows:
- Atrial Systole: This phase lasts about 0.1 seconds. During atrial systole, the atria contract to push blood into the ventricles, thus facilitating efficient filling.
- Ventricular Systole: This phase continues for approximately 0.3 seconds. Here, the ventricles contract forcefully to eject blood into the aorta and pulmonary artery, delivering oxygenated blood to the body and deoxygenated blood to the lungs.
- Atrial Diastole: During this stage, lasting around 0.7 seconds, the atria are relaxed and fill with blood returning from the systemic and pulmonary circulations. This relaxation phase is vital for preparing the atria for the next contraction.
- Ventricular Diastole: This phase occurs for about 0.5 seconds. In ventricular diastole, the ventricles relax and fill with blood from the atria, setting the stage for the subsequent contraction.
Therefore, the coordinated timing of these phases is essential for maintaining effective blood circulation and ensuring that the heart operates efficiently. The total duration of the cardiac cycle—approximately 0.8 seconds—allows for a rhythmic and organized flow of blood through the heart, emphasizing the significance of each phase in the overall process.
- Pollock JD, Makaryus AN. Physiology, Cardiac Cycle. [Updated 2022 Oct 3]. In: StatPearls [Internet]. Treasure Island (FL): StatPearls Publishing; 2024 Jan-. Available from: https://www.ncbi.nlm.nih.gov/books/NBK459327/
- https://www.kenhub.com/en/library/physiology/cardiac-cycle
- https://courses.lumenlearning.com/suny-ap2/chapter/cardiac-cycle/
- https://www.osmosis.org/learn/Cardiac_cycle
- https://cvil.ucsd.edu/wp-content/uploads/2017/02/cardiac-cycle.pdf
- https://en.wikipedia.org/wiki/Cardiac_cycle