What is Fermentation?
- Fermentation is a metabolic pathway characterized by the enzymatic conversion of organic substrates, predominantly carbohydrates, into simpler compounds, resulting in the production of energy, primarily in the form of adenosine triphosphate (ATP).
- This process occurs in the absence of oxygen, rendering it anaerobic in nature. The primary objective of fermentation is the regeneration of the coenzyme nicotinamide adenine dinucleotide (NAD+), which is essential for glycolysis, a preliminary step in energy extraction.
- From a biochemical perspective, fermentation is defined by the extraction of energy from carbohydrates without the involvement of an electron transport chain or the final electron acceptor, oxygen.
- Instead, in fermentation, organic molecules such as pyruvate or acetaldehyde act as the terminal electron acceptors. This distinguishes fermentation from anaerobic respiration, where molecules like sulfate ions (SO4–2) or nitrate ions (NO3–) serve as the final electron acceptors in the electron transport chain.
- The products of fermentation vary based on the pathway and organisms involved. Two common types include lactic acid fermentation, resulting in the production of lactic acid, and alcoholic fermentation, yielding ethanol and carbon dioxide.
- The net energy gain from fermentation is modest, producing only 2 ATP molecules per glucose molecule, a stark contrast to the up to 32 ATP molecules generated through aerobic respiration.
- Historically, the significance of fermentation was first elucidated by Louis Pasteur in the mid-19th century, marking the inception of zymology, the study of fermentation processes. Microorganisms, both prokaryotic and eukaryotic, are the primary agents of fermentation.
- While some organisms, known as obligate anaerobes, rely solely on fermentation for energy production, others, termed facultative anaerobes, can switch between fermentation and aerobic respiration based on environmental conditions. For instance, under oxygen-limited conditions, human muscle cells can shift to lactic acid fermentation to meet energy demands.
- Commercially, fermentation has profound implications, particularly in the food and beverage sector. The conversion of sugars into ethanol by yeasts is foundational for the production of alcoholic beverages. Similarly, the production of organic acids through fermentation is pivotal for the preservation and flavoring of various food products, from dairy items like yogurt and cheese to fermented vegetables like kimchi.
- In summation, fermentation is an ancient, yet sophisticated, metabolic process that facilitates energy production in the absence of oxygen. Its applications span from fundamental biological processes to the production of a myriad of food products and beverages that have been integral to human culture and diet for millennia.
Definition of Fermentation
Fermentation is an anaerobic metabolic process in which organic substrates are converted into simpler compounds, producing energy, primarily ATP, without the use of oxygen or an electron transport chain. Commonly associated with the production of gases, acids, or alcohols, fermentation is utilized by various microorganisms and is fundamental in many food and beverage preparations.
History of Fermentation
Fermentation, as a practice, traces its roots back to ancient civilizations. Historically, communities harnessed the principles of fermentation to produce a myriad of beverages, including beer from malted barley, wine from grapes, chicha from maize, and octli, now referred to as “pulque,” derived from the agave plant. Despite the widespread application of this technique, the underlying biological mechanisms remained an enigma for centuries.
The dawn of the 17th century marked a pivotal shift in our understanding of fermentation, propelled by advancements in microscopy. Antoni van Leeuwenhoek’s pioneering work with microscopes unveiled the existence of microorganisms, including yeasts. Subsequent enhancements in microscopic technology further elucidated the diverse realm of microorganisms. Among the early revelations was Charles Cagniard de la Tour’s discovery that yeasts, being living microorganisms, played a potential role in the fermentation process. He noted their proliferation through a budding mechanism during alcoholic fermentation.
However, the most significant strides in comprehending fermentation were made by Louis Pasteur in the mid-19th century. Through meticulous experimentation, Pasteur conclusively demonstrated that living yeasts were instrumental in converting glucose into ethanol in fermented beverages, a process he aptly termed “respiration without air.” Furthermore, Pasteur discerned two primary forms of fermentation: alcoholic fermentation, facilitated by yeasts, and lactic acid fermentation, driven by bacteria. His observations underscored that the transformation of sugars to alcohol was contingent on the presence of live yeast, while the acidification of beet juice, resulting in acetic acid production, was attributed to live bacterial species.
Yet, the exact modus operandi of these microorganisms in fermentation remained elusive until the late 19th century. Eduard Buchner, a German chemist, made a groundbreaking discovery by demonstrating that crushed yeast cells could still catalyze the conversion of sucrose into alcohol and carbon dioxide. He introduced the term “zymase” to describe the enzyme complex extracted from yeast responsible for this transformation.
In the subsequent years, the scope of fermentation expanded, with the identification of various organisms, including human muscle cells, capable of executing this metabolic process. Today, our understanding of fermentation stands on the shoulders of these scientific giants, who meticulously unraveled its intricacies over centuries.
Principle of fermentation
Fermentation is a fundamental biochemical process that facilitates the extraction of energy from carbohydrates, particularly glucose, in an environment devoid of oxygen. The process is characterized by a series of enzymatic reactions that ensure the efficient production and utilization of energy molecules.
Initially, glucose undergoes partial oxidation through the glycolytic pathway, resulting in the formation of pyruvate. This process, known as glycolysis, can proceed via the Embden–Meyerhoff (EMP) or Entner–Doudoroff (ED) pathways. As a result, molecules of ATP and NAD(P)H are generated, which are crucial for cellular energy transactions.
In the subsequent phase of fermentation, in the absence of external electron acceptors, pyruvate becomes the focal point. It undergoes reduction, leading to the regeneration of the coenzyme NAD+(P). This regeneration is pivotal for the continuity of the glycolytic pathway, ensuring a steady production of ATP. The reduction of pyruvate culminates in the formation of specific fermentation end-products, such as ethanol or organic acids, depending on the microbial species and environmental conditions.
For instance, in the lactic acid fermentation pathway exhibited by bacteria like Streptococcus lactis, pyruvate is reduced to lactic acid. This reduction facilitates the regeneration of the NAD+ coenzyme, and concurrently, two ATP molecules are synthesized. Conversely, in certain yeasts, such as Saccharomyces, pyruvate is transformed into ethyl alcohol (ethanol), leading to the reformation of NAD+.
It’s noteworthy that fermentation, while essential for many microorganisms, is energetically less efficient compared to aerobic respiration. Specifically, fermentation harnesses merely 5% of the energy that could be potentially obtained through aerobic pathways. Nevertheless, the primary objective of fermentation is the production of ATP via substrate-level phosphorylation and the maintenance of redox balance through the regeneration of NAD+ from NADH.
In essence, the principle of fermentation revolves around the anaerobic metabolism of glucose, ensuring energy production and redox equilibrium in the cell, even in the absence of oxygen.
Fermentation Equation
Fermentation, a metabolic process that occurs in the absence of oxygen, involves the breakdown of organic compounds to produce energy. The specific chemical equation for fermentation depends on the substrates and products involved. Here, we elucidate the primary equations associated with two common types of fermentation: ethanol fermentation and lactic acid fermentation.
- Ethanol Fermentation: Ethanol fermentation, primarily carried out by yeasts, involves the conversion of glucose into ethanol and carbon dioxide. The chemical representation of this process is as follows:
C6H12O6(glucose)→2C2H5OH(ethanol)+2CO2(carbon dioxide)+energyC6H12O6(glucose)→2C2H5OH(ethanol)+2CO2(carbon dioxide)+energy
Simplified, this can be represented as:
C6H12O6→2C2H5OH+2CO2C6H12O6→2C2H5OH+2CO2
This process yields a net gain of two ATP molecules for each glucose molecule metabolized. - Lactic Acid Fermentation: Lactic acid fermentation is a process where glucose is converted into lactic acid. This type of fermentation is commonly observed in muscle cells under anaerobic conditions and in certain bacteria like lactobacilli. The chemical equation for homolactic fermentation is:
C6H12O6(glucose)→2CH3CHOHCOO−(lactate)+energyC6H12O6(glucose)→2CH3CHOHCOO−(lactate)+energy
In its simplified form:
C6H12O6→2C3H6O3C6H12O6→2C3H6O3
Some bacteria, such as Leuconostoc mesenteroides, can further metabolize lactate, leading to the production of not just lactate but also alcohol and carbon dioxide. This heterolactic fermentation can be represented as:
C6H12O6(glucose)→CH3CHOHCOO−(lactate)+C2H5OH(alcohol)+CO2(carbon dioxide)+energyC6H12O6(glucose)→CH3CHOHCOO−(lactate)+C2H5OH(alcohol)+CO2(carbon dioxide)+energy
In conclusion, the specific equation for fermentation is contingent on the type of fermentation and the organisms involved. These equations provide a snapshot of the chemical transformations that occur during the fermentation process, highlighting the versatility and adaptability of various organisms in energy production.
Types of fermentation
Fermentation is a metabolic process that facilitates the conversion of carbohydrates into simpler compounds, primarily in anaerobic conditions. Various types of fermentation have been identified, each characterized by specific substrates, microorganisms, and end products. Here, we delve into the primary types of fermentation:
- Lactic Acid Homofermentation:
- Reaction: Glucose → Lactic acid
- Microorganisms: Genera such as Lactococcus, Enterococcus, Streptococcus, Pediococcus, and specific Lactobacillus species.
- Characteristic: Homolactic bacteria convert glucose exclusively into lactic acid. Lactococcus spp., for instance, is integral to dairy starter cultures.
- Lactic Acid Heterofermentation:
- Reaction: Glucose → Lactic acid + Acetic acid + Ethyl alcohol + 2CO2 + H2O
- Microorganisms: Genera like Leuconostoc, Oenococcus, and Weissella, along with heterofermentative lactobacilli.
- Characteristic: Heterofermentative LAB produce a mix of lactic acid, ethanol/acetic acid, and carbon dioxide.
- Propionic Acid Fermentation:
- Reaction: Glucose → Lactic acid + Propionic acid + Acetic acid + CO2 + H2O
- Microorganisms: Genus Propionibacterium and species Clostridium propionicum.
- Characteristic: These bacteria can utilize both sugar and lactate, producing propionate through a series of intermediate reactions.
- Diacetyl and 2,3-Butylene Glycol Fermentation:
- Microorganisms: Genera such as Enterobacter, Erwinia, Hafnia, Klebsiella, and Serratia.
- Characteristic: The production of 2,3-butanediol involves a unique double decarboxylation step.
- Alcoholic Fermentation:
- Reaction: Glucose → Ethyl alcohol
- Microorganisms: Yeasts, certain fungi, and bacteria.
- Characteristic: Pyruvate, formed via the EMP or ED pathways, is reduced to ethanol, ensuring redox balance by regenerating NAD+.
- Butyric Acid Fermentation:
- Reaction: Glucose → Acetic acid + Butyric acid
- Microorganisms: Predominantly the genus Clostridium.
- Characteristic: Acetyl-CoA is oxidized to produce butyric acid, with certain species like Clostridium acetobutylicum undergoing acetone butanol fermentation.
In summary, fermentation processes are diverse, each tailored to specific substrates and yielding distinct products. The choice of microorganisms and the metabolic pathways they employ dictate the type and efficiency of the fermentation process.

Products of fermentation
Fermentation, a metabolic process, results in the production of various compounds depending on the specific enzymes and microorganisms involved. This process has been harnessed for various industrial, food, and medical applications. Here, we elucidate the primary products and their significance.
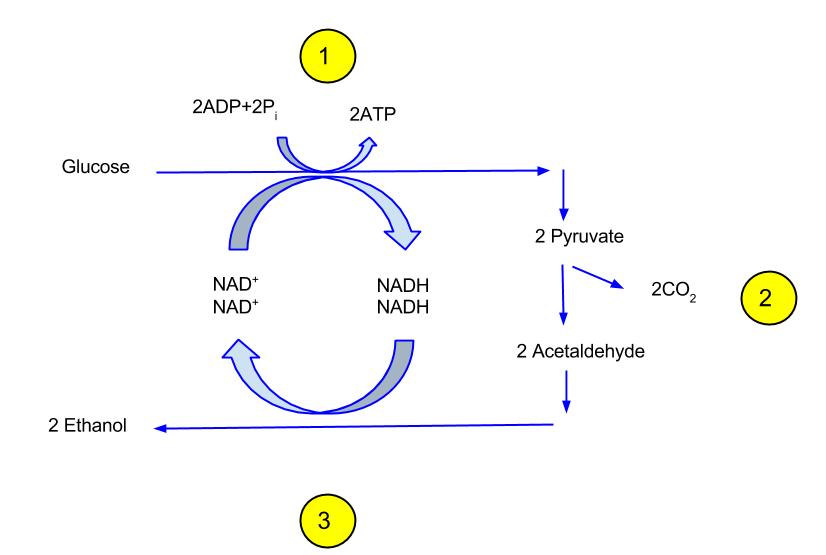
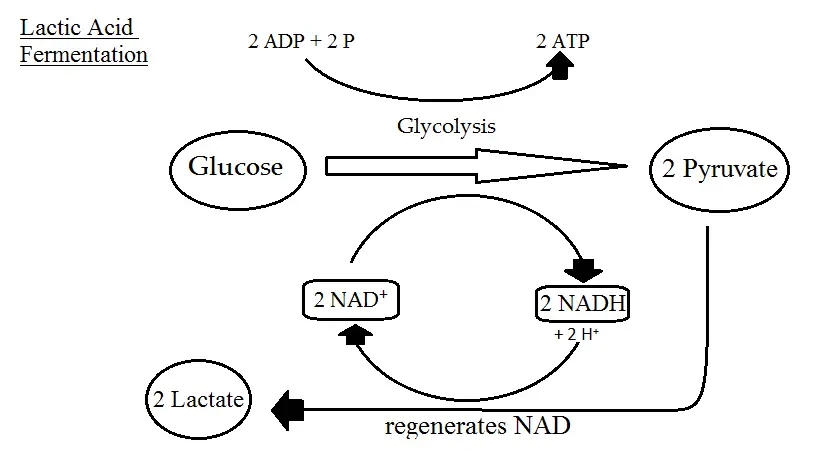
- Ethanol:
- Formation: Ethanol is produced when pyruvate, derived from glucose, is acted upon by enzymes such as pyruvate carboxylase and alcohol dehydrogenase. The overall reaction converts one glucose molecule into two ethanol and two carbon dioxide molecules.
- Applications: Ethanol fermentation is pivotal in the production of alcoholic beverages like wine and beer. Additionally, it plays a role in bread-making, where the released carbon dioxide aids in dough rising. Ethanol also serves as a biofuel, supplementing or replacing gasoline.
- Lactic Acid:
- Formation: Homolactic fermentation results in the conversion of glucose into two molecules of lactic acid. This process is facilitated by the enzyme lactate dehydrogenase.
- Applications: Lactic acid fermentation is crucial in the dairy industry, producing yogurt and certain cheeses. The sour taste of yogurt is attributed to lactic acid. Some bacteria can further metabolize lactate, producing ethanol, carbon dioxide, and other compounds.
- Acetic Acid:
- Formation: Acetic acid bacteria oxidize sugars or ethanol to yield acetic acid. The reaction involves the conversion of ethanol in the presence of oxygen to acetic acid and water.
- Applications: The commercial production of vinegar is a notable application of acetic acid fermentation.
- Hydrogen Gas:
- Formation: Certain fermentation processes result in the production of hydrogen gas, serving as a mechanism to regenerate NAD+ from NADH. The gas is produced as a byproduct in reactions involving specific bacteria.
- Applications: Hydrogen gas has potential as an energy source, and its production through fermentation is an area of active research.
- Other Products:
- Butanediol, Butyrate, Caproate, Acetone: These are products of various specialized fermentation processes. Their production and significance vary based on the microbial species and conditions.
- Alternative Protein Sources:
- Formation: Modern fermentation techniques enable the production of recombinant proteins, serving as meat analogues, milk substitutes, and egg substitutes.
- Applications: These proteins, such as recombinant myoglobin and leghemoglobin, are used in the production of meat alternatives, offering texture, flavor, and aroma akin to real meat.
- Enzymes:
- Formation: Industrial fermentation facilitates enzyme production, where microorganisms produce and secrete proteins with catalytic activity.
- Applications: Enzymes find applications across various industries, including food, beverage, baking, detergents, textiles, and pulp and paper industries.
In conclusion, the products of fermentation are diverse and have been harnessed for a multitude of applications, from food and beverages to industrial processes and medical applications. The versatility of fermentation and its products underscores its significance in biotechnology and industry.
Different Stages of the Fermentation Process
Fermentation, a complex metabolic process, undergoes distinct stages, each characterized by specific biochemical transformations and activities. These stages ensure the efficient conversion of substrates into desired products, often accompanied by the release of gases and other secondary metabolites. Here, we delineate the three primary stages of the fermentation process:
- Primary Fermentation:
- Initiation: This stage commences when yeast is introduced into aerated, cooled wort. The yeast swiftly utilizes the available oxygen to synthesize sterols, which are vital for yeast cell proliferation.
- Anaerobic Transition: Once the oxygen is exhausted, the yeast transitions to an anaerobic mode. In this phase, the majority of wort carbohydrates are metabolized into ethanol and carbon dioxide.
- Yeast Growth and Metabolite Synthesis: Concurrently, there is a proliferation of yeast cells. The synthesis of aroma and flavor compounds is directly linked to the magnitude and velocity of yeast growth during this stage.
- Secondary Fermentation:
- Metabolite Conversion: This stage is initiated when the bulk of the wort sugars have been metabolized, and the fermentation rate has substantially decelerated. Residual sugars are consumed during this phase, and the yeast begins to metabolize certain secondary metabolites.
- Yeast Flocculation: As the alcohol concentration increases and the availability of sugars and nutrients diminishes, yeast cells start to flocculate, forming clumps, and commence settling.
- Conditioning Stage:
- Initiation: This stage is triggered when the fermentation reaches its terminal gravity, and the fermentation vessel is cooled to specific chilling temperatures, typically between 31-38 °F (0-3 °C).
- Yeast Settling: The yeast continues to flocculate and settle during this period, clarifying the brew.
- Flavor Refinement: The yeast plays a pivotal role in refining the beer’s flavor by eliminating various undesirable compounds. It’s worth noting that ales, unlike lagers, benefit less from prolonged conditioning. The quintessential flavors in ales tend to diminish over time, making it prudent to minimize the conditioning duration before packaging.
- Oxygen Sensitivity: At this juncture, the beer becomes particularly susceptible to oxygen exposure, which can detrimentally affect its quality.
In summation, the fermentation process, through its distinct stages, ensures the systematic transformation of wort into beer, with each stage contributing to the flavor, aroma, clarity, and quality of the final product.
Important Factors before starting fermentation
You may want to make pickles with your vegetables or to begin the process of making your own beer, these guidelines can help you get started making.
- Establish your “starter” cultures: Microbes are present naturally in the air we breathe, but to get started with fermentation, you’ll usually need a “starter” set of cultures like the whey (from yogurt) or an Symbiotic Colony comprised of Bacteria and Yeast, or SCOBY (for Kombucha) or even liquid from an earlier ferment. These starter cultures are already filled in beneficial microorganisms. If you incorporate them into your beverage or food product They’ll grow rapidly and help kickstart the fermentation process.
- Keep your equipment clean: To stop bad bacteria from getting into your ferment it is vital to clean and disinfect your kitchen equipment and surfaces you use for your work.
- Avoid exposure: The exposure of your ferment to the air can stop the proper fermentation process from happening and increase the chance of food poisoning and spoilage. There are a variety of ways to prevent that.
- To stop fermenting food from coming in the air around it, place the food in an acid solution (brine). If you are fermenting large chunks of food, such as chops of vegetables, this technique works effectively. You can alter the pH of the process which determines the amount of oxygen will be present using vinegar in your mix.
- Storage: To prevent contamination by air, place your fermenting food in a container that is sealable. A lot of home fermenters utilize an ordinary mason jar that has lids to seal out air, however there are other options. Storage containers typically have the ability to release carbon dioxide gas that is released in the course of fermentation. If you’re determined to monitor the quality of your ferment to ensure that it doesn’t get spoiled and spoil, you can open the sealed containers manually and release carbon dioxide. (If you’re making wine, kombucha, or any other final product which benefit from carbonation, you may opt-out of carbon dioxide venting.)
- Fermentation management: By regulating the temperature of your environment and the temperature of the air, you can alter the result from your ferment. In general, microbes perform well in warm or room temperature however, the optimal temperature will depend on the kind of microbes that you’re using and the type of fermentation you’re doing. The temperature you choose to change can affect your process in a significant way. Moving your product into an environment that is cooler like an air-conditioned basement or refrigerator, can slow down the rate of fermentation , and sometimes stop it completely. The heating of a ferment in the opposite way could kill your vital microbes.
Modes of industrial operation
Industrial fermentation processes are pivotal in producing a myriad of products, from pharmaceuticals to food and beverages. The mode of operation chosen for a particular fermentation process can significantly influence its efficiency, cost, and product yield. Here, we elucidate the primary modes of industrial operation in fermentation.
- Batch Fermentation:
- Description: In batch fermentation, all ingredients are combined at the outset, and the fermentation proceeds without further additions. It is a traditional method used for millennia in bread-making and alcoholic beverage production.
- Characteristics: The process undergoes various phases, including a lag phase (cell adjustment), exponential growth phase, stationary phase (nutrient depletion), and a cell death phase. The fermentor requires sterilization between batches, which can be cost-intensive.
- Fed-batch Fermentation:
- Description: A variation of batch fermentation, fed-batch involves the incremental addition of ingredients during the fermentation process.
- Characteristics: This mode offers enhanced control over fermentation stages. By adding limited nutrients during the non-exponential growth phase, the production of secondary metabolites can be optimized. It often integrates elements of both batch and continuous operations.
- Open Fermentation:
- Description: This approach avoids the high costs associated with fermentor sterilization by employing methods resistant to contamination.
- Characteristics: Open fermentation can utilize mixed cultures, which are particularly beneficial in wastewater treatment due to their adaptability. Other strategies include employing thermophilic bacteria for lactic acid production at elevated temperatures to deter microbial contamination or using halophilic bacteria in hypersaline conditions. Solid-state fermentation, another variant, involves minimal water addition to a solid substrate.
- Continuous Fermentation:
- Description: Continuous fermentation involves the uninterrupted addition of substrates and removal of products.
- Characteristics: There are several types of continuous fermentation:
- Chemostats: Maintain constant nutrient levels.
- Turbidostats: Regulate to keep cell mass constant.
- Plug Flow Reactors: Feature a steady flow of culture medium through a tube, with cells recycled from the outlet back to the inlet.
- Advantages: If maintained effectively, continuous fermentation can offer a steady flow of feed and effluent, reducing batch setup costs. It can also extend the exponential growth phase and continuously remove inhibitory byproducts. However, maintaining a steady state can be challenging, and the design is often intricate.
In conclusion, the choice of fermentation mode is contingent upon the specific requirements of the industrial process, the nature of the product, and economic considerations. Each mode has its advantages and challenges, and the selection often involves a trade-off between operational simplicity, cost-efficiency, and product yield.
How Does Fermentation Work?
To master the art of fermentation it is necessary to know the scientific process behind it.
- Microorganisms can survive by consuming carbohydrates (sugars such as glucose) to generate energy and for fuel.
- Organic compounds such as adenosine Triphosphate (ATP) provide energy to all the cells in cells when it is needed.
- Microbes generate ATP using respiration. Aerobic respirationthat needs oxygen is the most effective method to accomplish this. Aerobic respiration starts with glycolysis, in which glucose is converted to the acid pyruvic. If oxygen is present aerobic respiration takes place.
- The process of fermentation is similar to that of anaerobic respiratory respiration, the type that occurs in the absence of oxygen available. But, it also triggers the creation of various organic molecules such as lactic acid. It produces ATP in contrast to respiration which relies on pyruvic acid.
- Based on the environmental conditions, the microbes and cells have the capability to switch between two different energy sources.
- Organisms usually obtain energy through fermentation, however, certain systems make use of sulfate to be one of the last electrons to be accepted by the electron transportation chain.
What Happens During the Fermentation Process?
Fermentation takes place when oxygen is not present (anaerobic conditions) and when there are beneficial microorganisms (yeasts and molds as well as bacteria) that get their energy via fermentation. If there is enough sugar available certain yeast cells such as Saccharomyces cerevisiae, will prefer fermentation to aerobic respiration, even when oxygen is plentiful.
- Through the process of fermentation beneficial microbes break down sugars and starches to alcohols and acids and make food more nutritious and also preserving it, allowing people to keep it for longer durations of time without it deteriorating.
- Fermentation products supply enzymes needed to digest food. This is vital because we have a limited amount of enzymes and they diminish with the aging process. Fermented foods contain enzymes that breakdown them.
- Fermentation can also help in the process of pre-digestion. In the process of fermentation the microbes consume sugars and starches and break down food before it’s eaten it.
Applications of fermentation
Fermentation, a metabolic process primarily characterized by the conversion of carbohydrates in anaerobic conditions, has been harnessed for various applications across diverse sectors. The versatility of fermentation is evident in its widespread use, from medicine to waste management. Here, we elucidate the primary applications of fermentation:
- Medical Applications:
- Antibiotics Production: Fermentation is pivotal in producing several antibiotics, which are compounds that inhibit or kill pathogenic microorganisms.
- Insulin Production: Through recombinant DNA technology, specific bacteria are engineered to produce human insulin via fermentation.
- Growth Hormones: Fermentation aids in the synthesis of certain growth hormones, essential for therapeutic purposes.
- Vaccines: Some vaccines are produced using fermentation processes, harnessing specific microorganisms or their components.
- Interferon Production: Interferons, proteins vital for immune responses, are produced using microbial fermentation.
- Food Industry Applications:
- Fermented Foods: Traditional foods like cheese, wine, beer, and bread owe their production and unique characteristics to fermentation.
- Bio-preservatives: Fermentation aids in producing food-grade preservatives that extend shelf life and maintain food quality.
- Functional Foods and Neutraceuticals: These are foods fortified with health-enhancing components, often produced through fermentation.
- Single-cell Protein Production: Fermentation is employed to produce protein-rich microbial biomass, serving as an alternative protein source.
- Environmental and Industrial Applications:
- Biofuels Production: Fermentation is instrumental in producing biofuels like biodiesel, bioethanol, butanol, and biohydrogen, offering sustainable energy alternatives.
- Bio-surfactant and Polymers Production: Certain microbial fermentations result in bio-surfactants and polymers like bacterial cellulose, which have industrial applications.
- Bioremediation: Fermentation plays a role in developing bioremediation processes, where microbes or their enzymes are used to treat contaminated soils and wastewater, restoring environmental balance.
In essence, fermentation, with its multifaceted applications, stands as a testament to the symbiotic relationship between humans and microorganisms. Its diverse applications underscore its significance in advancing medical, food, and environmental sectors.
Limitations of fermentation
Fermentation, while a versatile and widely employed process, is not without its challenges. The intricacies of microbial metabolism and the complexities of scaling up the process present several limitations. Here, we elucidate the primary constraints associated with fermentation:
- Economic and Energetic Concerns:
- Low-Scale Production: Fermentation, especially when tailored for specific high-value products, often operates on a smaller scale, which may not be economically viable for large-scale industrial applications.
- High Costs and Energy Consumption: The specialized equipment, stringent conditions, and energy-intensive operations can escalate the costs associated with fermentation processes.
- Microbial and Product Challenges:
- Contamination Risks: The introduction of undesired microorganisms can compromise the fermentation process, leading to reduced yields or product contamination.
- Natural Variations: Microbial cultures can exhibit natural variations over time, potentially affecting the consistency and quality of the end product.
- Impurity of Products: The products derived from fermentation often contain impurities or by-products, necessitating further purification steps, which can be time-consuming and costly.
- Unexpected End Products: Due to the multifaceted nature of microbial metabolism, sometimes the end products may deviate from the expected outcome, impacting the product’s quality and utility.
- Microbial Viability and Competition:
- Growth of Undesirable Microbes: In some fermentation setups, undesirable or contaminant microorganisms might outcompete the desired microbes, leading to reduced product yields or altered product profiles.
- Loss of Desired Microbes: The desired microorganisms, especially if they are sensitive to specific environmental conditions, might lose viability during the fermentation process, affecting the overall efficiency.
In summary, while fermentation offers a plethora of benefits across various sectors, it is imperative to recognize and address its limitations to optimize its applications and ensure consistent, high-quality outcomes.
Advantages of Fermentation
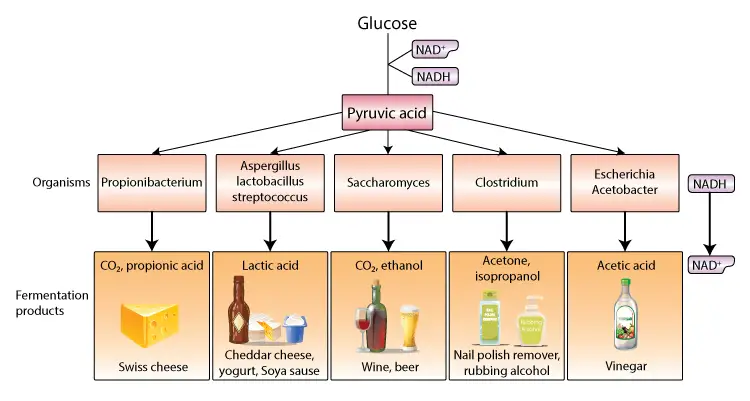
Fermentation, a time-honored metabolic process, offers a myriad of advantages, particularly in the realm of food and health. The transformation of raw materials by microorganisms not only enhances the nutritional profile of the products but also introduces beneficial compounds that can positively impact human health. Here, we delve into the primary advantages of fermentation:
- Enhancement of Gut Health:
- Probiotic Enrichment: Fermented foods are replete with probiotics, which are beneficial microorganisms that promote a healthy gut environment. A robust gut microbiota is essential for optimal nutrient absorption and overall digestive health.
- Immune System Augmentation:
- Antimicrobial Production: The gut, aided by the probiotics from fermented foods, synthesizes various antimicrobial compounds, including antibiotics, antiviral, antifungal, and anti-tumor agents. These compounds bolster the immune system, offering protection against potential pathogens.
- Creation of Unfavorable Environment for Pathogens: The acidic milieu resulting from fermentation impedes the proliferation of many harmful pathogens, ensuring gut health.
- Neutralization of Anti-Nutritional Factors:
- Phytic Acid Reduction: Fermentation can significantly reduce the levels of phytic acid, an anti-nutritional factor found in nuts, seeds, grains, and legumes. Phytic acid can bind essential minerals, rendering them unavailable for absorption, and potentially leading to mineral deficiencies. By neutralizing phytic acid, fermentation enhances the bioavailability of these minerals.
- Improved Digestibility: The reduction of phytates also enhances the digestibility of starches, fats, and proteins present in the food.
- Augmentation of Nutritional Content:
- Vitamin Enhancement: Fermentation can elevate the levels of certain vitamins, particularly those of the B-complex group, such as folic acid, riboflavin, thiamin, niacin, and biotin. Additionally, some fermented foods may also witness an increase in vitamin C content.
- Enhanced Mineral Absorption: The presence of probiotics, enzymes, and lactic acid in fermented foods facilitates the absorption and utilization of essential minerals, ensuring that the body derives maximum nutritional benefit.
In essence, fermentation not only amplifies the nutritional value of foods but also introduces compounds that can profoundly influence human health. The myriad benefits underscore the significance of incorporating fermented foods into a balanced diet.
Fermentation Facts
Fermentation, a metabolic process, has intrigued scientists and researchers for its unique characteristics and widespread occurrence in nature. Here, we present a compilation of salient facts about fermentation:
- Anaerobic Nature: Fermentation is inherently an anaerobic process, implying that it transpires in the absence of oxygen. This characteristic distinguishes it from aerobic respiration, which necessitates oxygen for energy production.
- Yeast’s Preference: Interestingly, even in oxygen-rich environments, yeast cells exhibit a predilection for fermentation over aerobic respiration, provided there is an ample supply of sugar. This showcases the adaptability and versatility of these unicellular organisms in energy production.
- Human Digestive System: Fermentation is not exclusive to microorganisms. It also transpires within the human digestive system, aiding in the breakdown of complex molecules.
- Gut Fermentation Syndrome: A rare yet intriguing medical condition known as gut fermentation syndrome or auto-brewery syndrome manifests when fermentation within the human digestive tract culminates in the production of ethanol. This endogenous ethanol production can lead to symptoms of intoxication in the affected individual.
- Muscle Cell Fermentation: Human muscle cells, under strenuous conditions, can resort to fermentation for energy production. When muscles are exerted beyond the oxygen supply rate, they derive ATP through glycolysis, an anaerobic process, ensuring continuous energy supply even in oxygen-deprived conditions.
- Beyond Fermentation: While fermentation is a prevalent anaerobic pathway, it’s worth noting that it’s not the sole anaerobic mechanism for energy derivation. Certain biological systems employ sulfate as the terminal electron acceptor in their electron transport chains, showcasing the diversity of anaerobic energy production mechanisms.
In summation, fermentation is a multifaceted process with a myriad of applications and manifestations across different organisms. Its ability to produce energy anaerobically underscores its evolutionary significance and its pivotal role in the survival and functioning of various organisms.
Comparison of Fermentation, Anaerobic Respiration, and Aerobic Respiration
The processes of fermentation, anaerobic respiration, and aerobic respiration are vital metabolic pathways that cells employ to generate energy. While they share certain similarities, they also exhibit distinct differences in terms of mechanisms, electron transport, ATP yield, and end products. Here, we present a comprehensive comparison of these three processes:
- Nature of the Process:
- Fermentation: An anaerobic process that does not utilize the electron transport chain.
- Anaerobic Respiration: An anaerobic process that employs the electron transport chain to transfer electrons to the final electron acceptor.
- Aerobic Respiration: An aerobic process that uses the electron transport chain, with oxygen serving as the final electron acceptor.
- ATP Production:
- Fermentation: Yields 2 ATP molecules per glucose molecule, exclusively through substrate-level phosphorylation.
- Anaerobic Respiration: The ATP yield varies depending on the specific pathway and organism.
- Aerobic Respiration: Produces approximately 38 ATP molecules per glucose molecule, through both substrate-level phosphorylation and oxidative phosphorylation.
- Final Electron Acceptor:
- Fermentation: Organic molecules, such as pyruvate in lactic acid fermentation or acetaldehyde in alcohol fermentation.
- Anaerobic Respiration: Inorganic compounds like sulfate ion (SO₄²⁻), nitrate (NO₃⁻), and ferric ion (Fe³⁺) or certain organic compounds like dimethyl sulfoxide and fumarate.
- Aerobic Respiration: Oxygen (O₂) acts as the final electron acceptor.
- End Products:
- Fermentation: Products include lactic acid, alcohol, hydrogen gas, and CO₂.
- Anaerobic Respiration: The end products vary. For instance, denitrification produces N₂, sulfate respiration yields HS⁻, and methanogenesis results in methane.
- Aerobic Respiration: The primary end products are water (H₂O) and carbon dioxide (CO₂).
- Examples:
- Fermentation: Processes include lactic acid fermentation, alcohol fermentation, and various other fermentative pathways.
- Anaerobic Respiration: Examples encompass denitrification, fumarate respiration, sulfate respiration, and methanogenesis, among others.
- Aerobic Respiration: Encompasses pathways like glycolysis followed by acetyl-CoA metabolism and fatty acid catabolism (beta-oxidation) coupled with acetyl-CoA metabolism.
In summary, while fermentation, anaerobic respiration, and aerobic respiration are all mechanisms to harness energy, they differ in their electron transport systems, ATP yields, electron acceptors, and resultant products. Understanding these differences is crucial for comprehending the diverse metabolic strategies organisms employ to thrive in various environments.
Criteria | Fermentation | Anaerobic Respiration | Aerobic Respiration |
---|---|---|---|
Nature of the Process | Anaerobic (without electron transport chain) | Anaerobic (with electron transport chain) | Aerobic (with electron transport chain) |
ATP Production | 2 ATP per glucose (substrate-level phosphorylation) | Varies | ~38 ATP per glucose (substrate-level and oxidative phosphorylation) |
Final Electron Acceptor | Organic molecules (e.g., pyruvate, acetaldehyde) | Inorganic compounds (e.g., SO₄²⁻, NO₃⁻, Fe³⁺) or certain organic compounds | Oxygen (O₂) |
End Products | Lactic acid, alcohol, hydrogen gas, CO₂ | Varies (e.g., N₂ in denitrification, HS⁻ in sulfate respiration, methane in methanogenesis) | Water (H₂O) and carbon dioxide (CO₂) |
Examples | Lactic acid fermentation, alcohol fermentation, etc. | Denitrification, fumarate respiration, sulfate respiration, methanogenesis, etc. | Glycolysis + acetyl-CoA metabolism, fatty acid catabolism (beta-oxidation) + acetyl-CoA metabolism, etc. |
Examples of Fermentation
Fermentation is a metabolic process that converts sugar to acids, gases, or alcohol. It occurs in yeast and bacteria, and also in oxygen-starved muscle cells, as in the case of lactic acid fermentation. Here are some common examples of fermentation:
- Alcoholic Fermentation:
- Beer: Yeast ferments the sugars present in malted grains to produce alcohol and carbon dioxide.
- Wine: Yeast ferments the sugars in grapes.
- Sake: Fermentation of rice by fungi and yeast.
- Lactic Acid Fermentation:
- Yogurt: Specific strains of bacteria convert lactose in milk to lactic acid.
- Cheese: Various bacteria ferment milk to produce different types of cheeses.
- Sauerkraut: Fermentation of cabbage by lactic acid bacteria.
- Kimchi: Fermented vegetables, primarily napa cabbage and Korean radishes, with chili pepper, garlic, ginger, and jeotgal (salted seafood).
- Pickles: Cucumbers fermented in a solution of salt and water.
- Acetic Acid Fermentation:
- Vinegar: Acetic acid bacteria ferment alcoholic beverages to produce vinegar.
- Mixed Acid Fermentation:
- Kombucha: A fermented tea drink produced by fermenting sweetened tea with a symbiotic culture of bacteria and yeast (SCOBY).
- Butyric Acid Fermentation:
- Certain cheeses and butter have a characteristic flavor due to butyric acid produced by fermentation.
- Propionic Acid Fermentation:
- Used in the production of Swiss cheese, giving it its characteristic holes and flavor.
- Fermentative Hydrogen Production:
- Some bacteria produce hydrogen gas as a byproduct of fermentation.
- Bread Making:
- Yeast ferments the sugars in dough, producing carbon dioxide, which makes the bread rise.
- Traditional African Beverages:
- Drinks like “ogi” and “ukodo” are produced by fermenting cereals.
- Asian Fermented Foods:
- Miso: A Japanese seasoning produced by fermenting soybeans with salt and koji (the fungus Aspergillus oryzae).
- Tempeh: An Indonesian product made from fermented soybeans.
- Nattō: Japanese fermented soybeans known for their strong smell and sticky texture.
These are just a few examples, and many cultures around the world have their unique fermented foods and beverages that have been integral to their culinary traditions for centuries.
Quiz
What is the primary product of fermentation in yeast?
a) Lactic acid
b) Ethanol
c) Methane
d) Acetic acid
Which of the following is NOT a product of fermentation?
a) Oxygen
b) Carbon dioxide
c) Ethanol
d) Lactic acid
In which food is lactic acid fermentation primarily used?
a) Bread
b) Wine
c) Yogurt
d) Beer
What is the main purpose of fermentation in bread making?
a) To add flavor
b) To produce alcohol
c) To make the bread rise
d) To preserve the bread
Which organism is primarily responsible for alcoholic fermentation?
a) Lactobacillus
b) E. coli
c) Saccharomyces cerevisiae
d) Acetobacter
Which of the following is a product of lactic acid fermentation in muscles?
a) Ethanol
b) Methane
c) Lactic acid
d) Hydrogen gas
What is the primary role of yeast in wine production?
a) Flavoring
b) Preservation
c) Fermentation
d) Coloring
Which of the following is NOT a benefit of fermented foods?
a) Enhanced nutrient absorption
b) Longer shelf life
c) Increased sugar content
d) Probiotic enrichment
In which process is acetic acid primarily produced?
a) Alcoholic fermentation
b) Lactic acid fermentation
c) Acetic acid fermentation
d) Butyric acid fermentation
Which of the following beverages is made by fermenting tea using a symbiotic culture of bacteria and yeast?
a) Beer
b) Wine
c) Kombucha
d) Cider
FAQ
What is fermentation?
Fermentation is a metabolic process that converts carbohydrates, such as sugars and starches, into chemical compounds like alcohol and organic acids using microorganisms like yeast and bacteria.
What are the main products of fermentation?
The main products of fermentation include ethanol (alcohol), lactic acid, carbon dioxide, and various organic compounds, depending on the type of fermentation and microorganisms involved.
Why is fermentation important?
Fermentation is essential in various industries, including food and beverage production, pharmaceuticals, and biofuel manufacturing. It is used to produce a wide range of products, from bread and yogurt to bioethanol and antibiotics.
What is the difference between aerobic and anaerobic fermentation?
Aerobic fermentation occurs in the presence of oxygen, while anaerobic fermentation occurs in the absence of oxygen. In aerobic fermentation, oxygen is the final electron acceptor, while in anaerobic fermentation, other molecules serve as electron acceptors.
How does fermentation contribute to food preservation?
Fermentation helps preserve food by creating an acidic or alcoholic environment that inhibits the growth of harmful microorganisms. This extended shelf life is a result of the production of organic acids, alcohol, and other antimicrobial compounds during fermentation.
Can humans perform fermentation?
Yes, humans can undergo fermentation in their muscles during strenuous exercise when oxygen supply becomes limited. This process produces lactic acid, causing muscle fatigue and soreness.
What are probiotics, and how are they related to fermentation?
Probiotics are beneficial live microorganisms that can improve gut health. Many fermented foods, like yogurt and kimchi, contain probiotics naturally, making them popular choices for maintaining a healthy gut microbiome.
What is the role of yeast in fermentation?
Yeast is a microorganism commonly used in alcoholic fermentation. It consumes sugars and produces ethanol and carbon dioxide as byproducts. This process is utilized in brewing and winemaking.
Are there any health benefits associated with consuming fermented foods?
Yes, fermented foods can have health benefits. They can improve digestion, enhance nutrient absorption, and contribute to a balanced gut microbiota, which is linked to overall health and well-being.
What are some examples of fermented foods and beverages?
Examples of fermented foods and beverages include yogurt, sauerkraut, sourdough bread, kefir, cheese, wine, beer, and kombucha. These products undergo fermentation to develop their distinctive flavors and properties.