Population, a term derived from the Latin word populus meaning “people,” refers to a group of organisms belonging to the same species that live in a specific geographical area and have the potential to interbreed. In ecological terms, a population is an assemblage of individuals of a species that occupy a particular space at a given time. For example, one can refer to the population of grasshoppers in a field, deer in a forest, or pine trees in a woodland. These organisms, although often in proximity, may exhibit different social structures, such as living solitarily or interacting at different stages of life.
Ecologist J. M. Clarke, in 1954, categorized populations into two primary types. A monospecific population consists of individuals that all belong to the same species, whereas a polyspecific or mixed population includes individuals from different species. The term “community” is frequently used to describe polyspecific populations, as it refers to the interactions between various species within a given habitat.
Populations are often organized into smaller, local groups known as demes, which are the smallest reproductive units capable of interbreeding. These demes share a common gene pool, and gene flow between them occurs through migration—both emigration and immigration. This movement between subpopulations can contribute to genetic variation within and among populations. When such genetic differences arise between subpopulations of the same species, it is termed genetic differentiation.
Natural selection, a key evolutionary process, operates on individuals within a population and results in changes over generations. Evolution, in this context, refers to alterations in the properties of populations over time, driven by changes in gene frequencies. Specifically, phenotypic evolution involves shifts in the average characteristics of a population due to variations in allele frequencies. Natural selection directly acts on phenotypes, favoring traits that enhance an individual’s fitness in a particular environment. As a result, allele frequencies within a population shift from one generation to the next, reflecting the differential survival and reproduction of individuals based on their traits. Thus, the changes in allele frequencies from one generation to the next can lead to long-term evolutionary changes in the population.
Population Characteristics
- Population density
- Methods of measuring population density
- Natality
- Types of natality
- Mortality
- Types of mortality
- Age structure
- Age pyramids
- Sex ratio
1. Population density
Population density is a fundamental characteristic of populations, defined as the number of individuals residing in a specific unit area or volume. This measure is crucial for understanding the dynamics of various species in their habitats and varies significantly based on several ecological factors.
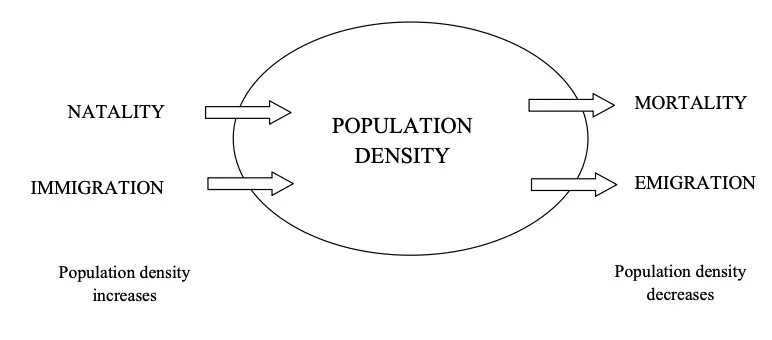
- Units of Measurement: Population density is expressed in various units depending on the context. Common examples include the number of squirrels per square kilometer, earthworms per acre, or paramecia per liter of water. This variability underscores the necessity of choosing appropriate units tailored to the specific population being studied.
- Variability and Limits: Population density is influenced by seasonal changes, availability of food resources, and climatic conditions. Although the lower limit of population density is not well-defined, homeostatic mechanisms often regulate densities to avoid extreme fluctuations. Conversely, the upper limit of density is influenced by factors such as the organism’s size and its trophic level. For instance, smaller organisms, such as insects, tend to have higher densities compared to larger animals like deer, which exhibit lower densities due to their size and habitat requirements.
- Determinants of Population Density: Several factors play a significant role in shaping population density. These include:
- Resource Availability: The abundance of food, water, and shelter directly affects how many individuals can survive in a given area.
- Productivity: The productivity of the ecosystem influences population growth and sustainability, leading to fluctuations in density.
- Energy Flow: Energy transfer within an ecosystem, from primary producers to various trophic levels, impacts population sizes.
- Physiological Stress: Environmental stressors can limit growth and reproduction, thus affecting population density.
- Dispersal Patterns: Movement of individuals between populations can lead to changes in density as organisms migrate to more suitable habitats.
- Types of Population Density: Population density can be categorized into two primary types:
- Crude Density: This measurement refers to the total number of individuals within a total area. For example, calculating the number of rhinoceroses in Kaziranga National Park provides a crude density measure. However, this method may lead to inaccuracies, as individuals are not uniformly distributed, and some areas may be unsuitable for habitation.
- Ecological Density: In contrast, ecological density accounts for the number of individuals per unit of available living space, focusing on the habitats actually occupied by the species. For instance, rhinoceroses in Kaziranga do not inhabit all areas of the park due to factors like food availability, leading to a higher ecological density in the regions they occupy.
- Measuring Population Density: Various methods are employed to measure population density effectively:
- Total Counts: This straightforward approach involves counting all organisms within a specific area, suitable for large or easily observable species, such as humans or whales.
- Sampling Methods:
- Quadrat Sampling: In this method, researchers count individuals within several quadrats of known size. The quadrats can be circular, triangular, or square, depending on the organism being studied. This method is particularly useful for estimating plant populations and invertebrates.
- Capture-Recapture Method: Developed by C.G.J. Peterson in 1898, this technique involves capturing, marking, and releasing animals, followed by recapturing them to estimate population size. For example, if 100 fish are tagged and released, and later 120 fish are recaptured (including 30 marked individuals), the total population can be estimated using the formula:

- Relative Density: In some cases, determining absolute density is impractical, so researchers may instead assess relative density, which serves as an abundance index. This can be measured using various methods:
- Traps: Counting individuals caught per day per trap.
- Fecal Pellet Counts: Evaluating the number of fecal pellets from species like rabbits or deer can indicate population size.
- Vocalization Frequency: Monitoring the frequency of calls, such as pheasant calls, within a designated time period can help estimate population density.
- Feeding Capacity: Measuring the amount of food consumed by animals before and after treatment provides insights into population changes, as seen in studies of rat or mouse densities.
2. Natality
Natality refers to the process by which new individuals are added to a population through birth, hatching, or other reproductive mechanisms such as fission or germination. It is a key factor in understanding population growth and is essential for determining the dynamics within any given population. Natality is often measured as the number of offspring produced per unit of time within a population.
- Types of Natality: Natality can be categorized into two distinct types:
- Absolute or Physiological Natality:
- This represents the theoretical maximum number of offspring that a population can produce under ideal conditions. Absolute natality is a measure of the organism’s inherent reproductive potential when no limiting factors—such as food shortages, predation, or environmental stress—are present.
- Absolute natality is rarely observed in natural populations because ideal conditions seldom persist in the wild. However, under controlled laboratory conditions or favorable environmental circumstances, organisms may exhibit their physiological maximum reproduction rate.
- An example of absolute natality can be observed in paramecia. When placed in a nutrient-rich culture medium, they multiply at their highest reproductive rate, reflecting their maximum reproductive capacity.
- Absolute natality is significant because it serves as a baseline for comparison with realized natality and helps establish mathematical models to predict population growth.
- Realized or Ecological Natality:
- Unlike absolute natality, realized natality reflects the actual rate of population increase under typical environmental conditions, including factors like availability of resources, predation, and habitat conditions.
- Realized natality is not constant for a given population; it varies over time depending on the age structure, size, composition, and physical conditions of the environment. This type of natality is more reflective of the actual dynamics within a population.
- Realized natality is often expressed as the number of new individuals added to a population over time per unit population. The formula used to express natality is:
- Absolute or Physiological Natality:
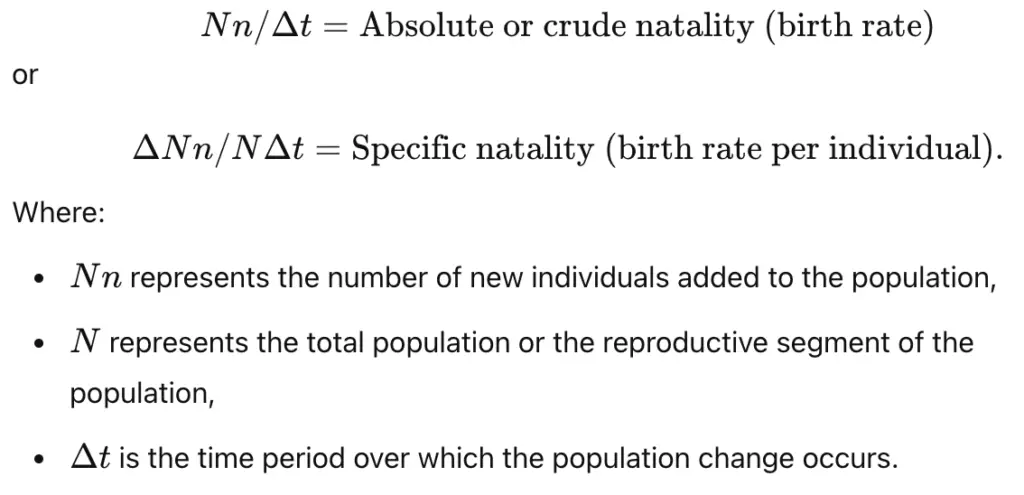
- Factors Affecting Natality:
- The natality rate is influenced by a variety of factors, particularly the number of females in the population, as reproduction typically occurs through female organisms. Consequently, the age-specific birth schedule is often used to estimate the reproductive output of a population, counting only the females and the offspring they produce.
- The birth rate varies with the species. For instance, fish may lay thousands of eggs, frogs produce hundreds, birds generally lay between 1 to 20 eggs, and mammals typically have fewer offspring, ranging from 1 to 10. This variation can be linked to the reproductive strategy of the species and is often inversely correlated with the level of parental care.
- Crude and Specific Birth Rate:
- Crude Natality (Birth Rate): This is the simplest form of natality, calculated by dividing the number of births in a population by the total population size over a given period. For instance, if a population of 1,000 individuals experiences 40 births in a year, the crude natality rate would be expressed as 40 births per 1,000 individuals, or 4% birth rate.
- Specific Natality: This measure refers to the number of offspring produced per female during a given time period and is particularly useful for assessing reproductive output in species with varied life history traits. Specific natality is often influenced by factors such as the availability of food, environmental conditions, and the organism’s age structure.
- Parental Care and Birth Rate:
- The level of parental care in a species is generally inversely related to its birth rate. Species that produce fewer offspring typically invest more energy in the care and survival of each individual offspring. In contrast, species that produce large numbers of offspring may invest less in each individual and provide little to no parental care.
- This relationship highlights the diversity in reproductive strategies, where the investment in offspring survival varies according to species-specific life history traits.
3. Mortality
Mortality refers to the rate at which individuals in a population die, a key factor influencing population dynamics. It provides insight into the health, age structure, and survival conditions within a population. Understanding mortality helps explain fluctuations in population size and provides critical information about environmental pressures, such as predation, disease, and resource availability.
- Types of Mortality:
- Ecological or Realized Mortality:
- This type of mortality represents the actual death rate of individuals under current environmental conditions. It is not constant and fluctuates with changes in the environment and the population’s interactions with its surroundings, including predation, disease outbreaks, and competition for resources.
- Ecological mortality is a reflection of real-world conditions where individuals face multiple threats such as exposure to predators, inadequate food supply, and environmental stress. These factors contribute to variations in mortality rates within populations.
- For example, a population of roundworms might experience higher mortality rates if they are exposed to a pathogenic parasite or if the environment becomes unfavorable for their survival.
- Theoretical or Minimum Mortality:
- Minimum mortality is the theoretical death rate under ideal, non-limiting conditions. It represents the number of deaths that would occur due to aging or senescence, assuming no external pressures, such as predation or disease, are present.
- This type of mortality assumes that conditions are optimal for the population, with no resource limitations or environmental stressors. The individuals would die naturally from old age, and the mortality rate would be constant.
- Minimum mortality is always greater than the ecological longevity (the maximum age an organism can reach in the wild under ideal conditions). Therefore, while organisms in nature may experience some aging, they are more likely to die due to external pressures before they reach old age.
- Ecological or Realized Mortality:
- Measuring Mortality:
- Mortality is often expressed as a death rate, which is the total number of individuals that die within a population during a specific time period. This rate is typically calculated per thousand individuals for consistency and ease of comparison.
- To calculate the death rate, the number of individuals that die in a given period is divided by the average population size during that period. For instance, if a population of 500 individuals is reduced to 400 after a certain time, the average population size over that period would be 450. The death rate would then be calculated as:

This indicates that 22% of the population died during the observed period.
- Probability of Death and Survival:
- The probability of death, or the likelihood that an individual will die during a given period, can be calculated by dividing the number of individuals that died by the total population at the start of the period. For example, if 100 individuals died out of an initial population of 500, the probability of death would be:

- The complement of this probability, known as the probability of survival, is calculated by dividing the number of survivors by the initial population size. In this example, 400 individuals survived, so the probability of survival is:

- Life Expectancy:
- Life expectancy is closely related to mortality rates and is used to predict the average number of years an individual of a certain age can expect to live in a population. It is a measure of future survival and is often calculated for a specific age group within a population.
- Mortality and life expectancy are inversely related; higher mortality rates generally result in lower life expectancy. Understanding these relationships can provide valuable information about the overall health and sustainability of a population. Life expectancy is a useful tool for scientists when evaluating the impact of environmental or ecological changes on a population’s survival rates.
4. Age structure
Age structure refers to the distribution of individuals across different age groups within a population. It plays a crucial role in determining the overall dynamics and future trends of that population. Age structure can be visually represented through age pyramids or polygons, which offer a snapshot of the relative sizes of various age groups in a population at a given moment in time. These pyramids are constructed by plotting the number of individuals or the percentage of individuals within each age class, with the width of each horizontal bar corresponding to the population size in that age category.
- Types of Age Pyramids:
- Bell-shaped Pyramid (Expanding Population):
- A bell-shaped pyramid with a broad base is indicative of an expanding population. In this structure, the largest number of individuals are found in the younger age classes. This suggests that the birth rate is high, and there are more individuals entering the reproductive phase than those exiting it.
- Expanding populations tend to exhibit rapid growth, often following an exponential growth pattern. This can be observed in species like Paramecium, bacteria, and yeast, which can reproduce rapidly under favorable conditions. In these populations, resources such as light, water, and nutrients are often readily available to support the increasing number of young individuals.
- Dome-shaped Pyramid (Stable Population):
- A dome-shaped pyramid reflects a more balanced population structure, where the proportion of individuals in the pre-reproductive, reproductive, and post-reproductive age groups are relatively equal. In this scenario, the reproductive and pre-reproductive phases are almost equal in number, with the post-reproductive phase being the smallest.
- A stable population is generally maintained when natality (birth rate) and mortality (death rate) are in balance. While the population may not grow or decline drastically, the age structure ensures a steady flow of individuals through the reproductive cycle, allowing for a sustainable population size over time.
- Urn-shaped Pyramid (Declining Population):
- An urn-shaped pyramid represents a declining population, where the pre-reproductive age group is much smaller than the reproductive and post-reproductive age groups. This structure indicates that the birth rate has declined drastically, resulting in a smaller number of individuals entering the reproductive phase.
- In such a population, the overall trend is towards a decline in population size, as fewer young individuals are born to replace the older, post-reproductive individuals. This can be seen in species or populations that are facing serious environmental stressors, such as habitat loss or other factors that severely reduce reproductive success.
- Bell-shaped Pyramid (Expanding Population):
- Age Structure and Population Dynamics:
- The age structure of a population is largely shaped by the age-specific patterns of mortality (death rate) and reproduction. These patterns influence how individuals progress through different age categories, impacting the overall population growth or decline.
- When a significant proportion of older individuals die or are removed from the population, it can open up resources such as light, water, and nutrients for the younger age groups. This can facilitate the growth and development of these younger individuals, thus promoting population growth or recovery.
- Age Distribution and Wildlife Management:
- In wildlife management, understanding the age distribution of a population can be a key factor in making decisions about conservation and resource allocation. For instance, if a population shows a low ratio of pre-reproductive to reproductive individuals, this may indicate poor breeding success or a declining reproductive season.
- However, age distribution alone cannot fully explain population trends, as it does not directly account for factors such as survivorship or fecundity (reproductive success). Age distribution is an important piece of the puzzle, but it should be considered alongside other biological and ecological factors to predict the long-term viability of a population.
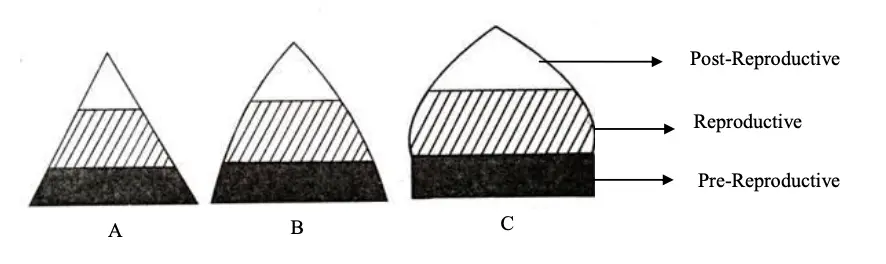
5. Sex ratio
Sex ratio refers to the proportion of male and female individuals within a given population, and it is an important demographic measure that can provide insights into the reproductive dynamics of sexually reproducing organisms. While sex ratio is typically considered in the context of sexually reproducing species, it is also relevant to some asexual organisms that may have the capacity for sexual reproduction to maintain genetic diversity within the population. Sex ratio can be classified into two distinct categories: the primary sex ratio and the secondary sex ratio.
- Primary Sex Ratio:
- The primary sex ratio represents the ratio of male to female individuals at the moment of conception. In many species, particularly those that reproduce sexually, the primary sex ratio tends to approach 1:1, meaning an equal number of males and females are conceived. This balance is believed to be an adaptive strategy to maximize reproductive success, although it is difficult to confirm universally due to the inherent challenges in measuring sex at conception.
- While many organisms exhibit a primary sex ratio of close to 1:1, some variations can occur depending on environmental factors, genetic influences, or reproductive strategies.
- Secondary Sex Ratio:
- The secondary sex ratio refers to the ratio of males to females at birth. In many sexually reproducing species, this ratio is often biased towards males, with males being more numerous at birth. However, as individuals age, the sex ratio shifts toward females, particularly in species with significant differences in lifespan between males and females.
- In mammals, including humans, the secondary sex ratio is often slightly biased towards males, with approximately 52% of births resulting in male offspring. However, as individuals age, the population tends to become more female-dominated due to the generally shorter lifespan of males. This disparity can be attributed to both physiological factors, such as differences in longevity, and behavioral factors, like male competition for mates or dominance in social hierarchies. In some species, males face higher mortality rates due to intense competition for breeding opportunities or higher vulnerability to predation.
- Variation in Sex Ratios Across Species:
- Some species exhibit significant variation in their secondary sex ratio due to environmental or behavioral factors. For instance, in the case of the Alaskan fur seal, male individuals take over breeding groups, resulting in a skewed male-to-female ratio that can exceed 3:1. These males experience higher mortality rates due to their peripheral location in the breeding groups, which increases their vulnerability to predation.
- Additionally, among birds, males may become more numerous due to the higher mortality rate of nesting females. Female birds often expend more energy during reproduction and may be more susceptible to predation during this period.
- Mating Systems and Their Impact on Sex Ratio:
- In sexually mature animals, the choice of mates is influenced by the mating system, which plays a key role in shaping the reproductive behavior of individuals and subsequently impacts sex ratios within populations. The main mating systems include monogamy, promiscuity, and polygamy.
- Monogamy:
- In monogamous species, males and females form a long-term pair bond, which is typically maintained for the duration of the breeding season or even for life. Monogamy is most commonly observed in birds and some mammals, where both parents actively cooperate in caring for their young. For instance, in species like bluebirds (Sialia sialis), the pair may even “cheat” by mating with others while maintaining their primary reproductive relationship. This behavior can help ensure the inclusion of additional genetic material in the gene pool.
- Polygamy:
- Polygamy refers to a system in which individuals acquire two or more mates. This can take two primary forms: polygyny, where one male pairs with multiple females, and polyandry, where one female pairs with multiple males. Polygyny is more common than polyandry and is observed in many species, including some mammals and birds. In contrast, polyandry is less frequent but well-developed in certain bird species, such as jacanas, phalaropes, and sandpipers. In polyandry, the female often competes for resources and defends her territory, while males incubate eggs and care for the offspring without direct involvement in the mating process.
Dispersal
Dispersal is a critical ecological process that allows organisms or their reproductive products to move across a landscape or seascape. It plays a fundamental role in shaping the distribution of species, gene flow, and the establishment of new populations. Dispersal is necessary for the colonization of new areas, which can, in turn, lead to changes in species composition or even the formation of new species due to environmental adaptations.
- Dispersal Mechanisms:
- Organisms employ various mechanisms to disperse across their habitats, depending on their mobility and environmental conditions.
- In plants, seeds and spores are often dispersed through wind, water, or by animals. Heavier seeds, such as the acorns of oak trees (Quercus spp.), typically disperse over shorter distances. In contrast, lighter seeds like those of maples (Acer spp.), milkweed (Asclepiadaceae), and dandelions (Taraxacum officinale) are carried over much longer distances by the wind. Additionally, some plants rely on animals for dispersal. For example, cherries and viburnums (Viburnum spp.) are dispersed by birds and mammals that eat the fruits and later deposit the seeds through their feces. Seeds of other plants have evolved adaptations like spines or hooks that attach to the fur of mammals, feathers of birds, or even human clothing, ensuring their transport to new locations.
- In animals, dispersal often involves the movement of fertilized eggs or offspring, particularly in aquatic environments. For example, many aquatic species rely on currents to move their larval forms to suitable habitats. Wind and water are common passive dispersal agents for mobile animals such as spiders, gypsy moth larvae, and brine shrimp cysts (Artemia salina). In some cases, animal dispersal may be facilitated by human activities, which have contributed to the global redistribution of species.
- Types of Dispersal:
- Dispersal can be categorized into several types based on the direction and periodicity of movement.
- Emigration: This refers to the outward movement of organisms from a population. Emigration typically occurs due to factors such as overpopulation, lack of resources, or unfavorable environmental conditions. For example, some birds may leave their original population to find better breeding grounds or food supplies elsewhere.
- Immigration: In contrast to emigration, immigration involves the movement of organisms into a new area. This process is essential for the survival and reproductive success of the immigrants. Immigration often enhances population growth in the new area by introducing fresh genetic material and resources.
- Migration: Migration is characterized by periodic, often seasonal, movements between habitats, usually for breeding or feeding purposes. Migration can occur over short distances, like the daily vertical migration of zooplankton, or over long distances, such as the seasonal movements of birds. For example, many birds in the Northern Hemisphere migrate northward during spring to breed and return south in autumn. Salmon, known for their anadromous migration, travel from freshwater rivers to the ocean to breed and then return to the river to spawn.
- Nomadism: Unlike other forms of dispersal, nomadism involves random, non-seasonal movement in search of resources, without a clear return to the original location. Some human tribes and even certain animal species exhibit nomadic behaviors, moving continuously from place to place.
- Dispersal can be categorized into several types based on the direction and periodicity of movement.
- Dispersal and Population Dynamics:
- Dispersal influences population dynamics by allowing individuals to escape crowded or resource-depleted environments, thus contributing to the spread and genetic diversity of the population. However, dispersal also depends on the interaction between an organism’s mobility and external barriers like geographic features or unfavorable environmental conditions.
- When populations are balanced by limiting factors, immigration and emigration have little effect on overall population growth. However, if a population exceeds its carrying capacity, immigration can prevent population extinction, while emigration may reduce overcrowding. Dispersal can have a significant impact on population structure. For example, when individuals of the Bluegill fish population were introduced into a pond already at carrying capacity, their introduction resulted in a reduction in the average size of individuals, despite no change in total biomass.
- Dispersal and Speciation:
- Dispersal is a key element in the process of speciation, particularly when populations are isolated and adapt to new environments. As individuals move into new regions, they encounter different environmental conditions that may drive evolutionary changes, eventually leading to the formation of new species.
- The process of gene flow is closely tied to dispersal. As individuals or their reproductive products move to new areas, they can interbreed with populations in those areas, leading to increased genetic diversity. This genetic interchange can help maintain population stability or facilitate evolutionary changes.
- Human Influence on Dispersal:
- In recent centuries, humans have become a dominant force in dispersing species across the globe. Human activities such as trade, transportation, and the introduction of species for agricultural or ornamental purposes have significantly altered natural dispersal patterns. In some cases, this has led to the introduction of invasive species that disrupt local ecosystems.
Dispersion
Dispersion refers to the spatial and temporal distribution of organisms within a population. The pattern of how individuals are spread across an environment plays a significant role in shaping population dynamics and interactions. This distribution can occur in various ways, depending on environmental conditions and the behaviors of the organisms involved. There are two primary forms of dispersion: spatial dispersion and temporal dispersion.
- Spatial Dispersion:
- Spatial dispersion refers to the pattern in which individuals of a population are arranged in space. There are three primary types of spatial dispersion:
- Random Distribution:
- In this type, individuals are distributed without any predictable pattern across the landscape. Each individual has an equal chance of being found anywhere in the environment. Random distribution typically occurs in areas where resources are uniformly available throughout the year, and there is no significant competition or attraction between individuals.
- Example: The distribution of clams (Mulinia lateralis) on intertidal mudflats in northeastern North America and some spiders on forest floors exhibit random distribution. This type is rare in nature, as most environments have some form of resource heterogeneity or competition.
- Uniform Distribution:
- In uniform distribution, individuals are spaced evenly, often due to competition for resources. This type of distribution suggests that each organism is trying to maximize its access to limited resources by evenly spacing itself out.
- Example: Desert bushes and forest plants often show uniform distribution due to competition for water and light, respectively. Territorial animals such as carnivorous birds also exhibit uniform spacing as they maintain individual territories.
- Clumped (Aggregated) Distribution:
- Clumped distribution, also known as aggregated distribution, is the most common type. Individuals are found in groups or clusters, often due to the presence of favorable resources, social behavior, or reproductive patterns that encourage group formation.
- Example: Human populations often follow clumped distribution patterns, influenced by factors such as economic opportunities, geographic conditions, and social behavior. Aggregation of plants, such as ferns, often occurs due to vegetative propagation.
- Clumped distribution can also result from certain biological processes, such as the ejection of undigested seeds by birds, which can result in clusters of seedlings in the vicinity.
- Random Distribution:
- Spatial dispersion refers to the pattern in which individuals of a population are arranged in space. There are three primary types of spatial dispersion:
- Allee’s Principle:
- The concept of Allee’s Principle is important when discussing clumped distribution. It suggests that individuals in a group have higher survival rates compared to isolated individuals, especially when facing environmental stress. This principle demonstrates that group living can provide benefits like protection against predators, more efficient foraging, or the ability to generate heat in cold environments.
- Example: Fish that live in groups can better withstand harmful substances like poisons, as collective mucous and secretions from the group can counteract the poison. Similarly, bees in a hive can collectively generate heat that helps protect the entire group during cold conditions.
- Therefore, population size and density in a clumped distribution can directly influence survival and reproductive success. However, exceeding optimal group size can lead to overcrowding and reduced resource availability, which can have negative effects on population health.
- Refuging:
- Refuging is a special form of aggregation where animals move to a particular area, fulfill their needs (such as food or shelter), and then return to their original habitat. This type of behavior is seen in various socially organized groups, including some human populations in developing countries that may migrate to urban areas temporarily in search of work and then return to rural areas.
- Temporal Dispersion:
- Temporal dispersion refers to the distribution of organisms based on time cycles, such as daily, lunar, or seasonal rhythms. This type of dispersion is influenced by factors like light availability and environmental cycles.
- Example: Some nectar-feeding insects or plankton show circadian rhythms, where they are active during specific periods of the day or night, typically in response to light and dark cycles. Marine animals, such as those following tidal cycles, adjust their activities based on moon phases and tidal patterns. Similarly, terrestrial animals are influenced by photoperiodism, which regulates their activities based on the length of day and night.