- Fertilization is the process by which a sperm and an egg come together to initiate the formation of a new individual. Both the egg and sperm undergo structural specializations to facilitate this crucial event.
- Eggs, unlike sperm, are non-motile cells. They are surrounded by protective coverings that play important roles in fertilization. These coverings serve to recognize and interact specifically with sperm, ensuring that only one sperm successfully fertilizes the egg and preventing polyspermy (fertilization by more than one sperm). In mammals, the egg possesses a specialized layer called the zona pellucida, which surrounds the egg’s plasma membrane. The zona pellucida acts as a barrier, making the egg impenetrable to more than one sperm. It serves as a protective shield, safeguarding the integrity of the fertilization process.
- Within the mammalian egg, beneath the zona pellucida, lies a layer called the cortical granules. These granules are responsible for certain important functions during fertilization. Once fertilization occurs, the cortical granules release their contents, causing biochemical and structural changes in the zona pellucida. This modification prevents additional sperm from binding to the egg, reinforcing the prevention of polyspermy.
- On the other hand, sperm cells are highly motile and designed for active movement towards the egg. They possess a distinct structure that enables them to navigate through the female reproductive tract and reach the egg. Sperm cells consist of a nucleus containing genetic material, mitochondria that provide the necessary energy source, and a flagellum for movement. The anterior end of the sperm, known as the acrosome, is highly specialized and aids in the penetration of the egg.
- The acrosome contains enzymes that are crucial for the fertilization process. When a sperm reaches the egg, these enzymes are released, allowing the sperm to penetrate the protective layers surrounding the egg. The successful penetration of the egg by the sperm’s nucleus is essential for the fusion of genetic material and the initiation of embryo development.
- Furthermore, sperm cells are also designed to activate the egg upon contact. Once a sperm binds to the egg’s surface, it triggers a series of biochemical reactions within the egg. These reactions lead to the activation of the egg, preparing it for the fusion of genetic material and subsequent development.
- In summary, fertilization involves the interaction of specialized sperm and egg cells. The eggs possess protective coverings, such as the zona pellucida and cortical granules, to ensure successful fertilization by a single sperm and prevent polyspermy. Sperm cells, with their motility and specialized structures, navigate towards the egg, penetrate its protective layers, and deliver their genetic material. The intricate interplay between eggs and sperm during fertilization is vital for the creation of new life.
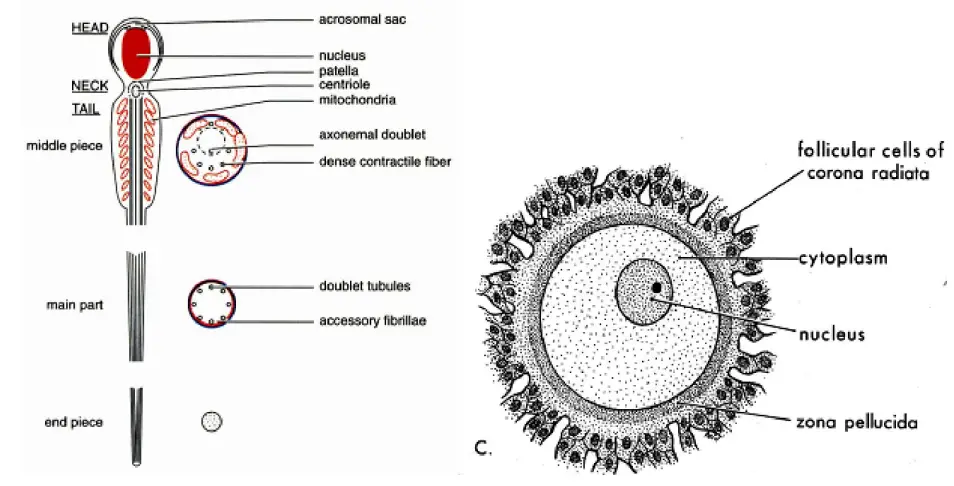
Special features of the gametes for fertilization
Both gametes, the sperm and the egg, possess special features that enable successful fertilization.
Egg:
- Protective Coverings: The egg is surrounded by protective coverings that serve multiple purposes. In mammals, the egg has a specialized layer called the zona pellucida, which surrounds the plasma membrane. This layer prevents the entry of multiple sperm, ensuring that only one sperm fertilizes the egg and avoiding polyspermy.
- Cortical Granules: Beneath the zona pellucida, the egg contains cortical granules. These granules play a crucial role in preventing polyspermy. Upon fertilization, they release their contents, causing modifications in the zona pellucida. These modifications make the egg impenetrable to additional sperm, reinforcing the prevention of polyspermy.
- Recognition Mechanisms: The egg’s coverings also serve as recognition mechanisms. They interact specifically with sperm, ensuring compatibility between the gametes. This recognition mechanism is essential for successful fertilization.
Sperm:
- Motility: Sperm are highly motile cells. They possess a flagellum, a long whip-like tail, that allows them to swim actively towards the egg. This motility is crucial for navigating through the female reproductive tract and reaching the site of fertilization.
- Acrosome: The anterior end of the sperm contains a specialized structure called the acrosome. The acrosome houses enzymes that are necessary for the fertilization process. When the sperm reaches the egg, the acrosomal enzymes are released, facilitating the penetration of the egg’s protective layers.
- Genetic Material: The nucleus of the sperm contains genetic material in the form of DNA. This genetic material will combine with the genetic material of the egg during fertilization, resulting in the formation of a new individual with a unique set of genetic instructions.
- Mitochondria: Sperm cells also contain mitochondria, which are responsible for providing energy for the sperm’s movement. These energy-producing organelles ensure that the sperm has the necessary resources to swim towards the egg and complete the fertilization process.
In summary, the egg and the sperm possess specialized features that are essential for fertilization. The egg has protective coverings, recognition mechanisms, and cortical granules to prevent polyspermy and ensure compatibility with the sperm. Sperm cells are equipped with motility, an acrosome for penetration, genetic material, and mitochondria for energy production. The combination of these special features in both gametes allows for the successful union of sperm and egg, leading to fertilization and the creation of new life.
Basic requirements of fertilization
Fertilization, the process of sperm and egg fusion to initiate the development of a new individual, has several basic requirements.
- Fluid Medium: Fertilization typically occurs in a fluid medium. Different animal species have specific requirements for this medium. In marine organisms, seawater serves as the fluid medium for fertilization. Freshwater organisms, on the other hand, require fresh water for successful fertilization. In viviparous animals, where the embryos develop inside the female’s body, the body fluid of the female serves as the medium for fertilization.
- Sufficient Number of Sperm: To increase the probability of successful fertilization, the number of sperm cells must exceed the number of eggs available for fertilization. This excess ensures that enough sperm are available to encounter and fertilize the eggs. This is particularly important considering that many external factors, such as the fluid medium and the presence of other organisms, may affect the availability and accessibility of the eggs.
- Limited Lifespan of Gametes: Both sperm and eggs have a limited lifespan outside the body. This means that fertilization must occur within a relatively short duration of time for successful conception. In species where eggs are shed in water, such as most invertebrates, fishes, and amphibians, the lifespan of the eggs is relatively short. They are highly susceptible to environmental factors and can only remain viable for a limited period. In contrast, in species where fertilization occurs within the body of the female, the eggs generally have a longer lifespan. This provides a greater opportunity for successful fertilization since the eggs are protected within the female’s reproductive system.
By meeting these basic requirements, organisms increase the chances of successful fertilization. The presence of a suitable fluid medium, an adequate number of sperm, and a timely encounter between the gametes are all essential for the continuation of the species. The specific adaptations related to these requirements may vary among different animal groups, but they ultimately serve the purpose of ensuring the successful union of sperm and egg, leading to the formation of offspring.
Site of fertilization
The site of fertilization can vary depending on the reproductive strategy of the organisms involved. Fertilization can occur either externally or internally.
- External Fertilization: In external fertilization, the gametes are released into the external environment, typically an aquatic medium. Both the male and female parents discharge their gametes into the water, and fertilization takes place outside the bodies of the parents. This method is common in many invertebrates and some vertebrates, such as fish and frogs. The aquatic medium can be either seawater or freshwater, depending on the specific habitat of the organisms involved. External fertilization is associated with the need for a large number of gametes to increase the chances of successful fertilization, as well as the reliance on external factors for the transport and union of the gametes.
- Internal Fertilization: In contrast, internal fertilization occurs when the fertilization process takes place inside the body of the female parent. The male transfers sperm directly into the female’s reproductive tract, where fertilization occurs. Internal fertilization is observed in various organisms, including insects like Drosophila, birds, and mammals, including humans. This method offers several advantages, such as increased protection of the gametes from external factors, greater control over the timing of fertilization, and the ability to provide additional resources and support for embryo development.
The specific site of internal fertilization can vary among different species. In insects like Drosophila, fertilization occurs in the female’s reproductive tract, where the sperm fertilize the eggs before they are laid. In birds, internal fertilization takes place in the female’s oviduct, where sperm from the male fertilizes the developing eggs before they are laid. In mammals, including humans, internal fertilization occurs within the female’s reproductive tract, typically in the fallopian tubes, where sperm and eggs meet and fertilization takes place.
Overall, the site of fertilization can be either external, with gametes meeting in an aquatic environment, or internal, where fertilization occurs inside the female’s body. The choice of external or internal fertilization is influenced by various factors, including the specific reproductive strategies, environmental conditions, and the need for protection and support for the developing embryos.
Mechanism of fertilization
The mechanism of fertilization involves a series of events that lead to the formation of a diploid zygote. While the process has been extensively studied in invertebrates like sea urchins and vertebrates such as amphibians and mammals, the general events of fertilization remain similar across different species. The following are the five general events involved in the process of fertilization:
- Recognition of Egg and Sperm: Fertilization begins with the approach of the spermatozoon (sperm) towards the egg. The sperm must recognize and bind to specific receptors on the surface of the egg. This recognition process ensures that only compatible sperm can successfully fuse with the egg. The attachment and binding of the sperm to the egg’s surface prepare for further interactions.
- Acrosome Reaction and Penetration: Upon reaching the egg’s surface, the sperm undergoes the acrosome reaction. The acrosome, a specialized structure located at the tip of the sperm, releases enzymes that help the sperm penetrate the egg’s protective layers. These enzymes aid in breaking down the barriers surrounding the egg, allowing the sperm to reach the egg’s plasma membrane.
- Fusion of Plasma Membranes: The next crucial step is the fusion of the plasma membranes of the sperm and the egg. Once the sperm has penetrated the egg, the membranes of both gametes merge, allowing the contents of the sperm to enter the egg’s cytoplasm. This fusion of membranes facilitates the transfer of genetic material and organelles from the sperm to the egg.
- Activation of Egg: The fusion of the sperm and egg triggers a series of biochemical reactions within the egg. These reactions collectively lead to the activation of the egg. Activation involves various changes, such as the release of calcium ions, resumption of the cell cycle, and preparation for embryonic development. The activation process is essential for initiating the subsequent development of the fertilized egg.
- Fusion of Egg and Sperm Pronuclei: Following the activation of the egg, the genetic material of the sperm and the egg, in the form of pronuclei, undergo fusion. The male pronucleus, containing the sperm’s genetic material, and the female pronucleus, containing the egg’s genetic material, combine to form a diploid zygote. This fusion of the pronuclei represents the genetic integration of both parents and marks the completion of fertilization.
In summary, the mechanism of fertilization involves the recognition and binding of the sperm to the egg, acrosome reaction and penetration of the egg’s protective layers, fusion of the plasma membranes, activation of the egg, and the final fusion of the pronuclei. These events ensure the successful fusion of genetic material from both parents and initiate the process of embryo development, leading to the formation of a new individual.
1. Recognition of Egg and Sperm
Encounter of spermatozoa and ova
- The encounter of spermatozoa and ova, the male and female gametes, is a complex process that varies depending on the reproductive strategies of different animal species. Special devices or specific behaviors are often employed to facilitate the close approximation of gametes.
- In freshwater animals, the timing of egg spawning by females and sperm shedding by males is highly specific. Females lay their eggs, and immediately after, the male releases the sperm directly onto the eggs. This immediate proximity increases the chances of successful fertilization.
- In marine organisms, however, the timing of gamete shedding may have longer intervals, ranging from weeks to months. This poses a greater challenge for the encounter of sperm and egg because the gametes are released into the open sea, where they can be readily dispersed. To overcome this challenge, a large number of gametes are produced to maintain a sufficient concentration in the water. Additionally, the aquatic medium is shared by other species that may release their gametes at the same time, further complicating the process. The movement of spermatozoa in the water can either be entirely random or influenced by attractive forces associated with the eggs, guiding the sperm towards the location of the eggs.
- During internal fertilization, as seen in mammals, the gametes of both sexes are deposited within the female reproductive tract. Within this environment, fluid movements assist in transporting the gametes to the site of fertilization. The female reproductive tract provides a protected and controlled environment, increasing the likelihood of successful encounters between sperm and egg.
- In summary, the encounter of spermatozoa and ova can be facilitated through various mechanisms depending on the reproductive strategies of the organisms. In freshwater animals, immediate proximity is achieved through specific timing, while in marine organisms, the dispersion of gametes requires the production of a large number of gametes and attractive forces associated with the eggs. In internal fertilization, the fluid movements within the female reproductive tract assist in transporting the gametes to the site of fertilization, providing a controlled environment for successful encounters.
Sperm attraction
- Sperm attraction, also known as chemotaxis, is a phenomenon observed in many animal species where sperm are attracted towards the eggs of their own species by following a chemical gradient secreted by the egg.
- Chemotaxis has been demonstrated in various groups of animals, including cnidarians, molluscs, echinoderms, and urochordates. These organisms release chemical substances that act as attractants for sperm, guiding them towards the eggs. The chemicals involved in this process vary among species but are generally species-specific.
- In the case of sea urchins, the egg jelly surrounding the eggs contains chemotactic factors that attract sperm. One such factor is resact, a 14-amino acid peptide that has been isolated from the egg jelly of the sea urchin species Arbacia punctulata. Resact acts as a specific attractant for sperm of the same species, guiding them towards the eggs for fertilization. Similarly, in other sea urchin species like Strongylocentrotus purpuratus and Hemicentrotus pulcherimus, a peptide called speract has been identified in the jelly surrounding the eggs. Speract is a 10-amino acid peptide that diffuses readily into the seawater and attracts sperm of the respective species.
- These chemical attractants play a crucial role in facilitating the encounter of sperm and eggs. By following the chemical gradient, sperm can navigate towards the eggs more efficiently, increasing the chances of successful fertilization. The species-specific nature of these chemotactic factors ensures that the sperm are attracted only to the eggs of their own species, preventing cross-species fertilization.
- In summary, sperm attraction, or chemotaxis, is a mechanism observed in various animal species where sperm are guided towards eggs by following a chemical gradient. These chemical attractants, such as resact and speract in sea urchins, help ensure the successful encounter of sperm and eggs by attracting sperm of the same species. This process plays a vital role in reproductive success and contributes to the specificity and efficiency of fertilization.
Fertilizin and Anti fertilizin interactions
- The interaction between fertilizin and anti-fertilizin plays a crucial role in mediating the initial contact and binding between sperm and egg, even before they physically make contact. This concept was proposed by F.R. Lillie in 1912, who observed the agglutination and activation of sperm motility when exposed to the egg water surrounding unfertilized sea urchin eggs. This reaction was found to be species-specific.
- Fertilizin, a factor present in the egg jelly coat, was identified as the substance responsible for these effects. It was later discovered that fertilizin is a proteoglycan present in both the jelly coat and the egg’s membranes, including the vitelline membrane and plasma membrane. Fertilizin is composed of varying amino acids and monosaccharides, which differ from one species to another, giving each species its specific type of fertilizin. Each fertilizin molecule possesses multiple “active groups,” allowing it to attach to two or more sperm and bind them together.
- On the other hand, the sperm plasma membrane contains receptor sites called anti-fertilizin, which are acidic proteins. The adhesion of spermatozoa to the egg’s surface is facilitated by the linking of fertilizin molecules with anti-fertilizin molecules. This interaction between fertilizin and anti-fertilizin is similar to an antigen-antibody reaction, where a chemical lock is formed between two complementary substances.
- The main function of fertilizin and anti-fertilizin is to regulate the number of spermatozoa around the egg. By binding to each other, they help thin out the population of sperm cells, reducing the chances of multiple spermatozoa fusing with the egg simultaneously. This ensures that only one sperm successfully fertilizes the egg, preventing polyspermy.
- In summary, the interaction between fertilizin and anti-fertilizin is an important mechanism that regulates the contact and binding between sperm and egg. Fertilizin, present in the egg jelly coat and membranes, attaches to anti-fertilizin on the sperm plasma membrane, facilitating the adhesion of spermatozoa to the egg’s surface. This interaction helps control the number of spermatozoa around the egg, reducing the likelihood of polyspermy and ensuring successful fertilization by a single sperm cell.
2. Acrosome reaction and penetration
The acrosomal reaction in sea urchin
The acrosomal reaction in sea urchins is a critical step in fertilization that enables the sperm to penetrate the surface coats surrounding the egg. When the sperm comes into contact with the egg, it undergoes a series of changes to prepare for fertilization, one of which is the acrosome reaction.
The acrosome is a specialized structure located at the tip of the sperm head, containing various enzymes and molecules necessary for fertilization. The acrosomal reaction involves two distinct processes that work together to facilitate the penetration of the egg’s surface coats.
The first process is the exocytosis of the acrosomal vesicle. Upon contact with the egg, the membrane enclosing the acrosome undergoes fusion with the plasma membrane of the sperm, leading to the release of the contents stored within the acrosome. These contents include enzymes such as hyaluronidase and acrosin, which are crucial for breaking down the protective layers surrounding the egg.
The second process is the extension of the acrosomal process. As the acrosomal vesicle is exocytosed, a specialized membrane extension called the acrosomal process emerges from the sperm head. The acrosomal process contains actin filaments and serves as a dynamic structure that aids in the physical penetration of the egg’s surface layers.
Together, these processes of exocytosis and acrosomal process extension facilitate the penetration of the surface coats surrounding the egg, allowing the sperm to reach the egg membrane and ultimately fertilize it. The acrosomal reaction is a highly regulated and tightly controlled event that ensures successful fertilization in sea urchins.
It is important to note that the acrosomal reaction in sea urchins is specific to this particular species, and variations in the acrosomal reaction can exist among different organisms. However, studying the acrosomal reaction in sea urchins provides valuable insights into the mechanisms of fertilization and the fundamental processes that occur during this crucial stage of reproduction.
a) Exocytosis of acrosomal vesicle
- The exocytosis of the acrosomal vesicle is a crucial process that occurs during the acrosome reaction, triggered by the contact of the sperm with the egg jelly coat component in sea urchins. This reaction is initiated by a fucose-containing polysaccharide present in the egg jelly coat, which acts as a signal to activate the acrosome reaction.
- Upon contact with the egg jelly coat, the fucose-containing polysaccharide stimulates the influx of calcium ions into the sperm head. The increase in calcium concentration serves as a key signal for the acrosome reaction to commence. Calcium ions play a pivotal role in various cellular processes, including the fusion of membranes.
- The increased calcium concentration leads to the fusion of the outer acrosomal membrane with the sperm plasma membrane. This fusion event results in the breakdown of the acrosomal vesicle, releasing its contents. Within the acrosomal vesicle, there are hydrolytic enzymes known as lysins. These lysins are released into the surrounding environment following the breakdown of the acrosomal vesicle.
- The lysins are responsible for digesting the egg envelope locally, breaking down the protective layers surrounding the egg. This localized digestion clears the path for the spermatozoa to reach the egg’s surface, specifically the vitelline membrane. By breaking down the egg envelope, the lysins create a passage for the sperm to successfully reach the egg surface and proceed with fertilization.
- The exocytosis of the acrosomal vesicle is primarily mediated by the calcium-triggered fusion of the acrosomal membrane with the adjacent sperm plasma membrane. Calcium serves as a critical mediator in this process, initiating the fusion event and subsequent release of the acrosomal enzymes.
- It is important to note that the factors present in the egg jelly coat that stimulate the acrosome reaction are highly species-specific. Different species may have distinct components in their egg jelly coat that trigger the acrosome reaction, highlighting the specialized nature of fertilization mechanisms across various organisms.
- Studying the exocytosis of the acrosomal vesicle and the underlying calcium-mediated fusion process provides valuable insights into the molecular events that drive successful fertilization in sea urchins and helps broaden our understanding of reproductive processes in other species as well.
b) Extension of acrosomal process
- The extension of the acrosomal process is the second part of the acrosomal reaction in sea urchins, complementing the exocytosis of the acrosomal vesicle. This process involves the formation and elongation of a specialized structure known as the acrosomal process, tube, or filament, which plays a crucial role in gamete binding and species-specific recognition.
- The acrosomal process is formed through the polymerization of globular active molecules into actin filaments. Actin is a protein that forms the structural framework of cells and is responsible for various cellular processes, including cell movement and shape changes. In the context of the acrosomal reaction, actin polymerization leads to the formation of the acrosomal process.
- After the sperm has penetrated the egg jelly, the acrosomal process comes into contact with the vitelline envelope of the egg. This interaction between the acrosomal process and the vitelline envelope is species-specific, meaning it varies between different sea urchin species. The specificity of this interaction is due to specific molecular interactions between molecules present on the sperm and the egg.
- One crucial molecule involved in species-specific recognition is bindin. Bindin is located specifically on the acrosomal process of the sperm. It acts as a binding protein and interacts with a specific receptor on the vitelline membrane of the egg, known as the bindin receptor. The bindin-receptor interaction is species-specific, meaning the bindin molecule from one species will bind specifically to the corresponding bindin receptor on the vitelline membrane of eggs from the same species.
- This species-specific binding between bindin and its receptor facilitates the recognition and binding of sperm to eggs from the same species, ensuring successful fertilization. It serves as a mechanism to prevent cross-species fertilization and promote reproductive isolation.
- The extension of the acrosomal process, along with the specific molecular interactions between bindin and the bindin receptor, contributes to the precise recognition and binding between sperm and eggs in sea urchins. Understanding these mechanisms sheds light on the complex processes involved in fertilization and reproductive compatibility in different species.
Gamete binding and recognition in mammals
In mammals, the process of gamete binding and recognition plays a crucial role in fertilization, involving several steps and molecular changes.
a) Capacitation
In mammals, fertilization occurs internally, and the reproductive tract of the female plays an active role in the process. Sperm undergo a process called capacitation before they are capable of fertilizing the egg. Capacitation involves physiological changes that occur when the sperm resides in the female reproductive tract for a certain period of time.
During capacitation, several molecular changes take place:
- Albumin proteins present in the female reproductive tract remove cholesterol from the sperm’s plasma membrane, altering its fluidity.
- Certain proteins or carbohydrates on the surface of the sperm are lost during capacitation.
- The membrane potential of the sperm becomes more negative as potassium ions leave the sperm, which opens calcium channels and allows calcium to enter. This influx of calcium facilitates the process of membrane fusion during the acrosome reaction.
- Protein phosphorylation also occurs during capacitation, although it is not fully understood how these events are related or which one specifically causes sperm capacitation.
b) Gamete Binding
The mammalian egg is surrounded by an extracellular envelope called the zona pellucida. Surrounding the zona pellucida is a layer of cumulus cells known as the corona radiata, embedded in a substance called hyaluronic acid. The sperm’s surface has hyaluronidase activity, which helps it penetrate the cumulus cell layer. After penetrating the cumulus cells, the sperm must bind to the zona pellucida before making contact with the egg’s surface.
The zona pellucida in mammals plays a role similar to that of the vitelline envelope in lower vertebrates and invertebrates. It is a glycoprotein matrix synthesized and secreted by the growing oocyte. The zona pellucida has two important functions in fertilization: it binds the sperm and initiates the acrosome reaction.
c) Acrosome Reaction
The binding of the sperm to the zona pellucida triggers the acrosome reaction, allowing the sperm to penetrate the zona pellucida. In mammals, the acrosome reaction involves the fusion of the outer membrane of the acrosome with the sperm’s plasma membrane. This fusion leads to the release of acrosomal contents.
During the acrosome reaction, the outer portion of the acrosomal membrane vesiculates and the acrosomal contents are exocytosed. Several enzymes are released during this process, which enable the sperm to approach the egg’s plasma membrane. Unlike in sea urchins, mammals do not form an acrosomal process during the acrosome reaction.
In summary, in mammals, the process of gamete binding and recognition involves capacitation, where sperm undergo physiological changes, followed by the binding of sperm to the zona pellucida surrounding the egg. This binding triggers the acrosome reaction, leading to the fusion of membranes and the release of enzymes that allow the sperm to approach the egg’s plasma membrane. The mammalian acrosome reaction differs from that of sea urchins, as no acrosomal process is formed.
3. Gamete fusion & Prevention of polyspermy
Sperm & egg plasma membrane fusion
The process of sperm and egg plasma membrane fusion is a critical event during fertilization, enabling the genetic material of the sperm to enter the egg’s cytoplasm.
In sea urchins, all regions of the egg’s plasma membrane are capable of fusing with the sperm. Upon contact, the cytoplasm of the egg bulges forward at the point of contact, resulting in the formation of a structure known as the fertilization cone. The fertilization cone is composed of hyaline cytoplasm and is responsible for facilitating the entry of the sperm into the egg.
During sperm-egg fusion in sea urchins, the actin protein polymerizes into microfilaments, leading to the extension of several microvilli. These microfilaments and microvilli contribute to the formation of the fertilization cone, which aids in the process of sperm entry. The sperm and egg plasma membranes join together, forming a cytoplasmic bridge. This cytoplasmic bridge widens due to actin polymerization, allowing the sperm nucleus and tail to pass through and enter the egg’s cytoplasm.
In mammals, after penetrating the zona pellucida, the sperm enters the perivitelline space surrounding the egg. The fusion of the sperm and egg plasma membranes begins at the equatorial region of the sperm head. At this point, the plasma membranes of the sperm and egg become continuous, forming a cytoplasmic bridge. Through this cytoplasmic bridge, the sperm nucleus enters the egg’s cytoplasm.
In both sea urchins and mammals, the entire sperm, including the nucleus, centriole, mitochondria, and flagellum, typically enters the egg’s cytoplasm. Once the sperm has entered the egg, a fertilization cone is formed, similar to that observed in sea urchins. The fertilization cone is an extension of the egg’s cytoplasm around the sperm head.
The process of sperm-egg membrane fusion is mediated by specific proteins. In mammals, a protein called fertilin is thought to play a role in mediating the fusion of the sperm and egg membranes. The precise mechanisms and proteins involved in the fusion process are still being investigated and may vary between different species.
Overall, the fusion of the sperm and egg plasma membranes is a crucial step in fertilization, allowing the transfer of genetic material from the sperm to the egg and initiating the development of a new individual.
The prevention of polyspermy
Sperm and egg plasma membrane fusion is a crucial step in fertilization, but it is important to ensure monospermy, the entry of only one sperm into the egg. Polyspermy, the entry of multiple sperm, can lead to various abnormalities and even the death of the embryo. To prevent polyspermy, organisms have evolved mechanisms to restrict the number of sperm that can penetrate the egg’s extracellular coats and fuse with the egg.
In fishes, the sperm can enter the egg only through a narrow opening called the micropyle, while the rest of the egg is covered by an impermeable chorion. This structural arrangement ensures that only one sperm can successfully enter the egg.
In sea urchins and mammals, there are restrictions on the number of sperm that can penetrate the extracellular coats surrounding the egg. In mammals, the sperm must undergo a long journey through the female reproductive tract to reach the egg. Additionally, structural changes in the zona pellucida, the glycoprotein matrix surrounding the mammalian egg, block polyspermy.
The most common way to prevent polyspermy is by blocking the entry of more than one sperm into the egg. In many animals, polyspermy is blocked as soon as the first sperm fuses with the egg’s plasma membrane. Sea urchin eggs have evolved two mechanisms to avoid polyspermy. The first is a fast reaction, accomplished by an electrical change in the egg’s plasma membrane. The second is a slower reaction caused by the exocytosis of cortical granules.
The fast reaction in sea urchins involves changes in the egg’s plasma membrane potential, which create an electric barrier preventing additional sperm from fusing. The slower reaction is triggered by the release of cortical granules, specialized secretory vesicles present in the egg. The cortical granules undergo exocytosis, releasing their contents into the perivitelline space. This results in the formation of a fertilization envelope or a physical barrier that blocks the entry of additional sperm.
These mechanisms ensure monospermy and prevent the fusion of multiple sperm with the egg, safeguarding the proper development of the embryo and avoiding abnormalities associated with polyspermy.
a) The fast and temporary block to polyspermy
- The fast block to polyspermy is a temporary mechanism that prevents the fusion of additional sperm with the egg. It is mediated by a rapid and transient depolarization of the egg’s plasma membrane, which occurs shortly after the entry of the first sperm.
- Within 1-3 seconds after the fusion of the first sperm with the egg, the electrical membrane potential across the egg’s plasma membrane undergoes a shift. It changes from -70 mV (resting membrane potential) to +20 mV. This shift is caused by a small influx of sodium ions into the egg, which leads to the depolarization of the egg’s membrane.
- The opening of sodium channels in the egg is facilitated by certain acrosomal proteins present in the sperm. These proteins induce the influx of sodium ions into the egg, resulting in the depolarization of the egg’s plasma membrane. This depolarization serves as the fast block to polyspermy, preventing the fusion of additional sperm with the egg.
- The fast block to polyspermy is a short-lived response, typically lasting for about 60 seconds. After this period, the membrane potential of the egg returns to its original level, restoring its ability to undergo fusion with another sperm if necessary.
- The fast block to polyspermy acts as an immediate defense mechanism to ensure monospermy, allowing only the first sperm to successfully fuse with the egg. It provides a temporary barrier while other mechanisms, such as the slower cortical reaction, are activated to establish a more permanent block against polyspermy.
b) The slow & permanent block to polyspermy.
- The slow and permanent block to polyspermy, known as the cortical reaction, supplements the fast block to ensure that only one sperm fuses with the egg. In sea urchins, sperm entry triggers the release of stored calcium ions from the endoplasmic reticulum in the egg cortex. Within a minute, a wave of calcium ions spreads throughout the entire egg, initiating the cortical reaction.
- The cortical reaction involves the exocytosis of cortical granules, which are located just beneath the egg’s plasma membrane. These granules fuse with the plasma membrane, releasing their contents into the perivitelline space between the plasma membrane and the vitelline envelope.
- Several proteins are released during the cortical reaction, each serving a specific purpose. Proteolytic enzymes, or proteases, break the bonds that attach the vitelline envelope to the egg’s plasma membrane. This creates a perivitelline space and removes any sperm attached to the bindin receptors. Mucopolysaccharides, specifically glycosaminoglycans, are also released, creating an osmotic gradient that causes water to rush into the perivitelline space. This expansion of the vitelline envelope forms the fertilization envelope.
- Another protein released during the cortical reaction is a peroxidase enzyme, which cross-links tyrosine residues on adjacent proteins of the fertilization envelope, hardening it. Additionally, a protein called hyaline forms a coating around the egg, and the egg’s plasma membrane adheres to it. This hyaline layer provides support for blastomeres during cleavage.
- Both the fertilization envelope and the hyaline layer prevent further sperm from binding to the egg’s plasma membrane, effectively blocking polyspermy.
- In mammals, the cortical reaction is similar to that in sea urchins, but the formation of a fertilization envelope does not occur. Instead, hydrolytic enzymes released during cortical granule exocytosis modify the zona pellucida sperm receptors. These changes, known as the zona reaction, prevent additional sperm from binding to the zona pellucida.
- Overall, the cortical reaction, whether in sea urchins or mammals, ensures the monospermy by creating physical barriers and modifying the surrounding structures to prevent the entry and binding of additional sperm, allowing only one sperm to successfully fuse with the egg and deliver its nucleus into the egg cytoplasm.
4. The activation of egg metabolism
The activation of egg metabolism follows the penetration of the sperm into the egg, initiating a series of cytoplasmic reactions. These reactions can be categorized into “early” and “late” responses based on their timing relative to the cortical reaction.
The early responses occur within seconds of the cortical reaction, which is triggered by the fusion of the sperm and egg plasma membranes. This rapid response involves immediate changes in the egg’s cytoplasm. One of the early responses is the temporary depolarization of the egg’s plasma membrane, known as the fast block to polyspermy. This depolarization is caused by a small influx of sodium ions into the egg, resulting from the opening of sodium channels by certain proteins present in the sperm. The fast block to polyspermy is a short-lived mechanism that helps prevent additional sperm from entering the egg.
Following the early responses, the late responses occur several minutes after fertilization begins. These responses are characterized by more profound changes in the egg’s metabolism. The entry of the sperm triggers various biochemical and molecular events within the egg. These events include the activation of enzymes, the release of calcium ions from intracellular stores, changes in protein synthesis, and alterations in gene expression. These processes collectively contribute to the activation of egg metabolism.
During the late responses, the egg’s metabolism becomes highly active and undergoes significant transformations to support early embryonic development. Metabolic processes such as protein synthesis, DNA replication, and cellular respiration are upregulated to provide the necessary energy and building blocks for embryo development. These metabolic changes are crucial for the egg to transition from a quiescent state to an active and proliferative state, facilitating the formation of the early embryo.
In summary, the activation of egg metabolism encompasses a series of cytoplasmic reactions that occur in response to the penetration of the sperm. The early responses involve rapid changes in the egg’s membrane potential, while the late responses entail more profound alterations in the egg’s metabolism, gene expression, and cellular processes. Together, these responses contribute to the initiation of embryonic development and the subsequent formation of the early embryo.
Early responses
- The early responses following the activation of the egg are aimed at preventing polyspermy, which refers to the entry of more than one sperm into the egg. These responses involve two major mechanisms: the fast block and the slow block.
- The fast block is the initial and rapid response to prevent polyspermy. It is initiated by the influx of sodium ions into the egg cell. Within one second of fertilization, the membrane potential of the egg rises, leading to a change in electrical charge across the membrane. This change in membrane potential serves as a quick defense mechanism to inhibit the entry of additional sperm.
- The next step in the early responses is the fusion of the sperm and egg, which occurs within approximately six seconds after fertilization. The fusion of the sperm and egg plasma membranes allows for the transfer of the sperm nucleus and other cellular components into the egg.
- Following the sperm-egg fusion, another important early response is the cortical vesicle exocytosis, which takes place within 15 to 60 seconds after fertilization. This process involves the release of cortical granules, which are small vesicles located beneath the egg’s plasma membrane. The cortical granules undergo exocytosis, where they fuse with the egg’s plasma membrane and release their contents into the perivitelline space surrounding the egg.
- The cortical vesicle exocytosis has several functions. Firstly, it creates a physical barrier by modifying the zona pellucida or the vitelline envelope surrounding the egg, making it impermeable to other sperm. Additionally, the release of enzymes from the cortical granules helps to break down or modify the sperm-binding receptors present on the egg’s surface, further preventing the attachment of additional sperm. The cortical vesicle exocytosis also contributes to the formation of the fertilization envelope, which plays a role in blocking polyspermy.
- In summary, the early responses to the activation of the egg involve the prevention of polyspermy through the fast block and the slow block mechanisms. The fast block is initiated by the influx of sodium ions into the egg, leading to a rapid change in membrane potential. The subsequent steps include the fusion of the sperm and egg plasma membranes and the cortical vesicle exocytosis, which involves the release of enzymes and the modification of the egg’s outer layers. These early responses collectively act as a defense mechanism to ensure that only one sperm successfully fertilizes the egg, preventing the detrimental effects of polyspermy.
Late responses
The late responses following egg activation involve a series of metabolic changes that contribute to various cellular processes:
- Increased rate of respiration: The activation of the egg leads to an increased utilization of glycogen and other food sources to generate energy in the form of ATP. This heightened metabolic activity provides the necessary energy for subsequent cellular processes.
- Activation of NAD kinase and changes in NADH and NADPH levels: NAD kinase converts NAD (nicotinamide adenine dinucleotide) to NADP (nicotinamide adenine dinucleotide phosphate), which serves as a coenzyme for lipid biosynthesis. The increase in NADPH is essential for the formation of new cell membranes during cleavage.
- Ionic changes: The concentrations of various cations, including sodium, potassium, and calcium, undergo intracellular changes. The pH of the egg remains high, and calcium ion concentration plays a significant role in the metabolic activation of the egg.
- Activation of protein and DNA synthesis: Following fertilization, there is an increase in the rate of protein synthesis by utilizing the existing mRNA present in the cytoplasm of the egg. Protein synthesis rates can increase three to twelvefold within five minutes after fertilization. DNA synthesis, initiating replication of the genetic material, typically begins around 20 minutes after fertilization.
- Resumption of meiosis: In many animal species, meiosis, the cell division process that reduces the chromosome number, is arrested at a specific stage and resumes only after fertilization. The precise timing of fertilization varies among different species, and spermatozoa may enter the egg at different stages of maturation.
- Initiation of mitosis: The activation of the egg leads to the initiation of mitosis, the process of cell division, for further development. This is facilitated by the increased rate of DNA synthesis following fertilization and the contribution of the centriole provided by the sperm, which is essential for proper mitotic division.
Late responses involve significant regulatory events, including the production of inositol triphosphate, diacylglycerol, the release of cytoplasmic free calcium ions, and an increase in hydrogen ion concentration. These events contribute to various cellular processes and play crucial roles in shaping the development of the fertilized egg.
In summary, the late responses following egg activation encompass a range of metabolic changes that drive cellular activities such as increased respiration, protein and DNA synthesis, and the resumption of meiosis and initiation of mitosis. These changes are crucial for the progression of early embryonic development and establishment of the necessary cellular machinery for subsequent growth and differentiation.
5. Fusion of genetic material in sea urchins and mammals
In the context of late responses, after the entry of sperm into the egg, several changes occur in the nucleus of both sea urchins and mammals:
In sea urchins:
- The sperm nucleus enters the egg perpendicular to the egg surface. Upon entry, it separates from the mitochondria and flagellum.
- The second meiotic division of the egg is completed, resulting in the formation of a haploid egg nucleus known as the female pronucleus.
- The sperm nucleus undergoes changes and becomes the male pronucleus. Its nuclear envelope becomes vesicular, and the chromatin de-condenses.
- The male pronucleus rotates 180 degrees so that the sperm centriole lies between the male and female pronuclei.
- To facilitate fusion, the male pronucleus must travel a significant distance through the egg cytoplasm to reach the female pronucleus. This movement is mediated by the sperm aster, which is a complex of long microtubules radiating from the sperm centriole.
- The microtubules of the sperm aster push the male pronucleus towards the center of the egg, while also making contact with the female pronucleus and pulling it towards the male pronucleus.
- The two pronuclei migrate towards each other and fuse, forming the diploid zygote nucleus. This fusion of male and female pronuclei is called amphimixis.
In mammals:
- The entry of sperm into the egg is tangential to the egg’s surface.
- Once inside the egg, the sperm nucleus becomes the male pronucleus.
- The sperm centrosome produces asters and makes contact with the female pronucleus.
- The male and female pronuclei migrate towards each other but do not fuse. Instead, they remain adjacent to each other.
- The nuclear envelopes of both pronuclei break down, and the chromatin condenses into chromosomes, aligning them on a common mitotic spindle.
- The paternal and maternal chromosomes become enclosed by a common nuclear membrane only after the completion of the first division of the fertilized egg, forming the nuclei of two blastomeres.
These late responses involving the migration, fusion, and alignment of the pronuclei play crucial roles in the subsequent development and genetic integration of the zygote in both sea urchins and mammals.
Rearrangement of egg cytoplasm
During fertilization, one of the significant consequences is the rearrangement of the egg cytoplasm. While these movements may not be evident in mammals or sea urchin eggs, they play a crucial role in determining cell fate during development in several other animals. Let’s explore two examples: Styela partita (an ascidian) and frogs.
- Styela partita: In Styela partita, the cytoplasmic movements are particularly remarkable. The mature egg exhibits distinct pigmentation in different cytoplasmic regions. The cortical cytoplasm, rich in yellow lipid granules, surrounds a central gray cytoplasm. Upon sperm entry in the vegetal hemisphere of the oocyte, the yellow cortical cytoplasm and the clear cytoplasm derived from the breakdown of the oocyte nucleus start moving towards the sperm in a vegetal direction. As the male pronucleus penetrates deeper into the cytoplasm, the yellow and clear cytoplasm move along with it. Just before the first cleavage, the yellow cytoplasm forms a crescent-shaped region called the mesodermal crescent below the egg’s equator. Simultaneously, a crescent of light gray cytoplasm, known as the notochordal crescent, appears subequatorially on the opposite side of the egg. These cytoplasmic displacements lead to the establishment of four distinct cytoplasmic regions: yellow cytoplasm on one side, light cytoplasm on the other side, yolk granule-rich cytoplasm below the vegetal pole, and clear cytoplasm in the animal hemisphere. This asymmetrical distribution of cytoplasm sets the stage for the irreversible fixation of different cytoplasmic constituents in each cell after the first division.
- Frogs: In frog embryos, the unfertilized egg is radially symmetrical along the animal-vegetal axis. Sperm can enter the egg from any point on the animal hemisphere. However, after sperm entry, the cortical cytoplasm undergoes a rotation of approximately 30 degrees relative to the inner cytoplasm. This rotation causes the underlying cytoplasm near the equator on the opposite side of the sperm entry point to contain diffuse pigment granules, giving it a gray appearance. This region is known as the gray crescent. The rotation of the cortical cytoplasm is of significance as it marks the initiation of gastrulation in amphibian embryos, with the gray crescent playing a crucial role. Additionally, this rotation causes marked changes within the inner cytoplasm. The cytoplasm on the future dorsal side becomes distinct from the future ventral side, where the sperm entered. As a result, the radially symmetrical embryo is transformed into a bilaterally symmetrical embryo. These cytoplasmic movements are responsible for establishing the dorsal-ventral axis of the future embryo.
In both Styela partita and frogs, the rearrangement of the egg cytoplasm plays a vital role in establishing asymmetry, determining cell fate, and setting the stage for subsequent developmental processes.
Preparation for cleavage
- The preparation for cleavage involves the activation of the egg and the initiation of developmental processes. Prior to fertilization, the egg is in a state of metabolic arrest. However, upon the entry of the sperm, the egg is released from this arrest, marking the beginning of development. This activation triggers various cellular events, including active protein synthesis and DNA synthesis within the egg.
- During cleavage, the first cell division is not a random process. Instead, it tends to be specified by two factors: the point of sperm entry and the subsequent rotation of the egg cytoplasm. The location where the sperm enters the egg influences the orientation of the first cleavage plane. Additionally, the rotation of the egg cytoplasm, which occurs as a result of the rearrangement of the cortical cytoplasm, further determines the orientation of the cleavage plane.
- The specific point of sperm entry and the subsequent rotational movements of the egg cytoplasm play a crucial role in establishing the initial patterns of cleavage and cellular divisions. These processes are essential for proper embryonic development and the subsequent formation of distinct cell lineages.