What is Population?
- Population is a concept that encompasses the number of individuals present in a particular area or ecosystem. It is a fundamental aspect of understanding human societies, as well as the natural world around us. While the term population commonly refers to human beings, it also extends to include animals, microorganisms, and plants, playing a crucial role in various scientific disciplines like ecology and genetics.
- The origin of the word population can be traced back to the Late Latin term “populationem,” which means a people or a multitude. This Latin word finds its roots in the Latin word “populus,” which signifies a community or a group of individuals.
- When discussing human population, governments and organizations often carry out censuses to accurately determine the size of the resident population within a specific jurisdiction. These censuses involve gathering data on various demographic factors such as age, gender, ethnicity, and occupation. By understanding the size and composition of a human population, policymakers can make informed decisions regarding resource allocation, infrastructure development, and social services.
- In addition to human populations, the concept of population extends to other living organisms. In the field of ecology, population refers to a group of individuals of the same species that occupy a particular habitat or ecosystem. Ecologists study population dynamics, including factors such as birth rates, death rates, migration patterns, and interactions with other species. This knowledge helps in comprehending the health and stability of ecosystems and aids in conservation efforts.
- Genetics is another discipline where the concept of population plays a crucial role. In this context, population refers to a group of individuals of the same species that share a common gene pool. By studying genetic variations within populations, scientists can gain insights into evolutionary processes, genetic diseases, and the impact of environmental factors on genetic diversity.
- Understanding the concept of population is essential for addressing various social, environmental, and scientific challenges. It allows us to analyze and comprehend the dynamics and interactions within and between different groups of individuals, whether they are human beings or other living organisms. By studying populations, we can develop strategies to promote sustainability, preserve biodiversity, and improve the well-being of both human and non-human communities.
Definition of Population
Population can be defined as the total number of individuals, whether human, animal, plant, or microorganism, residing in a specific area or ecosystem.
Features/Characteristics Of Population
Population characteristics are fundamental in understanding ecological dynamics, encompassing various aspects such as size, density, dispersion patterns, and dispersal mechanisms. The properties of a population are analyzed collectively, emphasizing the group rather than individual organisms.
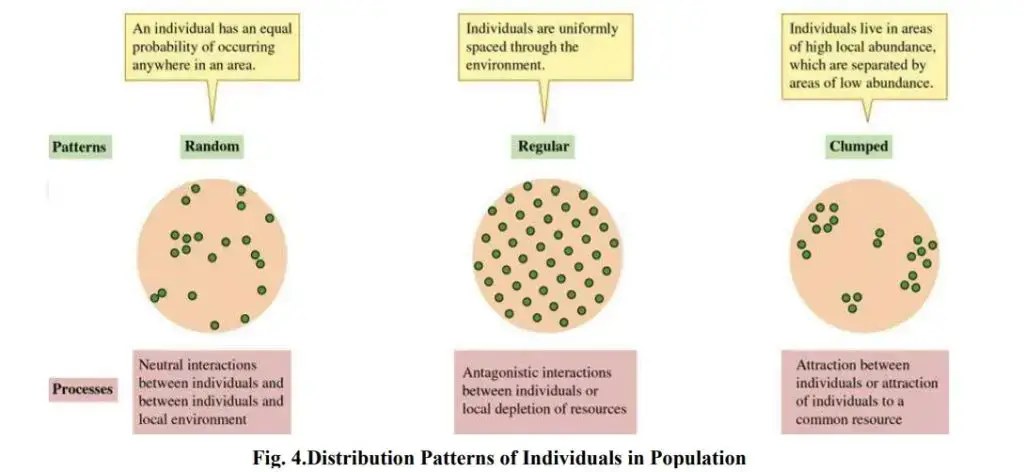
- Size and Density
- Population Size: This refers to the total number of individuals within a specific geographical range. Population size can fluctuate due to various ecological factors, such as resource availability and environmental conditions.
- Population Density: Density is defined as the number of individuals per unit area, serving as a crucial indicator of a species’ adaptability to its habitat. Two primary types of density are recognized:
- Crude Density: This measures the total density across the entire area considered, regardless of habitat suitability.
- Ecological Density: This focuses only on the area that the species occupies, reflecting the reality that populations do not utilize all available space but rather select areas conducive to their survival.
- Dispersion
- Dispersion refers to the spatial arrangement of individuals within their habitat, influencing their interactions and ecological fitness. The patterns of dispersion can be classified as:
- Random Distribution: In this pattern, individual positions are independent, occurring in a uniform environment without strong attraction or repulsion. This distribution is infrequent in nature, as seen in random seed dispersal.
- Uniform Distribution: Individuals maintain equidistant spacing, typically due to intraspecific competition for resources such as nutrients or space. Examples include forest trees, where roots and canopies do not overlap, and territorial animals like penguins.
- Clumped Distribution: This is the most common pattern in nature, where individuals aggregate in specific areas due to shared environmental needs. Clumping enhances survival through cooperative behaviors and resource sharing.
- Dispersion refers to the spatial arrangement of individuals within their habitat, influencing their interactions and ecological fitness. The patterns of dispersion can be classified as:
- Temporal Distribution
- This aspect examines how population distribution changes over time. Examples include the cyclical movements of plankton, seasonal blooming of flowers, and migratory patterns of birds. Such temporal dynamics underscore the importance of time scales in understanding population status.
- Dispersal Mechanisms
- Dispersal is the movement of individuals or their propagules from one habitat to another, vital for survival, growth, and reproduction. The mechanisms of dispersal include:
- Immigration: The influx of individuals into a population, leading to an increase in size.
- Emigration: The outflow of individuals, which reduces population size.
- Migration: Periodic movements that cause temporary population fluctuations.
- Dispersal is the movement of individuals or their propagules from one habitat to another, vital for survival, growth, and reproduction. The mechanisms of dispersal include:
- Types of Dispersal
- Active Dispersal: This occurs in mobile species capable of significant movement, exemplified by migratory birds and butterflies. These organisms can travel vast distances, ensuring gene flow and population stability.
- Passive Dispersal: Typically observed in plants and some stationary animals, this method relies on external agents like wind or animals to transport propagules. For instance, seeds may utilize wind for dispersal, while fruits may be adapted for animal-mediated transport.
- Factors Influencing Dispersion Patterns
- Several factors affect how populations are distributed, including:
- Edaphic and Climatic Features: The soil composition and climate of a habitat can determine where a population can thrive.
- Competition: Intraspecific and interspecific competition can influence resource availability, affecting distribution.
- Reproductive Strategies: The reproductive patterns of species also impact how populations spread and establish themselves in new areas.
- Social Behavior: Interactions within a population, such as cooperation or territoriality, shape distribution patterns.
- Several factors affect how populations are distributed, including:
- Barriers to Dispersal
- Various barriers can hinder dispersal, including:
- Physical Barriers: Changes in habitat, such as land-to-water transitions, can prevent movement.
- Physiological Barriers: Environmental conditions beyond a species’ tolerance limits may impede dispersal.
- Anthropogenic Barriers: Human activities, like urban development, can create obstacles that block movement and genetic exchange.
- Various barriers can hinder dispersal, including:
- Climate Change and Dispersal
- Climate change significantly influences dispersal patterns, affecting species’ geographical ranges and their ability to adapt to new conditions. Understanding how climate alters dispersal is crucial for predicting population dynamics and ecological responses to global environmental shifts.
Types of population
Populations can be categorized into two primary types based on their reproductive mechanisms: unitary populations and modular populations.
- Unitary Populations
- In unitary populations, each individual originates from a single zygote, resulting from sexual reproduction. This characteristic leads to several notable features:
- Distinct Identification: Each individual can be easily identified and distinguished based on fixed morphological traits. For instance, in a population of sheep, characteristics such as two legs, one head, and two ears remain consistent regardless of the individual’s age.
- Predictable Growth: The growth of these individuals follows a determinate pattern, making it easier to forecast their development and life stages.
- Common Examples: Most animal populations, such as those of mammals, birds, and reptiles, fall under this category. Their distinct features and predictable growth patterns allow for straightforward population assessments.
- In unitary populations, each individual originates from a single zygote, resulting from sexual reproduction. This characteristic leads to several notable features:
- Modular Populations
- Modular populations are characterized by the production of individuals through asexual means, allowing for the generation of new modules from a single or a few initial individuals. This results in several important aspects:
- Variability in Morphology: Individuals within a modular population often display significant variation in their physical characteristics. For example, no two plants in a population will exhibit the same number of branches, leaves, flowers, or fruits.
- Vertical and Horizontal Modular Units: The structures that develop into new modules are called vertical modular units, which can include buds that give rise to branches or flowers. Some plants, like grasses and pennywort, also propagate vegetatively through horizontal stems or roots, further illustrating the modular nature of their growth.
- Genets and Ramets: In modular populations, individuals produced by sexual reproduction are termed genets, while those that arise asexually from genets are called ramets. This distinction is crucial for understanding population dynamics, as genets may contribute to genetic diversity while ramets may influence local population density and distribution.
- Common Examples: Plants such as corals, sponges, and certain grass species exemplify modular populations, reflecting their ability to reproduce and propagate through a variety of asexual mechanisms.
- Modular populations are characterized by the production of individuals through asexual means, allowing for the generation of new modules from a single or a few initial individuals. This results in several important aspects:
Population Pyramids
Population pyramids are graphical representations that illustrate the distribution of different age groups and sexes within a population. They provide valuable insights into the demographic structure of a region or country. While there are various types of population pyramids, three common ones are:
- Expansive Pyramid: This type of population pyramid has a triangular shape, with each age group represented by a bar that is shorter than the one preceding it. The wider base of the pyramid indicates a higher birth rate, with a larger number of young individuals. As the age groups progress towards the top of the pyramid, the bars become narrower, indicating a higher mortality rate and shorter life expectancy in the region.
- Constrictive Pyramid: In contrast to the expansive pyramid, the constrictive pyramid displays a slight reduction in the younger age groups. This indicates a lower birth rate compared to the death rate, resulting in a population with a larger proportion of older individuals. The narrowing shape of the pyramid reflects a higher life expectancy and better living conditions, leading to a higher number of older citizens.
- Stable Pyramid: The stable pyramid is characterized by a rectangular shape, suggesting a relatively equal distribution of population across different age groups. The bars representing each age group are roughly of the same length, indicating a stable birth rate over time. A slight tapering at the top of the pyramid signifies an increase in mortality among older individuals, which is a natural occurrence. In regions with stable pyramids, birth control measures, improved living conditions, and access to medical services contribute to higher life expectancy.
Population pyramids provide crucial information for understanding the demographic dynamics and social challenges of a population. They can help identify trends such as population growth, aging populations, and shifts in birth and death rates. Governments, policymakers, and social scientists utilize population pyramids to make informed decisions regarding healthcare, education, retirement policies, and other areas impacted by population dynamics.
Population Growth
Population growth refers to the change in the size and composition of a population over time. Populations are dynamic entities, with individuals constantly being born, growing, reproducing, and dying. These processes collectively contribute to the growth or decline of a population.
Several factors influence population growth. Natality, which refers to the birth rate within a population, contributes to population growth by adding new individuals to the population. Mortality, or the death rate, influences population growth by removing individuals from the population. Immigration and emigration, the movement of individuals into and out of a population, also play a role in population growth. When immigration exceeds emigration, the population size increases, while the opposite occurs when emigration surpasses immigration.
Studying population growth is crucial for understanding the dynamics and future changes of populations. By analyzing changes in population dimensions, such as size, age structure, and sex ratio, researchers can make predictions about future population sizes and growth rates. This information is valuable for conservation efforts, as it helps assess the status and viability of endangered species or populations at risk.
Population growth studies are particularly relevant for invasive species and pathogens. Invasive species can have rapid population growth rates, leading to ecological disruptions and the displacement of native species. Understanding their growth patterns can aid in developing effective management strategies and eradication plans. Similarly, studying the growth of pathogenic populations can inform disease control measures and preventive actions.
Population growth studies also contribute to our understanding of community structure and dynamics. By examining how populations of different species interact and influence each other’s growth, researchers can make predictions about future compositional changes within communities.
Overall, studying population growth provides valuable insights into the dynamics, sustainability, and management of populations. It helps us understand how populations change over time and the factors that influence their growth or decline. By anticipating population sizes and trends, we can make informed decisions to ensure the conservation and well-being of species and ecosystems.
Processes governing growth of population
The growth depends on four significant processes: births, deaths, immigration, and emigration. Natality and mortality are associated with life history strategies, whereas immigration and emigration are dispersal movements.
1. Natality
Natality, also known as the birth rate or fecundity, plays a crucial role in the addition of new individuals to a population. It represents the rate at which new individuals are produced within a population. The birth rate is influenced by various factors, including the species type, environmental conditions, and the number of reproductive individuals present.
Natality can be categorized into two types: absolute or potential natality and realized or ecological natality.
- Absolute natality refers to the maximum number of individuals that can be theoretically produced by each individual under ideal environmental conditions. It represents the biological limit of the population’s reproductive capacity. However, in reality, wild populations seldom reach this limit due to various constraints.
- Realized natality, on the other hand, refers to the actual level of successful reproduction that occurs over time within a population. It is influenced by factors such as environmental conditions, resource availability, and population density. Natality can also be expressed as specific natality, which focuses on population increase under specific conditions. Unlike absolute natality, realized natality is not constant for a given population.
Natality rate can be measured using two different expressions: crude birth rate and specific birth rate. Crude birth rate represents the total population growth per unit time, providing an overall measure of natality. Specific birth rate, on the other hand, is calculated based on a specific criterion and is often used in animal populations. In animal populations, specific birth rate is determined by counting the number and age classes of females present, allowing for the calculation of the number of offspring produced per unit time in different age classes. In demographic studies, specific birth rate is sometimes referred to as fecundity.
The expressions of natality can be represented using mathematical formulas. The absolute or crude natality is denoted as ∆Nn / ∆t, which represents the change in the number of new individuals added to the population over a specific time interval. Specific natality or birth rate is expressed as ∆Nn / N ∆t, which represents the number of new individuals added per unit time divided by the total population or reproductive part of the population.
Determining natality in plant populations can be more challenging due to various factors that make it difficult to measure accurately. Factors such as fluctuation in seed production from year to year and among different age classes, as well as dormancy and unsuccessful germination, can complicate the estimation of natality in plant populations.
Understanding natality is crucial for studying population dynamics, assessing reproductive potential, and formulating conservation strategies. By examining the factors that influence natality and its various expressions, scientists can gain insights into population growth patterns and make informed decisions regarding population management and preservation.
2. Mortality
Mortality refers to the occurrence of death within a population, resulting in a decrease in population size. It is a natural process that affects individuals throughout their life cycle. Mortality can be classified into two main types: minimum or physiological mortality, and ecological or realized mortality.
Minimum or physiological mortality occurs when individuals of a species reach full maturity and die according to their natural life span. This type of mortality is associated with the normal aging process and reflects the inherent biological limits of an organism’s lifespan. It represents the minimum level of mortality that can be expected within a population.
Ecological or realized mortality, on the other hand, is influenced by various external factors such as unfavorable environmental conditions, resource scarcity, competition, or the occurrence of epidemics or diseases. These factors can increase the death rate within a population, leading to higher mortality. Ecological mortality is not solely determined by an organism’s intrinsic life span, but rather by the impact of the surrounding environment and the interactions with other individuals and species.
Both natality and mortality are species-specific processes, meaning that different species exhibit different rates and patterns of birth and death. However, the ultimate outcome of population dynamics is also influenced by other factors and pressures. Intrinsic and extrinsic factors play a role in controlling natural mortality rates. These factors are often linked to individual metabolism and include genetic factors, physiological processes, and overall health.
Understanding mortality rates and the factors influencing them is essential for studying population dynamics and assessing the health and sustainability of populations. Scientists analyze mortality patterns to gain insights into the impact of environmental changes, disease outbreaks, and other factors on population viability. This knowledge can inform conservation efforts, management strategies, and the development of interventions to mitigate the negative effects of mortality on populations.
3. Immigration
Immigration refers to the process of new individuals moving into a population, resulting in an increase in the population size. It is a natural phenomenon that occurs when individuals from one population or geographical area relocate and establish themselves within another population.
The movement of individuals through immigration contributes to population growth and can have various impacts on the receiving population. Immigration can introduce new genetic diversity to the population, which can be beneficial for the overall health and adaptability of the population. It can also increase the available workforce or reproductive potential within the population, leading to potential growth and expansion.
The occurrence of immigration is influenced by several factors. Environmental conditions, such as changes in habitat or resource availability, can trigger migration as individuals seek more favorable conditions. Immigration can also be driven by social factors, such as competition for resources or the formation of new social groups.
The effects of immigration on a population depend on the characteristics of the incoming individuals and their interactions with the resident population. Successful immigration often requires the establishment of suitable habitat, availability of resources, and compatibility with existing individuals. In some cases, immigration can lead to competition or conflict within the population, especially if resources become limited or if there are differences in behavior or genetic composition between incoming and resident individuals.
Immigration is a process commonly observed in animal populations, where individuals may disperse from their natal territories or migrate over long distances to seek new habitats. However, the concept of immigration can also be applicable to human populations, where individuals relocate to different regions or countries, leading to demographic changes and cultural interactions.
4. Emmigration
Emigration refers to the movement of offspring or individuals out of a population, resulting in a decrease in population size. It is a significant process that plays a crucial role in shaping population dynamics and can have various implications for the population and its surrounding ecosystem.
Emigration is more easily observed and calculated in animal populations compared to plant populations. In animal populations, individuals, particularly young offspring, often disperse from their natal habitat or territory to seek new areas for foraging, mating, or establishing their own territories. This movement away from the population contributes to population decline and affects the distribution and composition of individuals within the population.
In plant populations, emigration is not as distinctly visible as in animal populations. However, in the case of plants, the dispersal of seeds or propagules plays a vital role in the emigration process. Seeds or propagules are dispersed by various mechanisms, such as wind, water, animals, or self-dispersal mechanisms. This dispersal allows plants to colonize new areas, leading to the establishment of new populations and potentially contributing to the decline of the source population.
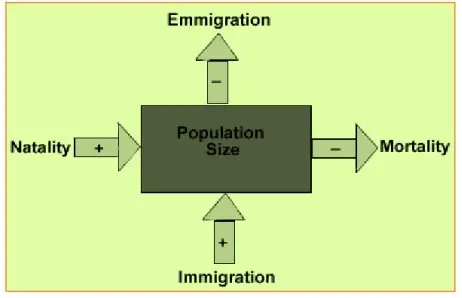
The influence of emigration on population dynamics can be expressed using the population growth equation:
Nt = N0 + B – D + I – E
Where: N0 represents the population size at a given starting time (time zero). Nt represents the population size at a future time, t. B represents the birth rate or natality, which contributes to population growth. D represents the death rate or mortality, which leads to a decrease in population size. I represents immigration, the movement of individuals into the population, which contributes to population growth. E represents emigration, the movement of individuals out of the population, leading to a decline in population size.
Understanding emigration and its interactions with other demographic processes, such as natality, mortality, and immigration, is important for comprehending population dynamics. Emigration can affect gene flow, population density, resource availability, and species interactions within ecosystems. Studying emigration patterns and the factors that drive or influence emigration helps researchers and conservationists assess population viability, habitat connectivity, and the potential impacts of habitat fragmentation on species persistence.
In conclusion, emigration represents the movement of offspring or individuals out of a population, resulting in a decrease in population size. While it is more easily observed and calculated in animal populations, in the case of plants, emigration is facilitated through seed or propagule dispersal. Emigration plays a significant role in population dynamics and can have implications for the overall structure and persistence of populations and ecosystems.
3. Growth Models
Population growth models are valuable tools used to understand and predict the dynamics of populations over time. Two prominent models commonly utilized to describe population growth based on the reproduction of organisms are the exponential model and the logistic model.
The exponential growth model is applicable in situations where resources are essentially unlimited, and individuals do not face significant competition. In this model, population size increases at an accelerating rate, with each individual contributing to the production of new offspring without constraints. The exponential growth model assumes ideal conditions, such as an abundance of resources, absence of predation or disease, and continuous reproduction. While the exponential growth model provides a simplified representation of population growth, it serves as a useful starting point to understand how populations can expand rapidly under optimal conditions.
On the other hand, the logistic growth model is suitable for situations where resources are limited, resulting in competition among individuals within the population. In this model, population growth initially follows an exponential pattern, similar to the exponential growth model. However, as the population approaches its carrying capacity, the rate of growth slows down and eventually stabilizes. The carrying capacity represents the maximum number of individuals that the environment can sustainably support given the available resources. The logistic growth model incorporates the concept of limiting factors, such as food availability, habitat space, and other resources, which eventually constrain population growth. It reflects a more realistic scenario where populations reach a balance with their environment.
Both models provide valuable insights into the dynamics of population growth, but they represent different scenarios based on resource availability and competition. The exponential growth model is useful for understanding situations with abundant resources and a lack of constraints, while the logistic growth model is more applicable to scenarios where resources are limited and individuals must compete for these resources.
It is important to note that these models provide idealized representations of population growth and may not fully capture the complexities of real-world populations. Factors such as environmental variability, predation, disease, and other interactions can significantly influence population dynamics. Nonetheless, growth models serve as essential tools in population ecology, enabling scientists to make predictions, evaluate the impacts of various factors, and develop strategies for managing and conserving populations in a changing world.
Exponential model
The exponential model of population growth is associated with the work of Thomas Robert Malthus (1766-1834), who recognized that any species has the potential to increase its numbers geometrically. The main parameters to consider in this model are the population size (N), the population size at the starting time (N0), and the population size at some future time (Nt).
The rate at which the population increases during one unit of time is represented by the growth factor (λ), which is calculated by taking the ratio of the population size in two adjacent time periods (e.g., t=0 and t=1). For example, if the population size doubles in one unit of time, λ would be 2; if it triples, λ would be 3, and so on. When the population remains stable, λ equals 1, indicating that Nt equals N0. If the population increases, λ is greater than 1 and Nt is greater than N0, while if the population decreases, λ is less than 1 and Nt is less than N0.
To calculate the rate of change in the population’s size, the equation ΔN/Δt = B + I – D – E is used, where ΔN represents the difference in population size over a specific time duration, and Δt represents the difference in time. Assuming that immigration (I) is equal to emigration (E), the equation simplifies to ΔN/Δt = B – D.
By replacing B with b (instantaneous birth rate) and D with d (instantaneous death rate), the equation can be further modified to dN/dt = bN – dN = (b – d) * N. In situations where the time interval is very short and instantaneous rates need to be calculated, Δ is replaced by the derivative d, resulting in the modified equation dN/dt = rN, where r represents the net instantaneous rate of increase.
For large time intervals and exponential growth calculations, the equation used is Nt = N0 * exp(r – t) = N0 * e^(rt). This equation describes the population size (Nt) at a specific future time (t), based on the initial population size (N0) and the instantaneous rate of increase (r).
The exponential model of population growth assumes that all individuals within the population have the same reproductive potential, there is no age structure, reproduction is continuous, and resources are unlimited. The model can yield three possible outcomes: 1) When r = 0, the population size remains constant; 2) When r > 0, the population grows rapidly; and 3) When r < 0, the population declines abruptly. This model is often observed in short-lived species such as microbes and annual plants.
Different versions of the growth rate (r) are used, such as rN = ΔN/NΔt (specific growth rate) and rN = ΔN/Δt × 100 (percentage growth rate). Another version of r is ro/rmax, known as the intrinsic rate of increase, which measures the biotic potential of the population. The difference between rmax and the actual r provides a measure of environmental resistance.
In summary, the exponential model provides a simplified representation of population growth under ideal conditions, where population size increases without constraints. However, it is important to note that in reality, various factors, such as limited resources and environmental limitations, can influence population dynamics and deviate from the assumptions of the exponential model.
Logistic Model
The logistic model of population growth was developed to account for the limitations and constraints that affect population growth. Unlike the exponential model, which assumes unlimited resources and constant growth rate, the logistic model acknowledges that population growth is influenced by factors such as population density and resource availability. This model was initially proposed by Pierre-Francois Verhulst in 1838 and later refined by Raymond Pearl and I.J. Reed in 1920, resulting in the Verhulst-Pearl logistic model.
According to the logistic model, when a population starts growing, resources are abundant and the population can increase rapidly. However, as the population density increases, competition for resources intensifies, and the population growth rate begins to decline. This decline continues as the population approaches a certain threshold called the carrying capacity (K). The carrying capacity represents the maximum number of individuals that a given habitat can support and sustain. It is believed that beyond this capacity, individuals either die or migrate to other habitats with more favorable conditions.
The dynamics of population growth under the logistic model are described by a differential equation: dN/dt = rN(K – N)/K, where dN/dt represents the rate of change in population size over time (t), r is the intrinsic growth rate, N represents the population size, and K is the carrying capacity. The term (K – N)/K in the equation represents the unutilized opportunity for population growth.
The logistic model exhibits two equilibria: N = 0 and N = K. The first equilibrium, where the population size is zero, is unstable because any slight deviation from this state leads to population growth. In contrast, the second equilibrium, where the population size reaches the carrying capacity, is stable. Even with small disturbances, the population tends to return to this equilibrium state.
dN/dt = rN(K-N/K) =rN(1-N/K)
The logistic model incorporates two important ecological processes: reproduction and competition. Both of these processes are density-dependent, meaning they are influenced by the population density. As the population density increases, competition for limited resources becomes more intense, which in turn affects the population growth rate.
The logistic model is commonly observed in long-lived species and is often associated with established ecosystems. It represents a more realistic depiction of population growth, considering the limitations imposed by resource availability and population density.
Time Lags
The concept of time lags in population dynamics recognizes that the response of populations to changes in the environment is not immediate but rather takes time to manifest. These time lags can introduce fluctuations in population growth and prevent the population from reaching its carrying capacity (K) as predicted by the logistic model.
There are two significant time lags to consider: the reaction time lag (w) and the reproductive time lag (g). The reaction time lag represents the delay between changes in the environment and the corresponding adjustment in the population growth rate. This lag reflects the time it takes for populations to respond to alterations in resource availability or other environmental factors. By incorporating the reaction time lag into the logistic equation, it is modified as follows:
dN/dt = rN (K – N(t – w) / K)
The reproductive time lag, on the other hand, accounts for the delay between environmental changes and the subsequent alteration in the reproductive capacity of the population. This lag signifies the time required for individuals to reach reproductive maturity or for changes in reproductive output to occur. Including the reproductive time lag in the equation modifies it as:
dN/dt = rN(t – g) (K – N(t – w) / K)
Both of these time lags introduce complexities in population dynamics and can lead to fluctuations in population growth. The delays in response to changing environmental conditions can cause population sizes to deviate from the carrying capacity and result in oscillations around it. These fluctuations are driven by the interplay between positive feedback from resources stimulating population growth and negative feedback from competition as population density approaches the carrying capacity.
It is important to recognize that these time lags can vary depending on the species, environmental conditions, and the specific context in which the population is situated. By considering the influence of time lags, population ecologists gain a more nuanced understanding of how populations interact with their environment and the factors that can lead to fluctuations in population growth.
Population Regulation
Population regulation refers to the mechanisms and processes that influence the size and growth rates of populations. Understanding population regulation is crucial for predicting and managing populations, assessing the impact of environmental changes, conserving biodiversity, and addressing issues related to pests, diseases, and invasive species.
Population growth studies provide valuable insights into the factors that cause changes in population size and growth rates. By analyzing population dynamics, scientists can unravel the intricate interactions between organisms and their environments. They can identify the drivers of population growth or decline and predict the future trajectory of populations.
The study of population growth helps in assessing the impact of changing environmental conditions on population dynamics. Environmental factors such as resource availability, habitat quality, climate change, and human activities can significantly influence population growth rates. By understanding these influences, scientists can better predict how populations will respond to environmental changes and design effective management strategies.
Population growth studies also play a vital role in pest and disease management. By monitoring population growth patterns, scientists can identify potential outbreaks of pests or diseases, enabling timely intervention and control measures. Understanding the factors that regulate population size and growth rates helps in mitigating the negative impacts of pests and diseases on agriculture, forestry, and human health.
Biodiversity conservation is another area where population regulation is of utmost importance. By studying population growth and its underlying mechanisms, scientists can assess the status of endangered species, monitor population trends, and develop conservation strategies. Population regulation studies help identify the factors threatening species survival and guide efforts to protect and restore their habitats.
In the case of invasive species, understanding population growth is crucial for predicting and managing their spread. By studying the population dynamics of invasive species, scientists can determine their growth potential, rate of spread, and ecological impacts. This knowledge enables the development of effective control and eradication strategies to mitigate the negative consequences of invasions on native ecosystems.
In summary, population regulation is a vital component of population growth studies. By investigating population dynamics, scientists gain insights into the drivers of population growth or decline, the impacts of changing environments, and the strategies for managing populations. This knowledge has implications for various fields, including conservation, pest and disease management, and the understanding of ecological interactions.
Growth forms of Population
The growth forms of populations provide insights into how populations change over time. There are two basic types of population growth forms: the J-shaped population growth form and the S-shaped population growth form.
1 .J – shaped population growth form
The J-shaped population growth form is characterized by exponential growth followed by a sudden crash. The population size increases rapidly over time, showing a steep upward curve. However, after reaching a peak value, the population experiences a sudden decline. This type of growth form is represented by the equation dN/dt = rN, where N represents the number of individuals and r denotes the growth rate. The J-shaped growth form suggests that the population is growing without constraints until it reaches a point where resources become limiting, leading to a sharp decline.
2. S -shaped population growth form
The S-shaped population growth form is characterized by a sigmoid growth curve, depicting changes in population size over time. It exhibits five distinct phases, each representing different dynamics within the population.
- .Lag phase: The first phase is the lag phase, during which individuals adapt to the new environment. This period is marked by a gradual increase in population size as individuals establish themselves and adjust to the prevailing conditions.
- Positive acceleration phase: Following the lag phase, the population enters the positive acceleration phase. During this period, the population experiences a slow but steady increase in size. Factors such as favorable environmental conditions and sufficient resources contribute to this growth.
- Logarithmic or exponential phase: As the positive acceleration phase progresses, the population enters the logarithmic or exponential phase. In this stage, the population undergoes a rapid rise in numbers due to an abundance of food and resources. With plentiful resources and minimal competition, individuals have optimal conditions for growth and reproduction. This exponential increase leads to a steep upward slope on the sigmoid growth curve.
- Negative acceleration phase /Transition Phase: After the exponential phase, the population enters the negative acceleration phase, also known as the transition phase. During this period, the rate of population growth slows down as environmental resistance increases. Resources become limited, and competition among individuals intensifies. As a result, the population experiences a gradual decline in growth rate. Only individuals that are better adapted and suited to exploit the available resources are more likely to survive and reproduce, leading to the elimination of less fit individuals.
- Stationary phase/The Population plateau: Eventually, the population reaches the stationary phase or population plateau. In this phase, the population size remains relatively constant over an extended period, often spanning multiple generations. The constancy in population growth during the stationary phase indicates that the number of individuals being born or immigrating is balanced by the number of individuals dying or emigrating. This equilibrium is maintained by the carrying capacity (K) of the habitat, which represents the maximum population size that the environment can sustain.
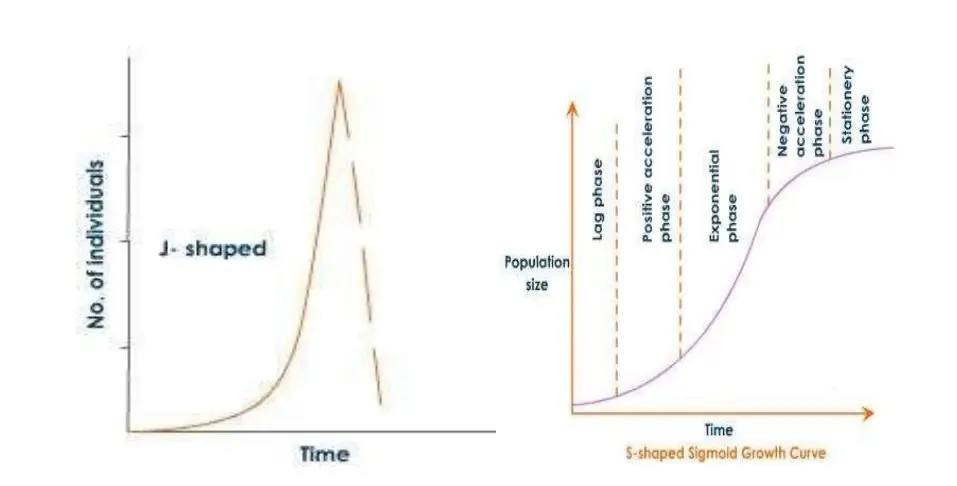
Understanding the S-shaped growth form of populations is crucial for assessing population dynamics and predicting future changes. It allows researchers and ecologists to analyze the factors influencing population growth, such as resource availability, competition, and environmental limitations. By studying the different phases of the S-shaped growth curve, scientists can develop strategies for sustainable population management and conservation practices.
r & K Selected Populations
The concepts of r-selected and K-selected populations are based on the influence of two key factors on population growth: reproductive potential (r) and carrying capacity (K).
Reproductive potential (r) refers to the inherent capacity of an organism to reproduce and produce offspring. It reflects the organism’s ability to have a high number of offspring within a short span of time. Species with a high reproductive potential tend to have a rapid rate of population growth.
Carrying capacity (K) represents the maximum number of individuals that an environment can sustain over the long term. It takes into account the availability of resources, such as food, water, and shelter, as well as the environmental conditions that limit population growth. When a population approaches or exceeds the carrying capacity, there is increased competition for limited resources, which can lead to a decrease in population growth rate.
Based on the dominance of these factors, populations can be classified as r-selected or K-selected populations, and the species that follow these strategies are known as r-selected or K-selected species.
r-selected populations are characterized by high reproductive potential (r) and rapid population growth. These species typically have short lifespans, reach sexual maturity at an early age, produce a large number of offspring, and provide minimal parental care. They are well-suited to environments with abundant resources and little competition. Examples of r-selected species include many insects, small mammals, and certain fish species.
In contrast, K-selected populations are characterized by a population growth strategy that is more influenced by the carrying capacity (K) of their environment. These species have a lower reproductive potential and tend to have a slower population growth rate. They invest more energy in each offspring, have longer lifespans, and often exhibit more advanced parental care. K-selected species are adapted to stable environments with limited resources and high competition. Examples of K-selected species include large mammals like elephants, whales, and primates.
Understanding the concepts of r-selected and K-selected populations helps us comprehend different reproductive strategies and population dynamics. It provides insights into how species adapt to their environments and allocate their resources for reproduction and survival. Additionally, this knowledge is valuable in ecological studies, conservation efforts, and understanding the factors influencing population growth and stability.
Features of r/ K Selected Species
r-selected species and K-selected species exhibit distinct features that are associated with their reproductive strategies and ecological characteristics.
r-selected species are governed by their biotic potential, which represents their maximum reproductive capacity (rmax). These species tend to have the following characteristics:
- Small size: r-selected species are often small in size, which allows them to reproduce quickly and efficiently.
- Fast reproduction: They have a high reproductive rate, producing a large number of offspring within a short span of time.
- Short generation time: The time it takes for individuals to reach reproductive maturity is relatively short for r-selected species.
- Short lifespan: These species typically have a shorter lifespan, as their focus is on rapid reproduction rather than longevity.
- Wide dispersal of offspring: r-selected species disperse their offspring widely, increasing the chances of colonization in new habitats.
r-selected species are opportunistic in nature and are well-adapted to disturbed habitats. They are often the first to take advantage of available resources in freshly burnt grasslands, cut forests, or any other vacant ecological niche. Examples of r-selected species include seasonal and annual plants, weedy species, bacteria, plankton, pest insects, some birds, rodents, and rabbits. Many of these species exhibit semelparity, where they reproduce only once in their lifetime.
The population growth of r-selected species follows the exponential growth equation and is represented by a J-shaped growth curve.
On the other hand, K-selected species are limited by the carrying capacity (K) of their environment. These species have the following characteristics:
- Larger body size: K-selected species tend to have larger body sizes, which allow them to compete effectively for resources.
- Long lifespan: These species have longer lifespans compared to r-selected species.
- Delayed reproduction: K-selected species invest more time in growth and development before reaching reproductive maturity.
- Slow growth: They have a slower growth rate compared to r-selected species.
- Poor dispersal: K-selected species have limited dispersal capabilities and are less likely to colonize new habitats quickly.
K-selected species are characterized by their competitive abilities and their ability to thrive in stable and predictable environments. They produce fewer offspring but provide extensive parental care. Examples of K-selected species include humans, tigers, elephants, and trees. Many of these species exhibit iteroparity, where they reproduce in successive years or breeding seasons.
The population growth of K-selected species follows the logistic growth equation and is represented by an S-shaped growth curve.
The r/K selection theory, developed by Robert MacArthur and E. O. Wilson, highlights the different selection pressures and reproductive strategies adopted by species. It suggests that r-selected species are favored in unpredictable environments, where they can rapidly exploit temporary habitats and niches. In contrast, K-selected species are strong competitors in crowded niches and invest heavily in their offspring, making them well-suited for stable environments. Both r-selected and K-selected species play important roles in maintaining ecosystem dynamics and functioning.
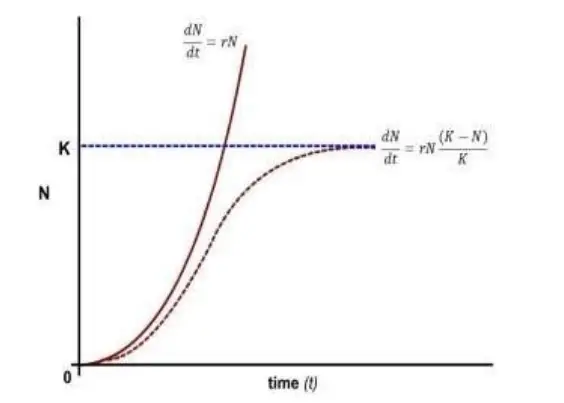
Comparative features of r & K species
Feature | r-selected | K-selected |
---|---|---|
Growth Pattern | Exponential | Logistic |
Growth curve | J-shaped | S-shaped |
Survivorship curve | Type III | Type I or II |
Energy utilization | Waste lot of energy | Efficient energy utilizers |
Life span | Short | Long |
Population size | Variable | Constant at K |
Size of organism | Small | Large |
Maturity | Early | Late |
Parental care | Nil or very little | Much care for offspring |
Mortality | Highly variable & density independent | Often regular & density dependent |
Competition | Poor competitors | Good competitors |
Environment | Unstable | Stable |
Nature | Opportunistic | Equilibrium |
Examples | Annual plants, Weeds, Microbes, Pest Insects, etc. | Perennial trees, Humans, Elephants |
Population growth regulation
Population growth regulation involves the influence of various factors that act to control or limit the growth of populations. These factors can be categorized into two main types: density-dependent factors and density-independent factors.
- Density-dependent regulation occurs when the effects on a population are proportional to its size. As the population size increases, the intensity of these effects also increases. This is primarily due to individuals within the population competing for limited resources. When the population density rises, the available resources may become depleted, causing a change in the carrying capacity (K). If resources increase, the carrying capacity increases, allowing for a larger population size. However, when resources become scarce due to increased density, the mortality rate may rise as individuals experience physiological stress and reduced natality. These processes work together to bring the population back to equilibrium. Intraspecific competition, where individuals of the same species compete for resources, plays a significant role in density-dependent growth regulation. In certain plant populations, such as tree stands, self-thinning is a common phenomenon. Research has shown that even-aged stands exhibit density-dependent mortality, and there is a relationship between individual plant growth and population mortality.
- Density-independent influences, on the other hand, affect populations in the same proportion regardless of their size. Natural disasters like storms, hurricanes, droughts, or floods are examples of density-independent factors. While these factors have an impact on populations, they do not regulate them. W.C. Allee, a notable ecologist known for his work on animal social behavior, proposed a concept called Allee’s principle. According to Allee, both low population density (under-crowding) and high population density (overcrowding) can limit population growth and survival. Allee’s principle holds true in various examples, both in plants and animals. Some plant species tend to occur in groups, which may be a response to habitat preferences, suitable climatic or environmental conditions, or reproductive strategies.
Overall, population growth regulation involves a complex interplay between density-dependent and density-independent factors, shaping the dynamics and size of populations in ecosystems.
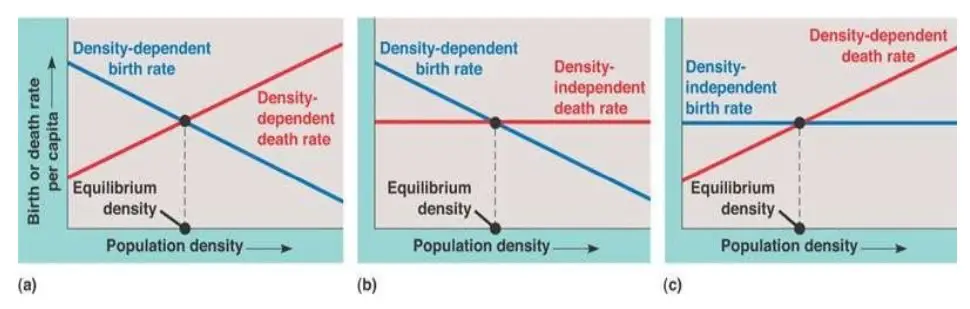
Population Fluctuations
- Population fluctuation refers to the observed ups and downs in population size over time. These fluctuations occur as a result of the interplay between density-dependent and density-independent factors.
- Density dependence, which pushes populations toward their carrying capacity (K), does not always lead to a static equilibrium because the environment is variable, causing K to also vary. Consequently, populations often do not stabilize at K but instead exhibit fluctuations in size. These population fluctuations can be categorized as either erratic (irruptive) or periodic (cyclic).
- Erratic fluctuations are primarily caused by variations in density-independent environmental factors that have a significant and immediate impact on population size. Examples of such factors include fires or other catastrophic events that rapidly and drastically affect the population.
- On the other hand, cyclic fluctuations, also known as oscillations, result from time lags in population responses to their own density. Populations gain “momentum” when high birth rates occur at low densities, leading to an overshoot of the carrying capacity. This overshoot subsequently results in very low survival and birth rates, causing the population to fall below K. Eventually, as birth rates increase due to lowered density conditions, the population undergoes a recovery phase. The cyclic nature of these fluctuations is influenced by the resilience of the population, which determines how quickly the population can regain equilibrium. Reproductive rates play a significant role in the population’s resilience.
- Population fluctuations can occur over various time scales, ranging from millions of years on a geological scale to shorter time scales such as years, seasons, or even weeks. Understanding fluctuations at shorter time scales is particularly important in the study of population dynamics.
- In summary, population fluctuation refers to the ups and downs observed in population size over time. These fluctuations are influenced by both density-dependent and density-independent factors, and can occur as erratic or periodic fluctuations. They play a crucial role in the dynamics of populations and can occur over various time scales.
Types of fluctuations
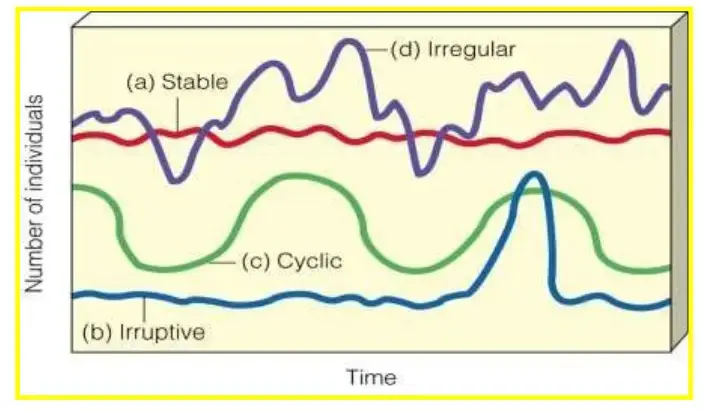
Fluctuations in population size can exhibit different patterns, including stable, irruptive, irregular, and cyclic fluctuations. These patterns provide insights into the dynamics of populations and the factors influencing their growth.
- Stable fluctuations: In stable fluctuations, the population size remains relatively constant and fluctuates around the carrying capacity. The population stays slightly above and below the carrying capacity, indicating a balance between births and deaths. This pattern is commonly observed in species living in fairly constant environments, such as those found in tropical rainforests.
- Irruptive fluctuations: Irruptive fluctuations involve a population that is generally stable but occasionally undergoes explosive growth (irruption) followed by a sharp decline below the carrying capacity. These fluctuations are often triggered by a temporary increase in a limiting resource, which temporarily raises the carrying capacity. Short-lived and rapidly reproducing species are more prone to irruptive fluctuations, as they can quickly take advantage of the available resources.
- Irregular fluctuations: Irregular fluctuations exhibit no apparent recurring pattern and display chaotic behavior in population size. The causes for this type of fluctuation are not well understood, and scientists attribute the irregular behavior to factors such as environmental variability, complex interactions, or stochastic processes. Irregular fluctuations make it challenging to predict population dynamics accurately.
- Cyclic fluctuations: Cyclic fluctuations occur over regular time periods, typically following a multiple-year cycle. These fluctuations involve repetitive patterns of population growth and decline. The length of the cycle can vary depending on the species and environmental conditions. Cyclic fluctuations are often associated with interactions between the population and its environment, such as predator-prey relationships or resource availability.
Understanding the different types of fluctuations can provide valuable insights into population dynamics, the impacts of environmental factors, and the resilience of species. By studying population fluctuations, scientists can gain a better understanding of how populations respond to changes in their environment and develop effective strategies for conservation and management.
Population Fluctuations and Species Conservation
- Population fluctuations play a significant role in species conservation, especially in the face of climate change, habitat destruction, and the threat of extinction. Understanding the dynamics of population fluctuations is crucial for developing effective conservation strategies.
- Climate change has emerged as a major challenge for the biosphere, leading to the extinction of many species and putting others at risk. The changing climate disrupts ecosystems, altering environmental conditions and affecting the availability of resources. These changes can lead to fluctuations in population size and distribution. It is essential to monitor these fluctuations to assess the vulnerability of species and identify populations that are at a higher risk of extinction.
- Habitat destruction, driven by factors such as deforestation, urbanization, and agricultural expansion, is occurring at an alarming rate worldwide. This destruction results in fragmented habitats, isolating populations and increasing their susceptibility to fluctuations. Populations on fragmented islands or isolated patches often experience marked fluctuations that can eventually lead to their disappearance. The likelihood of recolonization is low, making it vital to address these fluctuations and prevent local extinctions.
- In such fragmented and fluctuating conditions, supporting dispersal and interbreeding processes becomes critical for species conservation. Facilitating the movement of individuals between populations can help maintain genetic diversity and increase the resilience of species. Connecting fragmented habitats through corridors or creating stepping-stone habitats can aid in population exchange and mitigate the negative impacts of fluctuations.
- Preserving large population sizes is of utmost importance in species conservation. Larger populations are more likely to withstand normal fluctuations in population size and have a higher chance of persisting over time. By conserving and protecting large populations, we provide a buffer against the risk of extinction caused by fluctuations. Efforts should focus on conserving intact habitats that can support viable population sizes and ensure the long-term survival of species.
- Additionally, proactive conservation measures should be implemented to mitigate the effects of population fluctuations. This includes monitoring population trends, identifying critical habitats, implementing habitat restoration and protection initiatives, and managing threats such as invasive species or overexploitation. Conservation strategies should be adaptive and responsive to changing population dynamics to effectively address the challenges faced by species.
- In conclusion, population fluctuations pose significant challenges to species conservation, particularly in the context of climate change and habitat destruction. Understanding these fluctuations, preserving large population sizes, promoting dispersal and interbreeding, and implementing proactive conservation measures are crucial for safeguarding species and preventing extinctions. By addressing population fluctuations and their underlying causes, we can enhance the resilience and long-term survival of species in a rapidly changing world.
Sampling – What is a Sample?
Sampling is a fundamental concept in statistics, involving the selection of a subset of individuals or observations from a larger population. It allows researchers to gather data efficiently and make inferences about the population based on the characteristics observed in the sample. The process of selecting specific items from the population to form a sample is known as sampling.
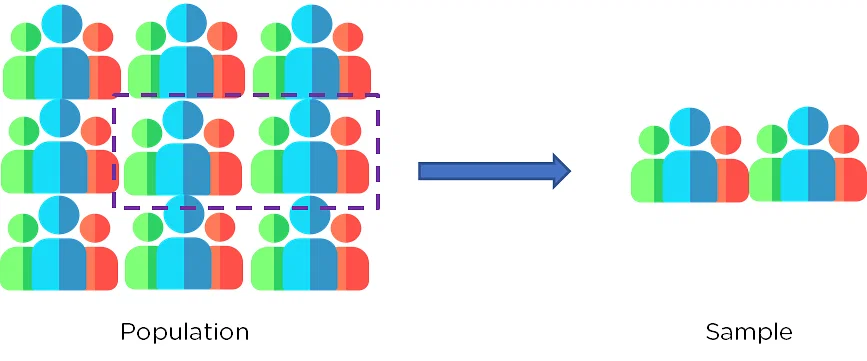
There are two main types of sampling methods:
- Probability Sampling: Probability sampling involves randomly selecting individuals from the population, ensuring that each member has an equal chance of being included in the sample. This method allows for statistical inference and provides a representative sample of the population. Common probability sampling techniques include simple random sampling, stratified sampling, cluster sampling, and systematic sampling.
- Non-probability Sampling: Non-probability sampling methods do not rely on random selection, and the probability of each individual being included in the sample is unknown. This type of sampling is often used when it is difficult or impractical to achieve random selection. Non-probability sampling techniques include convenience sampling, purposive sampling, snowball sampling, and quota sampling. Non-probability sampling may be useful in exploratory research or when obtaining a representative sample is challenging.
To illustrate the concept of sampling, let’s consider an example. Imagine a study aimed at determining the prevalence of malaria in a country’s population. The population would consist of all the people in the country, while the sample would be a subset of individuals selected from this population. In this case, the sample would include only those individuals who have been diagnosed with malaria. By studying this sample, researchers can estimate the proportion of people suffering from malaria in the entire population.
Sampling is necessary when the population is too large to collect data from each member, or when it is impractical or costly to do so. By selecting a representative sample, researchers can gather information that reflects the characteristics of the entire population. It is important for a sample to be unbiased, meaning it should be selected without favoring any particular group or characteristic. Randomness in the selection process helps ensure fairness and reduces potential biases.
In conclusion, sampling is a valuable technique in statistics that allows researchers to collect data efficiently and draw meaningful conclusions about a larger population. It enables researchers to make inferences, generalize findings, and provide insights into various phenomena, ultimately contributing to the advancement of knowledge and decision-making processes.
Population and Sample Formulas
When dealing with population and sample data, there are specific formulas used to calculate population parameters and sample statistics. These formulas provide insights into the central tendency and dispersion of the data. Let’s explore these formulas:
Population Parameter:
- Mean: The population mean (μ) is calculated by summing all the values in the population (ΣX) and dividing it by the population size (N). The formula is as follows: μ = (ΣX) / N
- Standard Deviation: The population standard deviation (σ) measures the dispersion of the values around the population mean. It is calculated by taking the square root of the average of the squared differences between each value (X) and the population mean (μ), divided by the population size (N). The formula is as follows: σ = √[(Σ(X-μ)²) / N]
Sample Statistic:
- Mean: The sample mean (x̄) is calculated by summing all the values in the sample (Σx) and dividing it by the sample size (n). The formula is as follows: x̄ = (Σx) / n
- Standard Deviation: The sample standard deviation (s) estimates the dispersion of the values in the sample. It is calculated by taking the square root of the average of the squared differences between each value (x) and the sample mean (x̄), divided by the sample size minus one (n-1). The formula is as follows: s = √[(Σ(x-x̄)²) / (n-1)]
It’s important to note that the formulas for population parameters and sample statistics are similar but use different notation and have slight differences in calculations. The population formulas utilize the entire population data, while the sample formulas are based on a subset (sample) of the population. Additionally, the sample standard deviation formula divides by (n-1) instead of n to account for the degrees of freedom and provide an unbiased estimate of the population standard deviation.
By applying these formulas, researchers and statisticians can calculate key measures for both populations and samples, facilitating data analysis, hypothesis testing, and making informed decisions based on the characteristics of the data.
Population interactions
Population interactions play a crucial role in shaping the dynamics and structure of ecological communities. These interactions occur between different species and can have significant impacts on population sizes, distribution, and overall ecosystem functioning. Here are some key types of population interactions:
- Competition: Competition arises when two or more species compete for the same limited resources, such as food, water, or territory. This interaction can lead to a struggle for survival and the exclusion of less competitive species from certain areas or niches.
- Predation: Predation is a relationship where one species (predator) feeds on another species (prey) for its survival. Predators have adaptations and behaviors that enable them to capture and consume prey. This interaction regulates prey populations and can influence their behavior, distribution, and evolutionary adaptations.
- Camouflage: Camouflage is a defensive adaptation where an organism blends with its environment to avoid detection by predators. This interaction allows prey species to reduce the risk of predation and increase their chances of survival.
- Symbiosis: Symbiosis refers to long-term interactions between different species where they live together in close association. There are three main types of symbiotic relationships:
- Mutualism: Mutualism is a symbiotic relationship where both species benefit from each other. This interaction can involve mutual exchange of resources, protection, or other services.
- Commensalism: Commensalism occurs when one species benefits from the interaction without causing harm or benefiting the other species. The benefiting species utilizes resources or shelter provided by the other species without affecting it positively or negatively.
- Parasitism: Parasitism is a symbiotic relationship where one species (parasite) benefits at the expense of another species (host). The parasite obtains resources and shelter from the host, often causing harm or weakening it.
These population interactions play a crucial role in maintaining the balance and functioning of ecosystems. They can influence population sizes, distribution patterns, evolutionary adaptations, and overall biodiversity. Understanding these interactions is essential for conservation efforts, as they can impact the stability and resilience of ecological communities in the face of environmental changes and human activities. By studying population interactions, scientists can gain insights into the complex relationships between species and develop strategies for sustainable management and conservation of ecosystems.
Examples of Population interactions
- Competition: Two bird species, the woodpecker and the nuthatch, compete for the same insect larvae in a forest. Both species rely on the larvae as their primary food source, leading to competition for this limited resource.
- Predation: The relationship between a lion (predator) and a zebra (prey) on the African savanna. Lions rely on zebras as a food source and employ hunting strategies to capture and consume them.
- Camouflage: The peppered moth in England provides an example of camouflage. During the industrial revolution, the dark-colored variant of the moth became more prevalent because it blended better with the soot-covered trees, offering protection from predators.
- Symbiosis:
- Mutualism: The relationship between flowering plants and pollinators, such as bees and butterflies. Flowers provide nectar as a food reward, while pollinators transfer pollen between flowers, facilitating plant reproduction.
- Commensalism: Remoras attaching themselves to sharks. Remoras benefit by gaining access to leftover prey or protection provided by the shark, while the shark is unaffected by the presence of the remoras.
- Parasitism: The relationship between a tick (parasite) and a dog (host). The tick feeds on the dog’s blood, obtaining nutrients for its survival and reproduction, while the dog may suffer from discomfort, disease transmission, or other negative effects due to the tick’s presence.
These examples illustrate the different types of interactions and their impacts on the populations involved.
Population Parameter vs. Sample Statistic
Parameter | Population Parameter | Sample Statistic |
---|---|---|
Definition | Numerical value that describes a characteristic of a population | Numerical value that describes a characteristic of a sample |
Calculation | Calculated using data from the entire population | Calculated using data from a sample of the population |
Purpose | Used to describe the entire population | Used to estimate the population parameter |
Precision and Accuracy | Usually more precise and accurate than sample statistic | Usually less precise and less accurate than population parameter |
Notation | Greek letters (e.g., μ for population mean) | Roman letters (e.g., x̄ for sample mean) |
Example | The population mean income of all households in a country | The sample proportion of people who own a car in a randomly selected group of households |
References
- Berryman A.A,2002 ,Population: a central concept for ecology?Oikos , 97, 3: 439–442
- Brooker RW, Travis JM, Clark EJ, Dytham C.,2007, Modelling species’ range shifts in a changing
climate: The impacts of biotic interactions, dispersal distance and the rate of climate change,J Theor
Biol.,245(1):59-65 - Crawley M.J.,1997 Plant Ecology,2nd ed. Blackwell Science,
- Gaston K.J. 2009 Geographic range limits of species Proc Biol Sci. , 276(1661): 1391–1393
- Gray L, He F L. 2009. Spatial point-pattern analysis for detecting density-dependent competition in a
boreal chronosequence of Alberta., Forest Ecology and Management, 259: 98–106. - Perry G L W, Miller B P, Enright N J. 2006. A comparison of methods for the statistical analysis
of spatial point patterns in plant ecology. Plant Ecology, 187: 59–82. - Silvertown, J.W., & Charlesworth. 2001. Introduction to Plant Population Biology, 4th ed. Blackwell
Scientific Publication. - Smith, R.L., & T.M. Smith. 2001. Ecology and Field Biology,6th ed. Benjamin Cummings.
- Zhang Y.T., Li J., Chang S., Li X., Lu Z.2012, Spatial distribution pattern of Picea schrenkiana
population in the Middle Tianshan Mountains and the relationship with topographic attributes,
Journal of Arid Land, 4(4): 457−468 - https://www.studysmarter.co.uk/explanations/biology/ecosystems/populations/
- https://www.simplilearn.com/tutorials/machine-learning-tutorial/population-vs-sample
- https://populationeducation.org/what-are-different-types-population-pyramids/
- https://www.geo41.com/population-structure
- https://testbook.com/maths/population-and-sample
- https://www.ecologycenter.us/species-richness/unitary-and-modular-organisms.html
- https://epgp.inflibnet.ac.in/epgpdata/uploads/epgp_content/S000004BY/P000092/M005137/ET/1513075312populationfeaturese-text.pdf
- https://www.teachmint.com/tfile/studymaterial/class-1st/zoology/unitarymodularpopulationspdf/874d0273-bbe3-4c0d-b6b7-cb541cf7dc02
- https://www.york.ac.uk/biology/staff/ahf/131/Lectures2-3.pdf
- https://people.clas.ufl.edu/rdholt/files/027.pdf
- https://www.numerade.com/ask/question/population-ecology-what-is-a-population-unitary-vs-modular-individuals-what-is-population-density-distribution-vs-geographic-range-distributions-random-uniform-clumped-subpopulations-vs-meta-43987/