A game changer for the microscopic world is epi-fluorescence microscopy. Imagine being able to see cells as vibrant, dynamic creatures showing off their inner workings instead of just as static objects. This method, based on the principle of fluorescence, has opened up the hidden details of life at the cellular level and made the invisible visible in a really cool way.
At its heart epi-fluorescence microscopy is all about shining a light on a specimen with precise wavelengths of light which then excites the fluorescent molecules, or fluorophores, in the sample. These fluorophores emit longer wavelength light which stands out against the dark background. Like turning on a neon sign in a black room the fluorescence draws your attention to proteins, organelles or cells. Many of the tiny things we look at now would be hidden in the shadows without this approach.
But how does it work? A mercury arc lamp or LED, the microscope shines light from a high energy source through a series of filters and mirrors to separate the wavelengths that excite the fluorophores. Before it hits the eyepiece or camera the light hits the sample and passes through several filters to emit fluorescence. Sounds complicated and it is, but the beauty is in how all these bits come together to produce an image that shows molecular level detail.
The flexibility of epi-fluorescence microscopy is cool. Want to learn the kinetics of a protein in a live cell? Tag it with a fluorescent marker like GFP and watch it move in real time. Studying tissue organisation? Multi colour labelling turns a sample into a beautiful mosaic of biological activity by letting several structures light up in different colours. The possibilities are endless and researchers are pushing the boundaries of what this technique can do.
Epi-fluorescence microscopy has its quirks even if it has its benefits. One of the problems is photobleaching where the fluorophores lose their fluorescence after extended light exposure. Like watching a candle burn down the light fades and you wish it would last just a little bit longer. And background noise from out of focus fluorescence can obscure the image; so advanced methods like confocal microscopy have become important to address this limitation.
Epi-fluorescence microscopy always amazes whether you’re a seasoned researcher trying to solve the mysteries of disease or a beginner looking at a first fluorescently labelled slide. It reminds us how light can be used to illuminate the darkest bits of biology and make new discoveries. Who knew you could uncover the secrets of life by just turning on a light?
Principle of Epi-Fluorescence Microscopy
Epi-fluorescence microscopy excites fluorescent molecules with strong light instead of visible light. Fluorophores absorb photons that activate electrons. These electrons emit longer-wavelength light when they return to their ground state. The microscope filters this emitted light from the excitation light to magnify the specimen.
The working mechanism has multiple critical steps. An excitation filter—a wavelength-selective bandpass filter—generates UV or visible spectrum wavelengths from source light. Select light wavelengths reflect off the dichromatic mirror and illuminate the item intensely. Light from fluorescence passes through a dichromatic mirror and an emission filter. After filtering out undesired excitation wavelengths, a CCD camera or equivalent detector displays the image.
Like most microscopes, this method targets fascinating specimens. Emission selectively highlights fluorescent objects while filtering out excitation light is its main benefit. This selective visualisation lets researchers study specific structures or chemicals in complex biological samples.
Parts of Epi-Fluorescence Microscope
- Light Source: An effective light system from which a wide range of wavelengths can come out is very important. Common sources are Tungsten-Halogen lamps, Mercury and xenon arc systems, Metal Halide lights like Chroma Tech-Photofluor and Zeiss HXP 120, LEDs, and Monochromators.
- Objective lens – This critical part sends light to the samples, which makes them look bigger. The brightness of the lens depends on numerical aperture and magnification. Phase contrast objectives are not the best because their phase ring stops the fluorescence emissions, but DIC objectives work very well if you take out the prism and analyser from the path.
- Filters– These special parts filter out certain wavelengths. The setup has an excitation filter that chooses certain wavelengths, and a dichroic mirror (or beamsplitter) separates the excitation and emission light. The dichroic reflects shorter wavelengths of excitation and allows longer wavelengths of emission to pass through. It also has the emission filter which filters out unwanted excitation light. Soft-coated filters and mirrors usually provide high-res images.
- Cameras – CCDs of good quality take excellent images of specimens rapidly and with high sensitivity. Color cameras are to be avoided, though, since they have low resolution; EMCCD systems are highly useful for recording fast events. These devices are really good for special uses requiring quick image capturing.
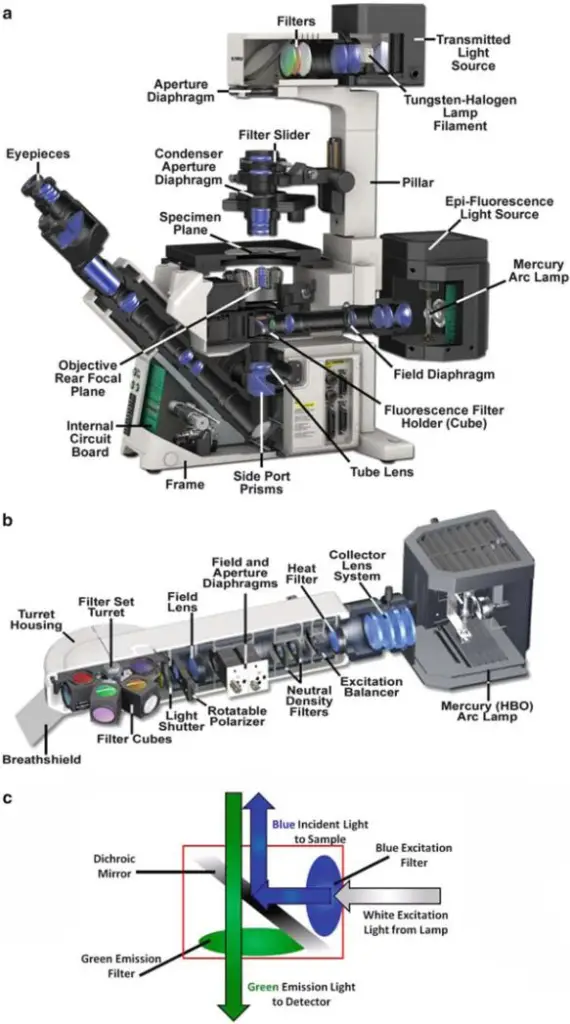
Sample Preparation for Epi-Fluorescence Microscopy
Usually seen with the Epi-fluorescence microscope are thick samples, such as those of 10µm depth. Combining the strong illumination with the activation of molecules outside the focal point could produce a background signal that may be significantly more important than other illumination techniques as TIRF and HILO.
The following describes the steps of preparation for this kind of microscopy:
- First the grown in vitro cells are adhered onto the coverslips using suitable incubation. Different proteins are incubated in particular solutions under precisely regulated temperature.
- The culture then three times rinsed in phosphate-buffered saline (PBS). After that, 0.2% Triton X-100 permeables the cells at room temperature for five minutes.
- The cells are then once more washed, three more times using PBS. Wet Kimwipes within the incubation chamber help to maintain the coverslips in a humid atmosphere so preventing their drying out.
- To inhibit nonspecific binding, the sample then is incubated for one hour at room temperature using 100 μl of 20% goat serum.
- The main antibody is then produced, diluted in 5% goat serum at 1:100 ratio. This is flexible; depending on the type of the antibody, some may need a lower or greater concentration.
- The coverslips—which now contain the sample—are next treated with 100 μl of primary antibody, once more at room temperature for one hour or, if preferred, at 4°C overnight.
- The coverslips are three times rinsed in PBS following incubation.
- Made in five percent goat serum, a fluorophore-conjugated secondary antibody is diluted at 1:500. Stored in darkness, this antibody should avoid any breakdown. Beforehand testing the concentration of this antibody guarantees low nonspecific labelling and maximum specificity.
- Incubation with 100 μl of the secondary antibody should then take at room temperature for one hour. Avoiding overnight incubation of the sample helps to prevent nonspecific binding. The sample should once this stage is finished once again be rinsed three times using PBS.
- The microscope slide then has 10 μl of mounting fluid including an anti-fade agent such as ThermoFisher added to it. The coverslip is then flipped onto the slide and, using a cotton swab, care must be taken to gently press it down to prevent trapping air bubbles.
- At last, the coverslips are sealed in a 1:1:1 ratio Vaseline, Lanolin, and Paraffin solution. The growing media aids in both hardening and curing of the specimen. The sample will be long-term preserved if this mounted specimen is kept in the dark at 4°C.
Operating Procedure of Epi-Fluorescence Microscopy
Epi-fluorescence microscopy’s operating technique is somewhat similar to that of the conventional fluorescence microscope. The following constitute the steps:
- First the mercury lamp is turned on while the operating room’s lights are turned out. Waiting roughly fifteen minutes will help the microscope to reach its maximum brightness since it guarantees the fully powered light source.
- The filter is then chosen based on the fluorescein used in the sample. This choice is absolutely important since it guarantees that the sample will be lit with the right wavelength, therefore maximising the fluorescence effect.
- The slide with the sample solution then is gently positioned on the stage and fastened with clips. This guarantees a steady sample that won’t change under observation.
- The focus is changed to produce a crisp image using both the fine and coarse knobs after the sample is in place. One can make fine changes to obtain a sharp image at great magnification power.
- The camera eyepiece is now fastened to the microscope to take the pictures of the sample. Documenting the seen sample or distributing the view to others in the lab depend on this.
- Usually, it is advisable to turn the focus off from the control box if the microscope is operated for about thirty minutes to avoid needless system strain. The mercury lamp thereafter gets switched off in order to save electricity.
- At last, the microscope is unplugged and gently returned to cover it so that it is kept free from damage and dust.
Applications of Epi-Fluorescence Microscopy
- The epi-fluorescence microscope is essential in cell biology, microbiology, pathology, biochemistry, and neurosurgery. Like other microscopes, it is essential for research and therapeutic use.
- This microscope focusses on cellular component structure, localisation, dynamics, and functions. These components can be investigated in live cells and real-time cellular activity. Tracking processes as they unfold helps understand cellular life’s complexity.
- Next, epi-fluorescence microscopy helps identify and observe microorganisms. Microbiology researchers need it to easily investigate microbial life since it highlights certain structures.
- This microscope also monitors and quantifies molecules in immunoassays. These immunoassays may detect chemicals, revealing biological processes.
- It can detect and research diseases and contaminants. This makes it useful in medical research and diagnostics, where illness processes and purity levels are crucial for therapy and further study.
Advantages of Epi-Fluorescence Microscopy
- This method offers great sensitivity in imaging and detecting certain molecules. Using fluorescent markers lets one observe low-abundance compounds that could otherwise be undetectable with conventional techniques.
- Epi-fluorescence microscopy provides real-time live cell viewing capability, much as most microscopes do. For cellular biology research, this makes the study of dynamic processes such protein localisation, cellular motions, and other activities as they occur important.
- Multiple fluorescence channels used at once allows one to concurrently see and examine many distinct components. Examining complicated systems where several targets must be investigated in parallel requires this very helpful.
- Then, compared to other imaging techniques, which might be labour-intensive or time-consuming, epi-fluorescence microscopy presents rather simple and quick sample preparation. This lets researchers process more samples in less time, hence raising output.
- When researching very small structures, it can especially help. It is perfect for visualising sub-cellular structures that could be too small to clearly resolve with other imaging techniques, including a standard stereoscopic microscope, given its great magnification capacity.
- Moreover, this microscopy technique is a great alternative for long-term live cell imaging as low phototoxicity and great resolution define it. Extended periods of observation of cells free from the harm linked with other methods guarantees the integrity of the sample.
- Generally speaking, epi-fluorescence microscopy exposes the materials to less possibly harmful light than some other fluorescence techniques since it calls for less light intensity. When handling delicate or sensitive specimens in particular, this is quite beneficial.
Limitations of Epi-Fluorescence Microscopy
- Epi-fluorescence microscopy is limited by background signal. Intense lighting activates molecules outside the focus point, scattering light and blurring pictures. This issue is worse than with TIRF or HILO, which reduce background signal.
- The limited spatial resolution compared to other advanced microscopy techniques is another problem. Epi-fluorescence microscopy is suitable for many applications, although it is not perfect for examining sub-cellular features at high resolution.
- Thick tissue sections can potentially be obscured by the microscope’s depth of focus. Images of thick specimens (>10 µm) may be obscured due to the focal plane not covering the entire depth, obscuring interior details.
- Due to prolonged light exposure, photobleaching might reduce the fluorescence signal. Working with fluorescent dyes or light-sensitive probes reduces image quality during long imaging durations.
- Epi-fluorescence microscopy, like most fluorescence-based methods, uses fluorescent probes, which can cause artefacts or nonspecific binding if not regulated. Misinterpretation of results or erroneous data can occur from these concerns.
- In samples with uneven or low fluorescence, fluorescence intensity estimation might be problematic. This reduces its reliability for quantitative analysis.
- The approach requires fluorescent dyes or markers, another drawback. For some compounds, producing or finding appropriate probes can be time-consuming and expensive.
Precautions
- Above all, it is essential to handle mercury lamps carefully when working with them. If appropriate eye protection is not used, the powerful ultraviolet (UV) radiation emitted by these lamps can seriously harm eyes. Always use UV-blocking goggles or shields to shield your eyes from dangerous rays.
- Furthermore, even with protective eyewear, it is imperative to avoid looking directly at the light source because prolonged exposure to bright light can cause eye strain or irreversible damage. Make sure the light path is always appropriately shielded or redirected out of your field of vision.
- Because many of these compounds are dangerous, using fluorescent dyes and probes needs caution. Always handle such materials with the proper gloves, lab coats, and fume hood if needed. To avoid any negative health impacts, stay away from the dyes and avoid breathing in fumes.
- Furthermore, adequate ventilation needs to be guaranteed, particularly while handling volatile or dangerous compounds. It’s crucial to operate in a well-ventilated environment or fume hood when working with fluorophores because some of them can break down when exposed to heat or light, creating hazardous byproducts.
- Then, while utilising fluorescent markers during sample processing, steer clear of too much strong light exposure. Long-term lighting can cause photobleaching, which reduces the fluorescence signal’s intensity and may produce unreliable results. When working with delicate fluorescent dyes, which need to be shielded from light exposure as much as possible, this is especially true.
- Furthermore, careful handling of the microscope is required to prevent harm to delicate parts. For instance, to guarantee optimum performance and avoid contamination, focus changes should be made carefully and the microscope should be maintained clean.
- The mercury light in the microscope should only be used within the duration and temperature ranges that are stated. The lamp may malfunction or possibly break due to overheating, which could result in dangerous circumstances. Give the light enough time to cool down before shutting it off.
- Finally, to avoid dust or debris buildup, disconnect the microscope after usage and cover it with a protective cover. To guarantee that every component, including the mercury lamp, filters, and optics, is in good operating order, routine maintenance should be performed.
- https://oni.bio/nanoimager/super-resolution-microscopy/epifluorescence-microscopy/
- https://pmc.ncbi.nlm.nih.gov/articles/PMC3483677/
- https://en.wikipedia.org/wiki/Fluorescence_microscope
- https://www.microscopyu.com/techniques/fluorescence/introduction-to-fluorescence-microscopy
- https://www.leica-microsystems.com/science-lab/life-science/multi-wavelength-epi-illumination-in-fluorescence-microscopy/
- https://anatomypubs.onlinelibrary.wiley.com/doi/10.1002/ar.22548