What is Pteridophyta?
- Pteridophyta, commonly known as pteridophytes, are a group of vascular plants that reproduce via spores instead of seeds or flowers. As non-flowering plants, they are sometimes referred to as “vascular cryptogams,” meaning their reproductive structures are hidden. This group includes ferns, horsetails, and lycophytes (like clubmosses, spikemosses, and quillworts). Though historically classified as “Pteridophyta,” this term is no longer widely accepted since ferns and horsetails are more closely related to seed plants than lycophytes. However, the study of these plants remains significant, and terms like pteridophyte, pteridology, and pteridologist are still commonly used in scientific communities.
- The name “Pteridophyta” is derived from the Greek words “pteron,” meaning feather, and “phyton,” meaning plant, which refers to their feather-like fronds. Pteridophytes are some of the earliest vascular plants on Earth, with a rich history that dates back around 400 million years to the Silurian period of the Paleozoic era. These plants thrived during the Devonian period and played a dominant role in Earth’s vegetation from the Devonian to the Permian periods. Fossil evidence of pteridophytes has been found in rocks from the Silurian and Devonian periods, highlighting their long-standing presence on the planet.
- One of the most distinctive features of pteridophytes is their well-developed vascular system, consisting of xylem and phloem, which allows for efficient water and nutrient transport throughout the plant. This adaptation enabled them to colonize land environments effectively. Pteridophytes are considered the first true land plants, making them an important subject for understanding plant evolution and the adaptations that facilitated terrestrial life.
- The life cycle of pteridophytes involves an alternation of generations, with two distinct phases: the gametophyte and sporophyte. Unlike bryophytes, where the gametophyte is the dominant phase, in pteridophytes, the sporophyte is the predominant generation. The sporophyte, being more robust and better suited to terrestrial environments, allows the plant to thrive in land habitats. The gametophyte, on the other hand, is typically smaller and shorter-lived.
- With around 12,000 species of pteridophytes existing today, these plants remain a diverse and ecologically important group. Though they no longer dominate Earth’s vegetation as they once did during the Paleozoic era, their evolutionary significance and role in understanding plant adaptations to land continue to make them a focus of botanical research.
General Characteristics of Pteridophytes
Pteridophytes are an ancient group of vascular plants known for their distinctive features and diverse forms. They primarily thrive in moist, shaded environments, although some adapt to aquatic or xerophytic conditions. Here are the key characteristics of pteridophytes, focusing on their morphology, structure, and reproductive processes:
- Habitat Preferences:
- Most pteridophytes are terrestrial, preferring cool, moist, and shaded areas, as seen in ferns.
- Some species are aquatic, such as Marsileci and Azolla, while others can survive in dry, xerophytic conditions (Selaginella rupestris, Equisetum).
- A few species are epiphytic, like Lycopodium squarrosum, growing on other plants.
- Growth Forms:
- Pteridophytes are predominantly herbaceous. However, certain species, such as Angiopteris, are perennial and can grow into tree-like forms.
- The size range varies, with the smallest pteridophyte being Azolla (an aquatic fern) and the largest being the tree fern Cyathea.
- Sporophytic Plant Body:
- The dominant phase of the plant body is the sporophyte, which is well-differentiated into roots, stems, and leaves.
- The roots are adventitious and can exhibit monopodial or dichotomous branching. Internally, the roots often have a diarch structure.
- Stem Characteristics:
- Stems in pteridophytes are typically branched, with either monopodial or dichotomous branching patterns.
- Branches do not arise from the axils of the leaves, and in many species, the stem is represented by a rhizome.
- Leaf Morphology:
- Leaves can vary widely in size and form. They may be small and scaly (microphyllous), as in Equisetum, or large and pinnately compound (megaphyllous), as seen in Dryopteris and Adiantum.
- Microphyllous leaves do not form leaf gaps in the vascular supply, while megaphyllous leaves do.
- Vascular Tissue:
- The vascular system, consisting of xylem and phloem, is present in both the stems and roots.
- Xylem contains only tracheids, while the phloem lacks companion cells and true sieve tubes, instead having sieve cells.
- The stele type varies, including protostele (e.g., Rhynia, Lycopodium), siphonostele (e.g., Equisetum), dictyostele (e.g., Adiantum), and polycyclic (e.g., Angiopteris).
- Secondary growth does not occur because cambium is absent.
- Reproduction and Spore Formation:
- Reproduction involves the formation of meiospores through sporic meiosis within sporangia.
- Leaves that bear sporangia are termed sporophylls.
- Most pteridophytes are homosporous, producing only one type of spore (e.g., Pteris, Adiantum). However, some are heterosporous, generating two different spore types: microspores and megaspores (e.g., Selaginella, Salvinia, Marsilea).
- Gametophyte and Sexual Reproduction:
- The gametophyte stage is small and usually independent of the sporophyte.
- Both male and female sex organs are multicellular and protected by a cell layer. Antheridia are small and sessile, while archegonia are partially embedded with a 4-rowed neck.
- The sperm cells are bi-flagellate or multi-flagellate, requiring water for movement to the archegonia for fertilization.
- Embryo and Life Cycle:
- An embryo stage is present in the life cycle.
- Pteridophytes exhibit heteromorphic alternation of generations, where the sporophyte is the dominant generation, while the gametophyte is comparatively short-lived and less prominent.
Habitat of Pteridophytes
Pteridophytes, being the first vascular plants to adapt to life on land, are primarily found in tropical and temperate zones. Although most thrive in terrestrial environments, they have adapted to a wide range of habitats. Below is a detailed overview of the various habitats in which pteridophytes can be found:
- Terrestrial Habitats:
- The majority of pteridophytes, from ancient fossils to modern species, inhabit terrestrial environments. These plants typically prefer moist, shaded areas.
- Examples of species found in terrestrial habitats include Ophioglossum sp., Lycopodium clavatum, Lycopodium cernum, and Equisetum sp.
- Some species demonstrate unique adaptability. For instance, Equisetum debile can grow in hydrophytic, mesophytic, and xerophytic environments, showing a remarkable range of tolerance.
- Other species exhibit more specific habitat preferences, such as Equisetum palustre, which thrives in swampy areas, and Equisetum arvense, which is commonly found in open grasslands.
- Aquatic Habitats:
- A subset of pteridophytes, primarily from the families Marsileaceae and Salviniaceae, have adapted to aquatic or semi-aquatic environments. These are commonly referred to as aquatic ferns.
- Common species in this category include Marsilea minuta and Marsilea quadrifolia, which are frequently found in water or wetlands.
- Xerophytic Habitats:
- Some species of the genus Selaginella are adapted to survive in dry, arid conditions. These xerophytic pteridophytes have evolved to endure harsh environments with limited water availability.
- Examples of xerophytic species include Selaginella wightii, Selaginella repanda, and Selaginella lepidophylla.
Origin of Pteridophytes
The origin of pteridophytes dates back to the Silurian period, with significant diversification occurring during the Lower Devonian. The evolutionary history of pteridophytes is a subject of debate, with two primary theories proposed: one positing an algal origin and the other suggesting that pteridophytes evolved from bryophytes. Each theory presents distinct evolutionary pathways and evidence, making the topic complex yet intriguing for researchers.
- Algal Origin:
- Some scientists propose that pteridophytes originated from algae, although there is no consensus regarding the exact type of ancestral algae. This theory is based on similarities between algae, especially those in the Chlorophyceae class, and pteridophytes. These common features include:
- Thalloid gametophytes.
- Photosynthetic pigments such as chlorophyll a and b, as well as carotenoids.
- Cell walls composed of cellulose.
- Starch as the storage form of food.
- Flagellated sperms.
- The necessity of water for fertilization.
- Phragmoplast formation during cytokinesis, involving a complex network of microtubules and membrane vesicles.
- Lignier’s Hypothesis (1908):
- Lignier suggested that pteridophytes evolved from Chlorophyta, with a dichotomizing parenchymatous thallus. He theorized that the transition from aquatic to terrestrial habitats involved the basal part of the plant entering the soil for anchorage and absorption. The erect parts remained photosynthetic, forming a primitive branching system, as seen in Rhynia.
- Church’s Hypothesis (1919):
- Church proposed a polyphyletic origin of pteridophytes, suggesting that advanced marine seaweeds called Thallasiophyta were the ancestors of both bryophytes and pteridophytes. He theorized that marine planktonic algae became benthic after the upheaval of ocean floors, leading to the formation of Thallasiophyta. The adaptation to terrestrial life then led to the development of land plants with roots, leaves, and vascular systems. However, this hypothesis lacks geological support and faces criticism due to differences in pigmentation between marine algae and early land plants.
- Fritsch’s Hypothesis (1916, 1945):
- Fritsch believed that pteridophytes and bryophytes evolved from a common Chaetophoraceous green algae ancestor. He proposed that heterotrichous algae, with both prostrate and upright systems, gave rise to erect land plants by enhancing the upright portion and reducing the prostrate part.
- Andrews’ Hypothesis (1950, 1959):
- Andrews theorized that fossil marine algae such as Nematothallus, Protosalvinia, and Crocelophyton independently gave rise to various groups of early vascular plants. He cited the morphological diversity in Psilopsida, Lycopsida, Sphenopsida, and Pteropsida as supporting evidence for this polyphyletic origin.
- Mehra’s Hypothesis (1969):
- Mehra proposed that both bryophytes and pteridophytes originated from a hypothetical Proto-archegoniate group, which in turn arose from Chaetophoraceous ancestors. This group led to the evolution of both the Psilophytaceous line and the Lycopodiaceous line.
- Some scientists propose that pteridophytes originated from algae, although there is no consensus regarding the exact type of ancestral algae. This theory is based on similarities between algae, especially those in the Chlorophyceae class, and pteridophytes. These common features include:
- Bryophytic Origin:
- The theory of bryophytic origin of pteridophytes is based on the similarities between early vascular plants and the sporophytes of certain mosses and hornworts. Shared characteristics include:
- Heteromorphic life cycle.
- Multicellular sex organs.
- Motile and flagellated sperms.
- Thalloid gametophytes.
- Water dependence for fertilization.
- Retention of the zygote within the female sex organ and development of the embryo.
- Plant and spore surfaces covered by cutin (cuticle).
- Anthocerotean Theory:
- Proposed by Campbell (1895), this theory argues that the sporophyte of Anthoceros shares many features with early vascular plants, such as rhizoids instead of roots, meristematic tissue for indefinite growth, columella, photosynthetic tissues with stomata, and limited tissue for spore production. Smith (1955) supported this hypothesis, suggesting that the meristematic region shifted from the base to the apex, allowing the dichotomy seen in early vascular plants.
- Strobilar Theory:
- Bower (1894, 1908) posited that pteridophytes evolved from an Anthoceros-like ancestor, with progressive sterilization of sporogenous tissues leading to the formation of well-differentiated sporophytes, as seen in Lycopodium selago. The evolution involved sporogenous tissue becoming superficial, dividing into separate masses, and eventually developing leaves with sporangia at their base.
- Phyton Theory:
- Celekovsky (1901) proposed that the fundamental part of the sporophyte was originally a leaf, with the axis forming later by the fusion of leaf bases.
- Protocorm Theory:
- Treub (1890) hypothesized that the primitive pteridophyte sporophyte resembled a protocorm, an undifferentiated mass. He supported this theory by pointing to the protocorm found in some species of Lycopodium, suggesting that it represented an early transitional stage in the evolution of the sporophyte.
- The theory of bryophytic origin of pteridophytes is based on the similarities between early vascular plants and the sporophytes of certain mosses and hornworts. Shared characteristics include:
- Modern Interpretation:
- Recent molecular and biochemical studies, including the analysis of 5S/16S rRNA sequences and molecular data from plastid, nuclear, and mitochondrial genes, suggest that bryophytes are not the direct ancestors of vascular plants. Instead, both bryophytes and pteridophytes likely evolved from green algal ancestors, possibly from Coleochaete, which is closely related to charophytes. Coleochaete is considered a model for the algal ancestor of land plants, possessing features such as zygote retention, phragmoplast cell division, and lignin presence, which align with those of land plants.
Classification of Pteridophytes
The classification of Pteridophytes represents a critical area of study in plant taxonomy, encompassing a diverse group of vascular plants commonly known for their absence of seeds. Historically, the term “Pteridophyta” was first introduced by Haeckel, leading to a series of systematic classifications that have evolved significantly over time due to advances in paleobotany and botanical research.
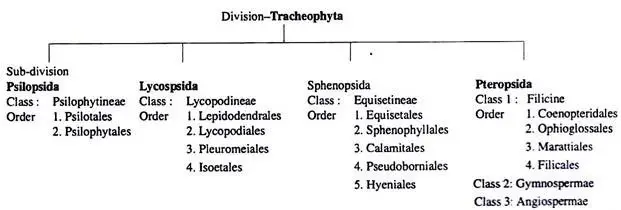
- Historical Classifications:
- Eichler (1883) divided the plant kingdom into two major categories: Cryptogamia (non-seed plants) and Phanerogamia (seed plants). Within Cryptogamia, Pteridophytes were classified along with Thallophyta and Bryophyta.
- Engler (1909) subsequently placed both Bryophyta and Pteridophyta under the umbrella of Embryophyta.
- Evolution of Classification:
- The classification of Pteridophytes has undergone significant changes due to the discovery of fossil plants, particularly seed-bearing forms like Cycadofilicales. This led to the recognition that older taxonomic divisions separating Pteridophyta (seedless vascular plants) from Spermatophyta (seed-bearing plants) were insufficient.
- In light of these developments, Sinnott (1935) introduced the term Tracheophyta to describe a division characterized by sporophytes with well-developed vascular tissues.
- Nomenclatural Standards:
- The International Code of Botanical Nomenclature (I.C.B.N. 1952) established specific suffix conventions for plant classifications, recommending that division names end with “-phyta,” sub-division names with “-phytina,” and class names with “-opsida.”
- Modern Classifications:
- Wardlaw (1955) categorized Pteridophytes into four main divisions based on structural and functional traits:
- Psilopsida: Encompasses both living (e.g., Psilotum) and fossil plants (e.g., Rhynia). These plants exhibit a simple structure, characterized by the absence of roots, with a body organization comprising a subterranean rhizome and an erect aerial shoot bearing homosporous sporangia.
- Lycopsida: This division includes both living (e.g., Lycopodium, Selaginella) and fossil forms (e.g., Lepidodendron). Members possess roots, stems, and microphyllous leaves, with sporangia developing on the adaxial surfaces of sporophylls, and can be classified as homosporous or heterosporous.
- Sphenopsida: This division comprises living species like Equisetum and fossil plants such as Calamophyton. They typically feature long, jointed stems with whorled leaves, and sporangia located at the apex of fertile branches, primarily exhibiting homospory, although some fossil forms are heterosporous.
- Pteropsida: Commonly known as ferns, this division includes approximately 300 genera and over 10,000 species. They thrive in diverse habitats and display a variety of morphological traits, such as large fronds and the presence of sori on leaves, which can be homosporous or heterosporous.
- Wardlaw (1955) categorized Pteridophytes into four main divisions based on structural and functional traits:
- Comprehensive Taxonomic Structures:
- Various taxonomists have contributed to the modern understanding of Pteridophyte classification, with significant contributions from Smith (1955) and Bold (1957). The most accepted structure is:
- Division I: Psilophyta
- Class: Psilotopsida
- Order: Psilophytales
- Class: Psilopsida
- Order: Psilotales
- Class: Psilotopsida
- Division II: Lycophyta
- Class: Eligulopsida
- Order: Lycopodiales
- Class: Ligulopsida
- Orders: Selaginellales, Lepidodendronales, Isoetales, Pleuromeales
- Class: Eligulopsida
- Division III: Sphenophyta or Calamophyta
- Class: Sphenophyllopsida
- Order: Sphenophyllales
- Class: Calamopsida
- Orders: Calamitales, Hyeniales, Equisetales
- Class: Sphenophyllopsida
- Division IV: Pterophyta (Ferns)
- Class: Primofilicopsida
- Orders: Cladoxyales, Coenopteridales
- Class: Eusporangiopsida
- Orders: Ophioglossales, Marattiales
- Class: Protoleptosporangiopsida
- Order: Osmundales
- Class: Leptosporangiopsida
- Orders: Filicales, Marsileales, Salvineales
- Class: Primofilicopsida
- Division I: Psilophyta
- Various taxonomists have contributed to the modern understanding of Pteridophyte classification, with significant contributions from Smith (1955) and Bold (1957). The most accepted structure is:
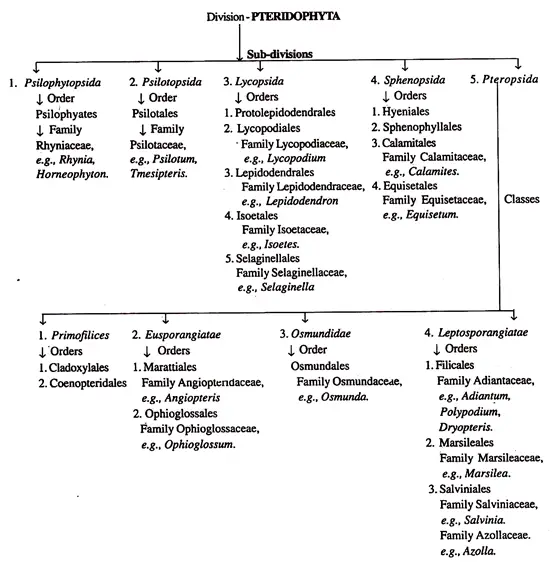
Reproduction of pteridophytes
Reproduction in pteridophytes is a complex process characterized by both vegetative and sexual methods. The primary mode of reproduction in these plants involves the production of spores, yet vegetative reproduction also plays a significant role in their life cycle.
- Vegetative Reproduction:
- Pteridophytes can reproduce vegetatively through several mechanisms:
- Formation of Gemmae or Bulbils: Many pteridophytes can form bulbils or gemmae, which are leafy side branches with a broad base that develop into new sporophytes. When these gemmae fall to the ground, they can germinate and grow into young plants. Examples include Psilotum, Lycopodium phlegmaria, and L. selago. Certain species of Selaginella also propagate using bulbils.
- Fragmentation: This method occurs when older regions of the stem die and decay, leading to the formation of new branches. These branches can develop into separate plants, a common practice among species such as Lycopodium, Selaginella, Dryopteris, Pteris, and Adiantum.
- Formation of Tubers: Tubers may arise from parenchymatous regions of the shoot and root. Surface tubers form at the ground surface, while underground tubers develop below ground. These tubers consist of cells rich in stored food and possess the capacity to germinate into new plants. Some species of Marsilea, Lycopodium renulosum, and Equisetum form such tubers.
- Formation of Adventitious Buds: Adventitious buds can be induced on isolated bulbil leaves or as a result of decapitating the stem near its apex. Species of Lycopodium and Selaginella may develop these buds. In Dryopteris, such buds arise in the leaf axils, detaching to form new plants. Additionally, in certain cases, such as Asplenium esculentum and platycerium, the root apex may develop directly into a leafy bud capable of growing into a new plant.
- Pteridophytes can reproduce vegetatively through several mechanisms:
- Asexual Reproduction:
- The predominant mode of reproduction in pteridophytes is asexual reproduction through haploid spores, which are produced in structures called sporangia. These sporangia may develop either on the ventral surface or within the axils of leaves, with the leaves bearing sporangia referred to as sporophylls. In the Psilophytales, sporangia are found caulinarily, whereas, in some fossil pteridophytes, such as Rhynia, they appear terminal on fine aerial branches. In genera like Azolla, Marsilea, and Salvinia, sporangia are contained within specialized structures called sporocarps, while in higher ferns, sporangia group into organized structures known as sori (singular: sorus).
- According to Goebel’s classification from 1881, sporangia can be categorized into two types:
- Eusporangiate: These develop from a group of initial cells.
- Leptosporangiate: These arise from a single initial cell.
- Sporophyte Generation:
- The sporophyte, the spore-producing body of pteridophytes, is the dominant and most conspicuous phase in their life cycle. Unlike bryophytes, where the gametophytic phase predominates, pteridophytes exhibit a free-living alternation between sporophytic (asexual) and gametophytic (sexual) generations. Although both generations are independent, the sporophyte typically attains a much greater size than the gametophyte.
- Pteridophytes may exhibit two basic types of life cycles:
- Homosporous: These pteridophytes produce only one type of spore, typically leading to the development of a hermaphroditic (monoecious) gametophyte.
- Heterosporous: In contrast, heterosporous pteridophytes generate two kinds of spores—larger megaspores and smaller microspores—leading to the formation of female and male gametophytes, respectively. Heterosporous pteridophytes include species like Selaginella, Isoetes, Marsilea, Salvinia, and Azolla, while homosporous cycles are observed in genera such as Psilotum, Tmesipteris, Lycopodium, Equisetum, and various homosporous Filicopsids.
- Gametophyte Generation:
- The gametophyte represents the sexual phase in the life cycle of pteridophytes. The haploid spores serve as units of the gametophyte, germinating to form a structure known as a prothallus. Typically, prothalli are green, simple, and somewhat branched; however, in some genera such as Lycopodium, they can be subterranean, branched, colorless, and saprophytic.
- Both antheridia (male sex organs) and archegonia (female sex organs) develop on the prothallus. Generally, homosporous pteridophytes produce monoecious prothalli, while heterosporous pteridophytes typically exhibit dioecious prothalli. Antheridia, surrounded by a jacket layer, produce antherozoids, which are unicellular and can be either uniciliate or multiciliate depending on the species. Archegonia consists of a projecting neck and a lower embedded venter containing the egg cell.
- Fertilization and Zygote Formation:
- Fertilization occurs in the presence of water, which facilitates the movement of antherozoids. As a film of water forms between the prothallus and the substratum, cap cells of the antheridia open to release the antherozoids. These are attracted to the archegonia by malic acid released from a mucilaginous mass, allowing them to swim toward the archegonia. Typically, only one antherozoid fuses with an egg cell within the archegonium, resulting in the formation of a diploid zygote, often following cross-fertilization due to the protandrous nature of the prothalli.
- Embryo Development:
- The zygote undergoes development into an embryo, with the first division generally occurring transversely. Subsequent divisions lead to the formation of a young sporophyte, marking the transition from the gametophyte generation back to the dominant sporophyte phase of the life cycle.
Alternation of Generation in pteridophytes
Pteridophytes, a diverse group of vascular plants, exhibit a true alternation of generations, a life cycle involving both a multicellular haploid stage (gametophyte) and a multicellular diploid stage (sporophyte). This process highlights the distinct reproductive strategies that facilitate survival and adaptation. The sporophyte generation is dominant and produces spores through meiosis, while the gametophyte generation forms gametes by mitosis, showcasing the intricate interplay between these two stages.
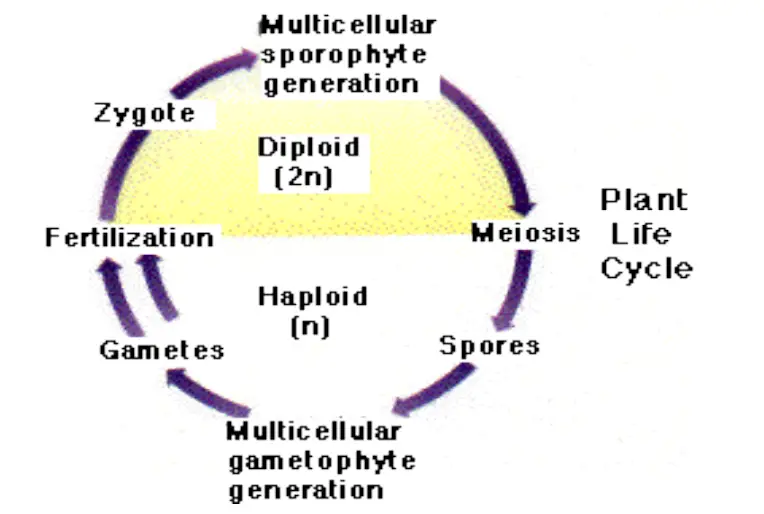
- Sporophyte Generation:
- The mature sporophyte is diploid, possessing two sets of chromosomes (2n).
- It produces haploid spores (n) through the process of meiosis, which reduces the chromosome number by half.
- These spores are vital for the continuation of the life cycle as they germinate and develop into gametophytes.
- Gametophyte Generation:
- The resulting gametophyte is haploid, containing one set of chromosomes (n).
- It matures and produces gametes (sperm and eggs) through mitosis, maintaining the haploid chromosome number.
- When two gametes fuse during fertilization, a diploid zygote forms, which eventually develops into a new sporophyte.
- Cycle of Alternation:
- The sporophyte and gametophyte stages alternate sequentially in the life cycle of pteridophytes.
- This pattern, termed “alternation of generations,” is an essential survival strategy, allowing pteridophytes to switch between sexual and asexual reproduction as environmental conditions change.
- Abnormalities in the Life Cycle:
- While the alternation of generations is typically consistent, it can be disrupted by phenomena known as apogamy and apospory.
- Apogamy:
- This phenomenon involves the development of a sporophyte directly from a gametophyte without any sexual fusion (syngamy).
- The resulting sporophyte retains the haploid chromosome number characteristic of the gametophyte (n).
- Apogamy was first identified by Farlow in 1874 in the species Pteris cretica and is prevalent among various ferns, including Pteris, Pteridium, Dryopteris, Adiantum, Osmunda, Todea, Athyrium, and Asplenium.
- Apospory:
- Apospory refers to the development of gametophytes from vegetative cells of the sporophyte, bypassing meiotic division and the formation of spores.
- This results in gametophytes that are diploid, a deviation from the typical haploid gametophyte.
- The term was first introduced by Druery in 1884, following his observations in Athyrium filix-femina. Subsequently, apospory has been documented in several pteridophytes, such as Pteridium aquilinum, Asplenium dimorphum, Osmunda regalis, and Todea.
The life cycle of pteridophytes
The life cycle of pteridophytes is characterized by a fascinating alternation between two generations: the sporophyte (2n) and the gametophyte (n). This process typically involves a periodic doubling of the chromosome number through syngamy (fertilization), followed by a halving through meiosis. However, deviations from this standard reproductive cycle are observed in some pteridophyte species, highlighting the diversity of reproductive strategies within this group.
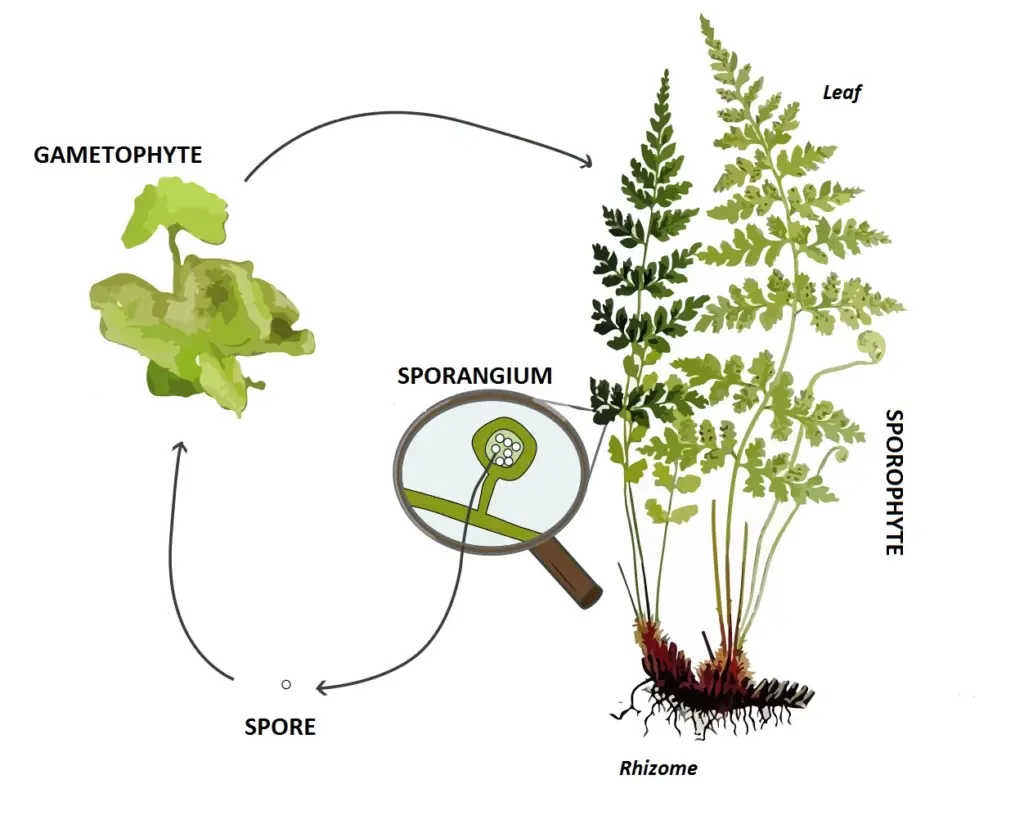
- Sporophyte Generation (2n):
- The dominant generation in pteridophytes is the sporophyte, which is typically diploid.
- It produces spores through meiosis in specialized structures called sporangia.
- These spores are haploid (n) and can develop into the gametophyte generation.
- Gametophyte Generation (n):
- The gametophyte is a free-living, haploid stage that arises from the germination of spores.
- It produces gametes (sperm and eggs) through mitosis in structures known as gametangia.
- The fusion of gametes during syngamy leads to the formation of a new diploid sporophyte.
- Variations in Reproductive Strategies:
- Parthenogenesis: In certain ferns, such as Marsilea, Cyathea, and Athyrium, the embryo can develop from an unfertilized egg. This phenomenon, known as parthenogenesis or “virgin birth,” allows for reproduction without the need for fertilization.
- Apospory:
- Apospory occurs when gametophytes develop directly from vegetative cells of the sporophyte, bypassing the haploid spore stage entirely.
- In this case, cytological studies have revealed that the resulting gametes are diploid. Consequently, when these gametes fuse, the resulting sporophyte is tetraploid, as seen in species like Osmunda regalis and Pteris vittata.
- Apogamy:
- Apogamy is another deviation wherein a sporophyte forms without fertilization, arising from the vegetative cells of the gametophyte.
- The chromosome number of the apogamous sporophyte is determined by that of the gametophyte, which can be either haploid or diploid. Examples include ferns such as Pteridium, Cheilanthes, and Pteris.
Stelar System in Pteridophytes
The stelar system in pteridophytes is a vital aspect of their anatomy and function, serving as a fundamental component of their vascular systems. The term “stele” is derived from the Greek word meaning “column” or “pillar,” reflecting its role as a central structural element. The concept of the stele as the primary unit of vascular organization was first proposed by van Tieghem and Douliot in 1886, who identified the stele as a central cylinder surrounded by the cortex, with the endodermis serving as the anatomical boundary between these two regions. This central cylinder typically comprises various vascular tissues, including xylem, phloem, pericycle, and sometimes pith or medullary rays.
- Formation and Types of Steles: In lycopsids, such as Lycopodium and Selaginella, the development of the stele occurs independently from the leaf traces, resulting in a cauline primary vascular system. The leaf traces in these plants are generally small and connected superficially to the vascular cylinder. In contrast, the stelar anatomy in ferns like Pteridium and Pteris is influenced by larger leaf traces and leaf gaps, leading to a composite structure that incorporates both cauline and foliar vascular components.
- Classification of Steles: Researchers have identified two principal types of stelar organization in pteridophytes: Protostele and Siphonostele.
- Protostele: Characterized by the absence of a central pith, protosteles are considered the most primitive form of stelar organization. They consist of a central strand of primary xylem surrounded by a cylinder of phloem. Protosteles are found in early vascular plants such as Rhynia, Horneophyton, and living representatives like Psilotum and Selaginella. Various forms of protostele include:
- Haplostele: A simple, solid core of xylem surrounded by phloem, seen in plants such as Tmesipteris and Selaginella.
- Actinostele: Features a lobed, star-shaped xylem core with phloem situated in groups between the lobes, found in species like Psilotum.
- Plectostele: Characterized by xylem breaking into several parallel plates, each encircled by phloem, seen in Lycopodium species.
- Mixed Protostele: Displays small groups of tracheids embedded in phloem, observed in Lycopodium cernuum.
- Siphonostele: This stele features a central column of parenchymatous pith, representing an advancement over protostele. In siphonosteles, the vascular tissues are arranged in a cylindrical fashion, with distinct pith at the center. Siphonostele can be classified into two main types based on phloem positioning:
- Ectophloic Siphonostele: Contains a single ring of phloem external to the xylem core, as seen in Equisetum and Osmunda.
- Amphiphloic Siphonostele: Features phloem surrounding the xylem on both sides, with two endodermal layers, observed in ferns like Marsilea and Dryopteris.
- Protostele: Characterized by the absence of a central pith, protosteles are considered the most primitive form of stelar organization. They consist of a central strand of primary xylem surrounded by a cylinder of phloem. Protosteles are found in early vascular plants such as Rhynia, Horneophyton, and living representatives like Psilotum and Selaginella. Various forms of protostele include:
- Further Classifications of Siphonosteles: Siphonosteles can also be divided based on the presence or absence of leaf and branch traces:
- Cladosiphonic: Characterized by the absence of leaf traces, found in lycopsids.
- Phyllosiphonic: Features both leaf and branch traces, as seen in members of the order Filicales.
- Evolutionary Aspects of Siphonostele: Based on the arrangement of leaf gaps, siphonosteles can be categorized into:
- Solenostele: Exhibits non-overlapping leaf gaps, with only one break in the vascular cylinder at any given point.
- Dictyostele: Characterized by overlapping leaf gaps, resulting in a highly dissected vascular structure, commonly found in ferns where the rhizome is compacted with leaves.
- Polycyclic Stele: A complex form of siphonostele with multiple concentric vascular cylinders, observed in species like Pteridium aquilinum and Montania peotinata. This type can further be classified into polycyclic solenosteles and polycyclic dictyosteles based on the outer cylinder’s characteristics.
Economic Importance of Pteridophytes
Pteridophytes, often overlooked in discussions about economic plants, hold significant importance across various sectors, particularly in food, medicine, horticulture, and environmental applications. Despite their limited documentation, the diverse uses of pteridophytes contribute to human welfare in several meaningful ways.
- Pteridophytes Used as Food:
- Young leaf tips of ferns, known as circinate ptyxis or croziers, are consumed as vegetables.
- For example, the young fronds of Ampelopteris prolifera are marketed as ‘dheki shaak’ in India and Bangladesh.
- Canned or frozen croziers of Matteuccia struthiopteris are enjoyed as spring vegetables in the USA and Canada.
- Additionally, the leaves of Marsilea, commonly referred to as ‘shushni’, serve as a vegetable.
- The rhizomes of many ferns, such as Pteris, are rich in starch and are utilized as food.
- The corms of Isoetes are also used as food for pigs, ducks, and other animals.
- Pteridophytes Used as Fodder:
- Dry fronds of various ferns provide livestock feed.
- The quadrifid lamina of Marsilea, which resembles clover (Trifolium), is utilized as animal fodder, serving as a substitute for traditional clover.
- Pteridophytes Used as Medicine:
- The spores of Lycopodium are extensively used in the pharmaceutical industry as protective dusting powder and water-repellents for sensitive skin.
- The foliage of Lycopodium is prepared as tinctures, powders, ointments, and creams for various medicinal purposes, including as a stomachic and diuretic.
- Decoctions of Lycopodium are applied in homeopathy to treat conditions such as diarrhea, bladder irritability, eczema, rheumatism, constipation, and liver inflammation.
- Equisetum is noted for its high content of silicic acid and silicates, alongside potassium, aluminum, manganese, and flavonoids, which contribute to its diuretic and connective tissue-strengthening properties.
- Several ferns serve as herbal remedies; for instance, oil extracted from the rhizome of Aspidium is used as a vermifuge, particularly against tapeworms.
- Decoctions from Asplenium are employed for cough relief, while extracts from Polypodium act as mild laxatives.
- The root decoction of Osmunda regalis is traditionally used for treating jaundice, and its root is applied topically to wounds.
- Additionally, the extraction of Osmanda vulgaris, known as ‘Green oil charity’, is effective for wound treatment.
- Pteridophytes Used as Horticultural Plants:
- Many species of pteridophytes are cultivated for their aesthetic appeal.
- For instance, variants of Psilotum are commonly grown in nurseries and greenhouses as ‘whisk fern’.
- Epiphytic species of Lycopodium, such as L. phlegmaria and L. lucidulum, are prized for their aesthetic value and are suitable for hanging baskets.
- Various species of Selaginella serve as ground cover, while the decorative qualities of species like Salaginella willdenovii and S. uncinata attract gardeners for their striking blue color.
- Ferns like Angiopteris, Asplenium, Marattia, Microsorium, and Nephrolepis are valued for their graceful shapes and beautiful soral arrangements.
- Pteridophytes Used as Biofertilizers:
- Azolla, a rapidly multiplying water fern, plays a crucial role in agriculture.
- It houses live colonies of the nitrogen-fixing cyanobacterium Anabaena azollae, creating a symbiotic relationship where the alga supplies nitrogen to the plant.
- Farmers utilize Azolla as a green manure in rice paddies, enhancing crop production.
- Pteridophytes Used as Indicator Plants:
- Certain pteridophytes serve as indicator plants for mineral deposits.
- For example, Equisetum can accumulate minerals, including gold, at rates up to 4.5 ounces per ton, making it a valuable resource in the search for gold ore deposits.
- Other species, such as Asplenium adulterinum and Actinopteris australis, indicate the presence of nickel and cobalt, respectively.
- Pteridophytes Used for Various Purposes:
- Pteridophytes have a range of applications beyond food and medicine.
- The stem of Equisetum has historical use for polishing wood and cleaning utensils.
- Roots and stems of Osmunda are utilized in making orchid beds.
- Water boiled with Lycopodium clavatum is used for dyeing wool, providing a blue hue when treated with Brazil wood.
- Lycopodium powder is also highly flammable, making it useful in pyrotechnics and artificial lighting demonstrations.
Ecological Importance of Pteridophytes
Pteridophytes, commonly known as ferns and their allies, play a vital role in ecosystems due to their unique characteristics and adaptability. Their ecological importance can be outlined as follows:
- Habitat Formation:
- Pteridophytes often thrive in diverse habitats, ranging from tropical rainforests to temperate woodlands and even arid regions. They contribute significantly to habitat structure, providing shelter and nesting sites for various organisms.
- Soil Stabilization:
- The extensive root systems of pteridophytes help prevent soil erosion, especially on slopes and in areas prone to landslides. Their ability to form dense mats of foliage can stabilize soil, maintaining soil quality and preventing loss of topsoil.
- Biodiversity Support:
- Pteridophytes contribute to biodiversity by offering habitats for numerous species, including insects, amphibians, and small mammals. They serve as a food source for herbivorous animals and as a substrate for various microorganisms.
- Nutrient Cycling:
- These plants are essential in nutrient cycling. As pteridophytes grow and die, they decompose, enriching the soil with organic matter and nutrients. This process enhances soil fertility, supporting a diverse range of plant species.
- Water Regulation:
- Pteridophytes play a crucial role in the water cycle. They can absorb and retain moisture in their leaves and surrounding soil, contributing to local humidity levels and influencing microclimates. Their transpiration helps maintain water balance in ecosystems.
- Medicinal Uses:
- Many pteridophytes have been utilized in traditional medicine for their therapeutic properties. Their bioactive compounds are being researched for potential applications in pharmaceuticals, highlighting their value beyond ecological contributions.
- Carbon Sequestration:
- By capturing carbon dioxide during photosynthesis, pteridophytes contribute to carbon sequestration, playing a role in mitigating climate change. Their capacity to store carbon in their biomass aids in reducing greenhouse gas concentrations in the atmosphere.
- Cultural and Aesthetic Value:
- In many cultures, pteridophytes are valued for their aesthetic appeal and are commonly used in landscaping and gardening. Their presence can enhance the beauty of natural environments, promoting ecotourism and conservation efforts.
- https://www.biologydiscussion.com/plants/pteridophytes-meaning-origin-and-economic-importance/46096
- https://www.geeksforgeeks.org/pteridophytes/
- https://plantlet.org/classification-of-pteridophytes/
- https://www.biologydiscussion.com/pteridophytes/classification-pteridophytes/classification-of-pteridophytes-botany/52998
- https://en.wikipedia.org/wiki/Pteridophyte
- https://www.jsscacs.edu.in/sites/default/files/Department%20Files/AN-%20HC%201.2-%20PTERIDOPHYTES_compressed%20%282%29_compressed.pdf
- https://plantlet.org/origin-of-pteridophytes/#google_vignette