What is Ossification?
- Ossification, also known as osteogenesis or bone formation, is the process by which bone tissue is created. It begins during embryonic development and continues until early adulthood, with slight individual variations. There are two types of ossification: intramembranous and endochondral.
- Intramembranous ossification involves the direct conversion of mesenchymal tissue into bone. This process forms the flat bones of the skull, clavicle, and most cranial bones. Mesenchymal tissue precursor cells differentiate into osteoblasts, which lay down the bone matrix directly.
- On the other hand, endochondral ossification starts with mesenchymal tissue transforming into a cartilage intermediate. The cartilage model serves as a template for bone formation. Osteoblasts gradually replace the cartilage with bone tissue, forming the majority of the axial skeleton and long bones.
- Ossification is a critical part of bone remodeling, where old or damaged bone is replaced with new bone tissue. Osteoblasts play a central role in this process by laying down new bone material. Intramembranous ossification is more commonly involved in fracture healing when bones are immobilized with plaster of Paris, while endochondral ossification is predominant when fractures are treated with open reduction and internal fixation using metal implants.
- Heterotopic ossification is a condition where bone tissue forms in abnormal locations outside of the skeleton. It is different from the normal ossification process and can occur due to various factors.
- Calcification, often confused with ossification, refers to the formation of calcium-based salts and crystals within cells and tissues. Calcification is a process that can occur during ossification but is not synonymous with it.
- The exact mechanisms that trigger bone development are still not fully understood. However, growth factors and cytokines are believed to play important roles in regulating the process.
- Ossification occurs in a specific timeline. In the third month of fetal development, ossification begins in long bones. By the fourth month, most primary ossification centers have appeared in the diaphyses (shaft) of the bones. Secondary ossification centers appear in the epiphyses (ends) of bones between birth and 5 years of age. Ossification then spreads rapidly from these centers, with various bones becoming ossified between 5 and 12 years of age in females and 5 to 14 years of age in males. Different bones continue to ossify until complete ossification occurs in specific regions by around 25 years of age.
- It’s important to note that individual variations and factors such as nutrition and overall health can influence the timeline and process of ossification.
Definition of Ossification
Ossification, also known as osteogenesis, is the process of bone formation, where mesenchymal cells differentiate into osteoblasts that lay down new bone tissue. There are two types of ossification: intramembranous, which directly converts mesenchymal tissue into bone, and endochondral, which involves the transformation of mesenchymal tissue into a cartilage intermediate before being replaced by bone. Ossification plays a crucial role in bone development, growth, fracture healing, and bone remodeling.
Time period | Bones affected |
---|---|
Third month of fetal development | Ossification in long bones beginning |
Fourth month | Most primary ossification centers have appeared in the diaphyses of bone. |
Birth to 5 years | Secondary ossification centers appear in the epiphyses |
5 years to 12 years in females, 5 to 14 years in males | Ossification is spreading rapidly from the ossification centers and various bones are becoming ossified. |
17 to 20 years | Bone of upper limbs and scapulae becoming completely ossified |
18 to 23 years | Bone of the lower limbs and os coxae become completely ossified |
23 to 26 years | Bone of the sternum, clavicles, and vertebrae become completely ossified |
By 25 years | Nearly all bones are completely ossified |
Bone formation – Ossification
The replacement of collagenous mesenchymal tissue by bone initiates bone growth. This causes the production of woven bone, a primitive form of bone with randomly ordered collagen fibers, which is then rebuilt into mature lamellar bone, which has regular parallel collagen rings. Osteoclasts and osteoblasts constantly rebuild lamellar bone. The process of bone formation can be separated into two parts: endochondral bone formation/ossification and intramembranous bone formation/ossification.
Intramembranous ossification or Intramembranous bone formation
- Intramembranous ossification, also known as intramembranous bone formation, is a process by which bone develops from fibrous membranes. This type of ossification is responsible for the formation of flat bones, such as the skull, mandible, and clavicles.
- The process begins during embryonic development and continues into adolescence. The connective tissue membrane composed of undifferentiated mesenchymal cells undergoes a transformation into bone. Osteoblasts, specialized bone-forming cells, are derived from mesenchymal cells and deposit an extracellular matrix that eventually hardens with the deposition of calcium. This matrix serves as a template for the future bone.
- During intramembranous ossification, the formation of bone tissue occurs directly from the mesenchymal connective tissue, without an intermediate cartilage stage. The process involves the initiation of ossification centers and the differentiation of osteoblasts that secrete bone matrix. As the matrix hardens, it forms spongy bone around blood vessels. Over time, this spongy bone is remodeled into a thin layer of compact bone on the surface.
- In the case of craniofacial cartilage bones, intramembranous ossification originates from neural crest cells. The earliest evidence of intramembranous bone formation in the skull occurs in the mandible during the sixth week of prenatal development. Ossification centers appear in the calvarial and facial areas by the eighth week.
- Intramembranous ossification is distinct from endochondral ossification, which involves the transformation of cartilage into bone. However, in some bones, such as the sphenoid and mandible, both types of ossification can occur simultaneously.
- Overall, intramembranous ossification plays a crucial role in the development and growth of flat bones, providing structural support and protection for vital organs.
Steps/Process of intramembranous bone formation
The process of intramembranous bone formation involves several steps:
- Differentiation of Mesenchymal Stem Cells: Mesenchymal stem cells within the matrix differentiate into various specialized cells, including blood vessels, osteoblasts, and osteogenic cells.
- Ossification Center Formation: Osteoblasts aggregate in what is known as the ossification center, where bone formation begins.
- Synthesis of Osteoid: Osteoblasts in the ossification center synthesize and secrete osteoid, which is the unmineralized, organic portion of the bone matrix.
- Calcification of Osteoid: The osteoid matrix undergoes calcification as calcium binds to it, resulting in the hardening of the matrix. Osteoblasts become trapped within the calcified matrix and transform into osteocytes.
- Trabecular Bone Formation: Osteoblasts continue to secrete osteoid, which surrounds the blood vessels in the developing bone, forming trabecular or cancellous bone. The blood vessels within the trabecular bone eventually develop into red bone marrow.
- Formation of Compact Bone: Mesenchymal cells on the outer surface of the newly formed bone differentiate into osteoblasts and secrete osteoid, contributing to the formation of compact or cortical bone. This process occurs on the inner surface of the periosteum.
- Remodeling and Maturation: The woven bone, initially formed during the early stages, is remodeled into a mature lamellar bone. Osteoblasts on the periosteum surface continue to produce osteoid, contributing to the thickening of the bone.
- Mineralization and Bone Formation: The osteoid matrix undergoes mineralization, transforming into mature bone tissue. The bone matrix becomes calcified, making the bone relatively impermeable to nutrients and metabolic waste. Trapped blood vessels supply nutrients to the osteocytes and bone tissue.
- Formation of Membranes: An essential membrane of bone, including the periosteum and endosteum, develops. The periosteum is vital for bone survival and plays a role in bone growth and repair.
- Bundle Bone and Lamellar Bone Formation: In intramembranous ossification, two types of bone are formed. Bundle bone develops directly in non-calcified connective tissue, while lamellar bone forms in mineralized matrices. Lamellar bone development occurs within the mineralized matrix and is responsible for the strength and mechanical properties of the bone.
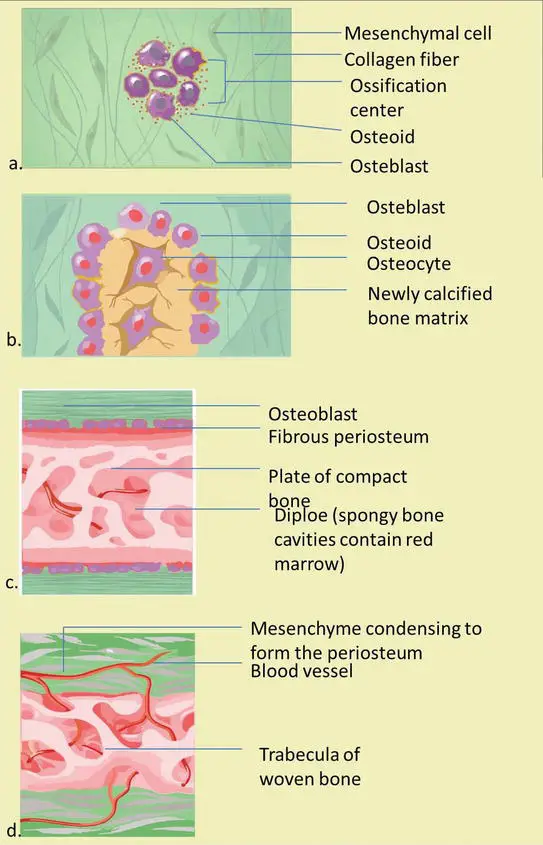
Intramembranous bone formation is a dynamic process involving the differentiation of mesenchymal cells, secretion of osteoid, calcification, and remodeling, resulting in the formation of mature bone tissue.
Factors that influence intramembranous bone formation
Several factors influence the process of intramembranous bone formation. These factors can be categorized into genetic factors, local epigenetic factors, and local environmental factors. Here are the key influences:
- Genetic Factors: Genetic factors play a role in intramembranous bone formation by providing the blueprint for skeletal development. They determine the overall morphology and structure of the bones, as well as the timing and number of growth periods. However, the direct effect of genetic factors on intramembranous bone formation is relatively nonspecific.
- Local Epigenetic Factors: Local epigenetic factors have a significant influence on intramembranous bone formation. These factors are specific to the local environment of bone development and can include factors such as muscle strength, external local pressure, and the presence of neighboring tissues and structures like the brain, eyes, tongue, and nerves. They play a role in guiding the differentiation and activity of osteoblasts and osteogenic cells involved in bone formation.
- Local Environmental Factors: The local environment surrounding the developing bone also influences intramembranous bone formation. This includes factors such as mechanical forces, nutrient supply, and oxygen availability. Mechanical forces, including muscle contractions and external pressure, stimulate bone growth and remodeling. Adequate nutrient supply is essential for the synthesis of bone matrix, and sufficient oxygen levels are required for the metabolic processes involved in bone formation.
- Endochondral Ossification: Endochondral ossification, the process of bone formation from cartilage, indirectly influences intramembranous bone formation. The growth and development of the cartilaginous template in endochondral ossification can impact the surrounding mesenchymal tissue and its potential to undergo intramembranous ossification.
- Orthodontic Therapy: Orthodontic treatment, which involves the use of braces, aligners, or other devices to correct dental and skeletal irregularities, can directly affect intramembranous bone formation. The mechanical forces exerted by orthodontic appliances on the surrounding bones and tissues can stimulate bone remodeling and influence the process of intramembranous bone formation.
Overall, the interplay between genetic factors, local epigenetic factors, local environmental factors, and the influence of neighboring structures and tissues determines the intricate process of intramembranous bone formation. These factors collectively shape the development and remodeling of bones, ensuring their proper growth, strength, and functionality.
Endochondral ossification – Endochondral bone formation
Endochondral ossification, also known as endochondral bone formation, is a vital process in the development of most bones in the body, except for certain flat bones like those in the skull and clavicles. It involves the transformation of a hyaline cartilage template into bone through a series of well-coordinated steps. Here is an overview of the key stages involved in endochondral ossification:
- Formation of Cartilage Model: During embryonic development, a hyaline cartilage precursor begins to form in the 6-8 week embryo. Mesenchymal cells differentiate into chondroblasts, which secrete an extracellular matrix. The chondroblasts become enclosed within the cartilage matrix and mature into chondrocytes. Surrounding the cartilage, the perichondrium forms and will later become the periosteum.
- Hypertrophy and Calcification: Chondrocytes in the center of the cartilage model undergo hypertrophy, increasing in size. Some chondrocytes burst, releasing their contents and triggering calcification of the surrounding matrix. The calcification process leads to the formation of small cavities within the cartilage, providing space for subsequent bone formation.
- Formation of the Primary Ossification Center: The perichondrium surrounding the cartilage contains blood vessels that supply nutrients to the developing bone. Osteoblasts, brought in by these blood vessels, begin to deposit bone around the diaphysis (shaft) of the bone. This results in the formation of a bone collar, which helps prevent nutrient diffusion into the hyaline cartilage and leads to chondrocyte death at the center of the cartilage model. The cavities formed by chondrocyte death become channels for blood vessels and osteogenic cells to penetrate, creating the primary ossification center.
- Replacement of Cartilage with Bone: Osteoblasts continue depositing bone within the cavities, gradually replacing the calcified cartilage matrix. The cartilage template continues to grow as chondrocytes lay down cartilage above and below the primary ossification center. This new cartilage is eventually replaced by bone.
- Formation of Secondary Ossification Centers: After birth, secondary ossification centers develop at the ends of long bones. Mesenchymal cells and blood vessels enter these areas through periosteal buds. The primary and secondary ossification centers are separated by a thin layer of cartilage called the epiphyseal growth plate, which allows for bone elongation and growth.
- Growth Plate Maturation: The growth plate consists of several distinct zones, each with a specific function. These zones include the reserve zone, proliferative zone, hypertrophic zone, calcification zone, and ossification zone. The growth plate allows for longitudinal growth of the bone by the proliferation and replacement of cartilage cells. As individuals reach adulthood, the growth plate matures, and chondrocyte division slows down. Eventually, the growth plate ossifies, leading to the closure of the epiphyseal line and the cessation of bone growth in length.
Endochondral ossification is a dynamic process that lays the foundation for the development and growth of most bones in the body. It involves the precise orchestration of chondrocyte differentiation, cartilage matrix calcification, blood vessel invasion, and osteoblast activity to transform the cartilaginous template into mature bone.
Steps/Process of endochondral bone formation
The process of endochondral bone formation involves several sequential steps that result in the transformation of a cartilaginous template into mature bone. Here is an overview of the key stages involved:
- Mesenchymal Cell Aggregation: Mesenchymal cells group together to form a preliminary shape template of the future bone.
- Differentiation into Chondrocytes: The mesenchymal cells undergo differentiation and become chondrocytes, which are specialized cartilage cells.
- Hypertrophy and Calcification: The chondrocytes undergo hypertrophy, increasing in size. The matrix surrounding the chondrocytes starts to calcify, forming a calcified central cartilage primordium matrix.
- Blood Vessel Invasion: Blood vessels and connective tissue cells invade the calcified cartilage. Nutrient arteries supply the perichondrium and penetrate the nutrient foramen, stimulating osteoprogenitor cells in the perichondrium to differentiate into osteoblasts. This process leads to the conversion of the perichondrium into the periosteum and initiates the formation of ossification centers.
- Periosteum Development and Matrix Production: The periosteum continues to develop, and cell division (chondrocytes) persists. This ongoing division contributes to increased matrix production, enabling the bone to grow in length.
- Appositional Growth: The perichondrial membrane surrounding the bone’s surface develops new chondroblasts, which contribute to growth in width through appositional growth.
- Central Cartilage Lysis and Calcification: Cells located at the center of the cartilage undergo lysis, triggering calcification of the surrounding matrix.
- Endochondral Ossification: The stage of endochondral ossification begins with the differentiation of mesenchymal cells into chondrocytes. This stage involves the formation of the cartilage model of the future bone, the development of the perichondrium, penetration of capillaries into the cartilage, and the transformation of the perichondrium into the periosteum. A periosteal collar of compact bone forms around the cartilage of the diaphysis, and a primary ossification center develops.
- Growth at Bone Ends and Secondary Ossification Centers: As the cartilage continues to grow at the ends of the bone (future epiphyses), bone length increases. The cartilage in the diaphysis is gradually replaced by bone. After birth, secondary ossification centers form in the epiphyseal regions. These centers undergo similar processes of matrix mineralization, chondrocyte death, blood vessel invasion, and osteoblast activity. Each secondary ossification center contributes to bone growth and development.
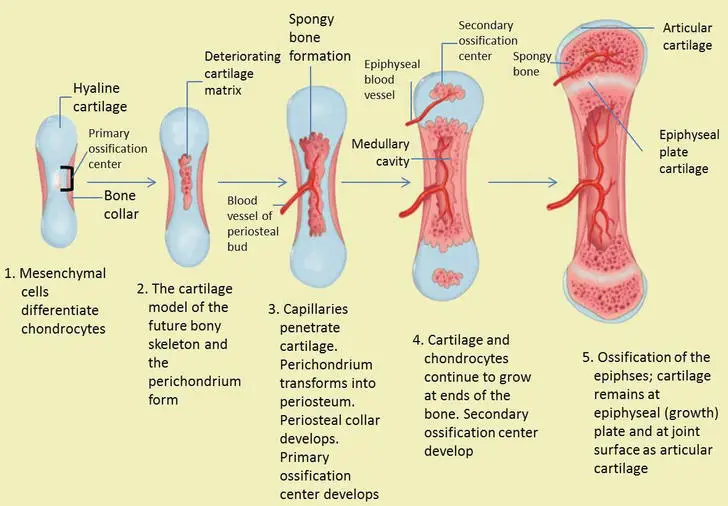
The presence of cartilage is essential in endochondral bone formation, providing flexibility, pressure tolerance, and growth in specific locations. Cartilage growth occurs in adjacent areas and within tissues through chondrocyte cell division and the addition of intercellular matrix. Unlike bone tissue, cartilage cannot directly grow in areas of high compression and relies on the vascularization of the bone formation covering the membrane. Cartilage growth occurs where linear growth is required, allowing the bone to lengthen in the direction of pressure, particularly in areas of strength that have not yet fully developed through membrane ossification in conjunction with periosteal and endosteal surfaces.
Overall, the process of endochondral bone formation is a complex and highly regulated series of events that contribute to the development, growth, and adaptation of bones in the body.
Factors that influence endochondral ossification
Several factors influence the process of endochondral ossification, which plays a crucial role in bone development. Here are some factors that can affect endochondral ossification:
- Membrane Disorders and Vascular Supply: Disorders in the essential membranes involved in endochondral ossification or problems with vascular supply can directly impact bone cell viability and lead to bone damage. The calcified bones formed through endochondral ossification are relatively hard and inflexible, making them sensitive to pressure.
- Genetic Factors: Chondrogenesis, the process of cartilage formation, is primarily influenced by genetic factors. These genetic factors are involved in the initial embryogenesis of facial mesenchyme and play a role in the differentiation of cartilage and cranial bone tissues. Genetic factors contribute to determining the pattern and extent of endochondral ossification.
- Epigenetic and Environmental Factors: While genetic factors have a significant influence on endochondral ossification, the impact of local epigenetic and environmental factors is relatively minor. Epigenetic factors refer to modifications that affect gene expression without altering the underlying DNA sequence. Environmental factors can include physical, chemical, and nutritional influences. In the context of endochondral ossification, these factors have limited effects on the amount of cartilage formation. They have minimal impact on the shape and direction of endochondral ossification, as observed in the growth of the mandibular condyle.
- Cranial Synchondrosis: Cranial synchondrosis, such as the sphenoethmoidal and sphenooccipital growth plates, is influenced by chondrogenesis. These growth plates undergo endochondral ossification and contribute to the development of the cranial base. The genetic factors involved in chondrogenesis determine the growth and fusion of these synchondroses, which ultimately influence the shape and structure of the cranial base.
- Local Factors in Mandibular Condyle Growth: The growth of the mandibular condyle, which also undergoes endochondral ossification, may be more influenced by local factors. Since the condyle cartilage is considered secondary cartilage, it is assumed that local factors have a greater impact on its growth. These local factors, which may include epigenetic and environmental influences, can affect the shape and direction of cartilage formation during endochondral ossification in the mandibular condyle.
In summary, endochondral ossification is influenced by a combination of genetic, epigenetic, and environmental factors. While genetic factors play a significant role in determining the overall process and pattern of endochondral ossification, local factors can have more pronounced effects on specific regions, such as the mandibular condyle. Understanding these factors is essential for comprehending bone development and the potential influences that can impact the formation and growth of bones through endochondral ossification.
What is Chondrogenesis?
Chondrogenesis is the process by which cartilage is formed from condensed mesenchyme tissue. It involves the differentiation of mesenchymal cells into chondrocytes, which are specialized cells responsible for producing the extracellular matrix of cartilage.
The process of chondrogenesis can be described in five steps:
- Production of Extracellular Matrix: Chondroblasts, the precursor cells of chondrocytes, produce the extracellular matrix of cartilage. This matrix provides a firm but flexible support for the developing cartilage tissue.
- Embedding of Cells in the Matrix: As chondroblasts continue to secrete the matrix, they become completely embedded within their own matrix material. At this stage, the chondroblasts transform into chondrocytes. The newly formed chondroblasts can be distinguished from the surface membrane called the perichondrium. This process contributes to the increase in cartilage size through appositional growth.
- Enlargement, Division, and Matrix Production by Chondrocytes: Chondrocytes undergo enlargement, division, and continued production of the extracellular matrix. This leads to an increase in the size of the cartilage mass from within, a process known as interstitial growth.
- Maintenance of an Uncalcified Matrix: The cartilage matrix remains uncalcified. It is rich in chondroitin sulfate, which is associated with non-collagen proteins. Nutrients and metabolic waste are exchanged directly through the soft matrix between the cells, eliminating the need for blood vessels within the cartilage tissue.
- Surface Membrane (Perichondrium) and Adaptation to Pressure: Cartilage is typically covered by a membrane called the perichondrium, although it is not essential for cartilage existence. This property allows cartilage to grow and adapt in areas where pressure is exerted, such as the joints.
Chondrogenesis sets the stage for the subsequent process of endochondral ossification, where cartilage is gradually replaced by bone tissue. During endochondral ossification, hypertrophic cartilage undergoes changes, and the intercellular matrix becomes calcified. Blood vessels and mesenchymal tissues penetrate the calcified cartilage, and the cells differentiate into osteoblasts and osteocytes. The mineralized cartilage is eroded by chondroblasts, forming cavities. Osteoid layers are deposited on the remaining calcified spicules and mineralized to form spongy bone. This process, known as primary spongiosa formation, involves the transformation of cartilage into bone tissue.
Both endochondral and perichondral bone growth occur toward the epiphyses and joints. The lengthening of bones during endochondral ossification depends on the growth of the epiphyseal cartilage. Once the epiphyseal plate closes, bone lengthening ceases. Unlike bone, cartilage growth relies on both appositional and interstitial growth. Various zones with distinct characteristics develop within cartilage bone and merge with each other during the conversion process. Environmental influences, such as orthopedic functional tools, can significantly affect condylar cartilage due to its more superficial location.
Bone growth
Bone growth is a dynamic process that occurs during embryonic development and continues throughout childhood and adolescence until the epiphyseal plates close. Here are some key points about bone growth:
- Embryonic Development: During the third month of intrauterine life, cartilage bone height development takes place. The cartilage plate extends from the nasal bone capsule to the base of the skull. In the early stages, the cartilage skeleton forms without internal blood supply, relying on diffusion for nutrient exchange.
- Ossification Centers: By the fourth month in the uterus, vascular elements develop and reach various points of the cartilaginous skeleton. These points become ossification centers, where the cartilage is gradually replaced by bone. The cartilage continues to grow rapidly, but it is gradually replaced by bone, resulting in the increase of bone mass.
- Cartilage Growth: Cartilage growth in relation to skeletal bone follows a similar pattern to limb growth. The cartilage initially provides a scaffold for bone formation, which is gradually replaced by bone tissue.
- Longitudinal Bone Growth: Longitudinal bone growth occurs at the epiphyseal plates, also known as growth plates. These plates consist of cartilage cells that divide and differentiate, leading to the formation of new bone tissue. The process involves both appositional growth, where bone is added to the outer surface, and remodeling, which includes bone formation and reabsorption.
- Epiphyseal Plate Closure: Bone growth continues until the epiphyseal plates close. In males, this typically occurs around the age of 21, while in females, it generally happens around the age of 18. Once the epiphyseal plates close, longitudinal bone growth ceases.
- Factors Affecting Bone Growth: Proper dietary intake of protein, minerals, and vitamins is crucial for normal bone growth. Vitamin D plays a significant role in calcium absorption from the gastrointestinal tract. A deficiency of vitamin D can result in conditions such as rickets in children or osteomalacia in adults, where bones become soft and weak due to the inability to deposit calcium salts.
Bone growth is a complex and regulated process that involves the coordination of various factors, including genetic, hormonal, and environmental influences. It is essential for the development of a strong and healthy skeletal system.
Oppositional bone growth
Oppositional bone growth refers to the process by which bones increase in thickness through the addition of new bone tissue on the outer surface. Here are some key points about oppositional bone growth:
- Bone Structure: Long bones, such as those found in the limbs, have a central axis called the diaphysis and bony caps at the ends called epiphyses. The area between the epiphyses and diaphysis is known as the epiphyseal plate, which is a layer of hyaline cartilage.
- Epiphyseal Plate: The epiphyseal plate is a crucial center for bone growth, especially in long bones. It consists of five zones of cells and activity. The cartilage cells in the active zone divide and proliferate, leading to the growth of the cartilage.
- Cartilage Maturation: As the cartilage cells in the epiphyseal plate continue to multiply, some of them are pushed towards the diaphysis. These cells undergo hypertrophy and secrete an extracellular matrix. Eventually, the matrix becomes filled with minerals and is rapidly replaced by bone tissue.
- Proliferation and Maturation Balance: During normal growth, the rate of cartilage cell maturation exceeds the proliferation level. This leads to the replacement of cartilage by bone in the epiphyseal plate. As long as cartilage cells continue to multiply, bone growth will continue.
- Completion of Growth: Towards the end of the normal growth period, the epiphyseal plate undergoes closure. The rate of maturation surpasses the proliferation level, resulting in the replacement of cartilage by bone. The epiphyseal plate disappears, and bone growth is considered complete, except for surface changes in thickness that can occur through the activity of the periosteum.
- Hormonal Control: Hormones play a crucial role in regulating bone growth. When chondrocytes, the cartilage cells in the epiphyseal plate, cease their proliferation, and bone replaces the cartilage, longitudinal growth stops. The closure of the epiphyseal plate is marked by the formation of an epiphyseal line. Epiphyseal plate closure typically occurs around 18 years of age in females and 21 years of age in males.
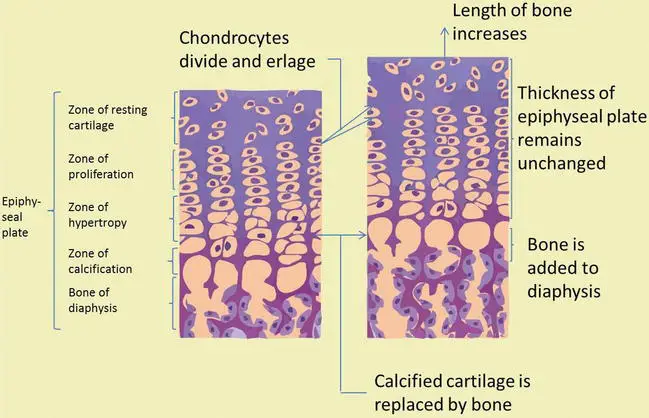
Oppositional bone growth allows bones to increase in thickness and strength, complementing the longitudinal bone growth that occurs at the epiphyseal plates. The balance between cartilage proliferation and maturation, regulated by hormonal control, ultimately determines the completion of bone growth.
Epiphyseal plate growth
Epiphyseal plate growth refers to the process of longitudinal bone growth that occurs at the epiphyseal plates, also known as growth plates. Here are the key stages of epiphyseal plate growth:
- Resting Zone: The resting zone is the region closest to the epiphysis. It consists of hyaline cartilage with a few chondrocytes. In this zone, there are no significant morphological changes in the cells.
- Proliferative Zone: Moving away from the epiphysis, we enter the proliferative zone. In this zone, chondrocytes rapidly divide and form columns of stacked cells that are oriented parallel to the long axis of the bone. The proliferation of chondrocytes contributes to the growth and elongation of the bone.
- Hypertrophic Cartilage Zone: As chondrocytes further mature, they enter the hypertrophic cartilage zone. In this zone, the chondrocytes increase in size and volume. They modify the surrounding matrix, elongating the bone. The cytoplasm of hypertrophic chondrocytes accumulates glycogen. The resorbed matrix between chondrocytes becomes reduced to thin septa.
- Calcified Cartilage Zone: Beyond the hypertrophic cartilage zone lies the calcified cartilage zone. In this zone, the chondrocytes undergo apoptosis, and the thin septa of cartilage matrix become calcified. This calcification provides structural support to the developing bone.
- Ossification Zone: The final zone of the epiphyseal plate is the ossification zone. In this zone, endochondral bone tissue begins to form. Blood capillaries and osteoprogenitor cells, which originate from the periosteum, invade the cavities left behind by the apoptotic chondrocytes. The osteoprogenitor cells differentiate into osteoblasts, which then deposit bone matrix over the three-dimensional calcified cartilage matrix. This process leads to the formation of new bone tissue.
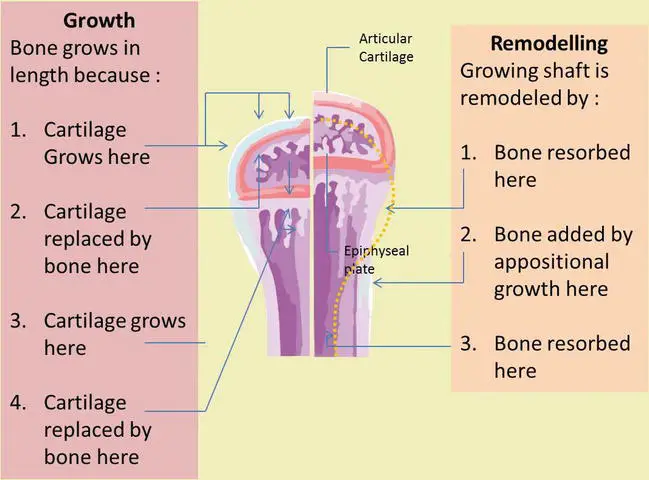
Epiphyseal plate growth is a dynamic process that allows for the longitudinal growth of bones. The proliferation and maturation of chondrocytes, along with the subsequent replacement of calcified cartilage with bone, contribute to the overall increase in bone length. This process continues until the closure of the epiphyseal plates, marking the end of longitudinal bone growth.
Appositional bone growth
- Appositional bone growth refers to the increase in bone diameter that occurs even after longitudinal bone growth has ceased. It involves the addition of new bone tissue on the periosteal surface (outer surface) while simultaneously removing and resorbing existing bone tissue on the endosteal surface (inner surface) of the bone.
- During appositional bone growth, osteoblasts and osteoclasts play crucial roles. Osteoblasts are responsible for secreting new bone matrix on the external surface of the bone, particularly from the diaphysis. This deposition of new bone tissue helps increase the diameter of the bone. On the other hand, osteoclasts are involved in bone resorption on the endosteal surface. They break down and remove old bone tissue from the internal surface of the diaphysis, allowing for the expansion of the medullary cavity.
- As the old bone is resorbed by osteoclasts, the empty space created within the medullary cavity is filled by yellow marrow, which moves in from other areas. Simultaneously, osteoblasts generate new bone tissue beneath the periosteum through a process known as intramembranous ossification. The periosteum, which covers the outer surface of the bone, plays a significant role in increasing the bone’s thickness and shaping its external contour.
- The combined actions of bone resorption along the medullary cavity and new bone deposition under the periosteum lead to an increase in both the diameter of the diaphysis and the diameter of the medullary cavity. This process is referred to as modeling and contributes to the overall structural adaptation and strength of the bone.
- Appositional bone growth ensures that bones continue to adapt and strengthen throughout life, even after longitudinal growth has ceased. It allows for adjustments in bone shape and size to meet the mechanical demands placed on the skeletal system.
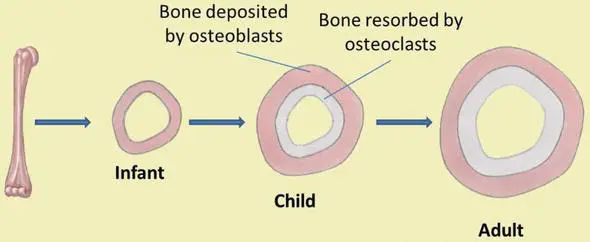
Bone Remodeling and Repair
Bone remodeling and repair are essential processes that allow bones to adapt to stress, maintain their structural integrity, and heal after injury. Throughout adulthood, bone renewal continues through the continuous cycle of bone deposition and bone resorption.
Bone remodeling involves the coordinated activity of two key cell types: osteoblasts and osteoclasts. Osteoblasts are responsible for bone deposition, synthesizing and secreting new bone matrix. They play a crucial role in adding new bone tissue to the existing bone structure. On the other hand, osteoclasts are involved in bone resorption, breaking down and removing old or damaged bone tissue. This dynamic process allows for the removal of worn-out bone and the replacement with fresh, healthy bone tissue.
Proper bone growth and maintenance require various factors, including adequate nutrition. Vitamins D, C, and A, as well as minerals such as calcium, phosphorus, and magnesium, are necessary for normal bone growth. Hormones, including parathyroid hormone, growth hormone, and calcitonin, also play important roles in regulating bone remodeling and maintaining bone health.
Bone turnover rates are relatively high, with approximately five to seven percent of bone mass being recycled each week. However, the turnover rates can vary in different areas of the skeleton and within different regions of a bone. For instance, bone in the head of the femur may be fully replaced every six months, while bone along the shaft may undergo remodeling at a slower rate.
Bone remodeling allows bones to adapt to stress and become stronger when subjected to mechanical loads. When bones are not subjected to normal stress, such as during immobilization in a cast, they may experience bone loss or reduced mass.
When a bone is fractured or broken, a series of stages occurs during the repair process:
- Hematoma formation: Blood vessels in the broken bone tear, leading to the formation of a blood clot or hematoma at the site of the fracture. The clot helps seal the severed blood vessels and provides a foundation for subsequent repair.
- Fibrocartilaginous callus formation: Capillaries grow into the hematoma, and phagocytic cells start to remove dead tissue. Fibroblasts produce collagen fibers that connect the broken bone ends, and osteoblasts begin to form spongy bone. The repair tissue that forms between the broken ends is known as the fibrocartilaginous callus, composed of a combination of hyaline and fibrocartilage.
- Bony callus formation: The fibrocartilaginous callus is gradually converted into a bony callus composed of spongy bone. This process involves the ossification of cartilage, similar to the endochondral bone formation process. Osteoblasts, osteoclasts, and bone matrix are present during this phase.
- Remodeling: The bony callus undergoes remodeling, where osteoclasts and osteoblasts work together to remove excess material from the exterior of the bone and within the medullary cavity. Compact bone is added to the bony callus, gradually restoring the bone’s original structure and strength. This remodeling process can take several months, and the bone may remain uneven for an extended period.
Bone remodeling and repair are complex and dynamic processes that allow bones to heal and adapt to changing mechanical demands. They ensure the maintenance of bone health and integrity throughout life.
Importance of Ossification (Bone Growth and Development)
Ossification, also known as bone growth and development, is a vital process in the formation and maintenance of the skeletal system. It plays a crucial role in providing structural support, protecting internal organs, enabling movement, producing blood cells, and storing minerals. The importance of ossification can be understood through the following points:
- Structural Support: Bones provide a framework that supports and maintains the shape of the body. They create a solid structure that allows us to stand upright, maintain posture, and withstand mechanical stresses and forces.
- Protection of Organs: The skeletal system acts as a protective shield for vital organs. For example, the skull protects the brain, the ribcage protects the heart and lungs, and the spinal column safeguards the spinal cord.
- Facilitation of Movement: Bones, along with muscles and joints, enable movement and locomotion. They act as levers and attachment points for muscles, allowing the body to perform a wide range of movements, from simple actions like walking to complex athletic activities.
- Hematopoiesis: Certain bones contain bone marrow, which is responsible for the production of blood cells. Red blood cells, white blood cells, and platelets are produced within the bone marrow, supporting the body’s immune function and oxygen transport.
- Mineral Storage: Bones serve as reservoirs for important minerals, particularly calcium and phosphorus. When the body needs these minerals for various metabolic processes, bone tissue can release them into the bloodstream to maintain proper mineral balance.
- Bone Metabolism: Ossification involves a balance between bone formation (osteogenesis) and bone resorption (osteolysis). This dynamic process allows bones to adapt to changing mechanical stresses and repair micro-damage, ensuring the maintenance of bone health and integrity.
- Growth and Development: Ossification is essential for the growth and development of the skeletal system. During childhood and adolescence, the growth plates (epiphyseal plates) located at the ends of long bones are responsible for longitudinal bone growth. Ossification also plays a role in shaping and modeling bones, ensuring proper proportions and alignment.
- Repair and Healing: Ossification is crucial for bone repair and healing after fractures or injuries. The deposition of new bone tissue and the remodeling process help restore the integrity and strength of the injured bone, allowing it to regain its function.
Overall, ossification is essential for the formation, growth, maintenance, and repair of the skeletal system. It provides structural support, protects vital organs, enables movement, produces blood cells, stores minerals, and contributes to overall bone health and functionality.
FAQ
What is ossification?
Ossification is the process by which cartilage or fibrous tissue is transformed into bone, leading to bone growth and development.
How does ossification contribute to bone growth?
Ossification occurs at the growth plates (epiphyseal plates) located at the ends of long bones. It involves the conversion of cartilage into bone, leading to longitudinal bone growth.
What is the role of growth plates in ossification?
Growth plates are responsible for longitudinal bone growth during childhood and adolescence. They consist of cartilage that undergoes ossification, allowing bones to grow in length.
How long does bone growth typically continue?
Bone growth continues until the growth plates close. This usually occurs around the age of 18 in females and 21 in males, resulting in the cessation of longitudinal bone growth.
Are all bones in the body capable of ossification?
Yes, all bones in the body undergo ossification to varying extents. However, some bones, such as the flat bones of the skull, undergo different types of ossification compared to long bones.
What factors influence bone growth and ossification?
Factors that influence bone growth and ossification include genetics, hormonal balance (e.g., growth hormone, sex hormones), nutrition (particularly calcium and vitamin D), physical activity, and overall health status.
What is the difference between intramembranous and endochondral ossification?
Intramembranous ossification is the direct conversion of mesenchymal tissue into bone, occurring mainly in flat bones of the skull and some facial bones. Endochondral ossification involves the replacement of cartilage with bone and is the primary process for long bone growth.
Can bone growth be affected by certain medical conditions?
Yes, certain medical conditions can affect bone growth. Examples include growth hormone deficiencies, hormonal imbalances (e.g., hypothyroidism), genetic disorders (e.g., achondroplasia), and nutritional deficiencies (e.g., rickets).
Does ossification only occur during childhood and adolescence?
While ossification is most active during childhood and adolescence, it continues throughout adulthood. Ongoing bone remodeling and repair processes involve a balance between bone formation and resorption.
Can ossification be influenced by external factors or interventions?
Yes, external factors and interventions can impact ossification. Adequate nutrition, regular exercise, hormone therapies (when medically necessary), and certain medications (e.g., bisphosphonates) can affect bone growth and development.