What is Embryo Development in Plants?
- Embryo development in plants, also known as plant embryogenesis, is a critical phase in the plant life cycle, beginning immediately after fertilization of the ovule. This process marks the transformation of a fertilized egg, or zygote, into a fully formed embryo. The journey from zygote to mature embryo is essential, setting the foundation for subsequent stages such as germination and dormancy.
- After fertilization, the zygote undergoes a series of divisions and differentiations. These cellular processes gradually shape the zygote into a mature embryo, which is composed of key structures. These include the hypocotyl, shoot apical meristem, root meristem, root cap, and cotyledons. Each of these components plays a distinct role in the future growth and development of the plant.
- One of the unique aspects of plant embryogenesis is that, unlike in animals, the resulting embryo is relatively simple. It lacks complex structures such as leaves, stems, or reproductive organs at this early stage. Despite this simplicity, both plant and animal embryos pass through a critical phase known as the phylotypic stage. This stage imposes constraints on morphological diversification, thereby guiding the independent evolution of plants and animals.
- As the embryo continues to develop, it progresses through stages that differ depending on whether the plant is a dicot or a monocot. Up to the globular embryo stage, the development process is similar in both groups. However, after this stage, dicots and monocots diverge in their embryonic development, reflecting their distinct evolutionary pathways.
Development of the embryo (Embryogenesis)
The development of the embryo in angiosperms, known as embryogenesis, is a complex and highly regulated process that transforms a diploid oospore (zygote) into a mature embryo. This process involves several key stages and can be categorized into different types based on the patterns of cell division and the roles of various cells. Below is an outline of the stages and types of embryogenesis:
- Initiation of Embryogenesis:
- Zygote Division: The process begins with the division of the diploid oospore or zygote. This division results in the formation of a two-celled proembryo.
- Formation of Proembryo: The first division is transverse, creating a basal cell and a terminal cell. The basal cell is positioned near the micropyle and often develops into the suspensor, while the terminal cell, facing towards the center of the embryo sac, is responsible for further development of the embryo.
- Roles of Basal and Terminal Cells:
- Basal Cell: The basal cell forms the suspensor, which anchors the embryo to the embryo sac and facilitates nutrient transfer. This cell may or may not contribute significantly to the formation of the embryo proper.
- Terminal Cell: The terminal cell, also known as the apical or embryo cell, is crucial for the subsequent development of the embryo. It gives rise to the majority of the embryo structures.
- Types of Embryogenesis:
- Onagrad or Crucifer Type:
- Examples: Found in families such as Annonaceae, Brassicaceae, Onagraceae, Pedaliaceae, Ranunculaceae, and Scrophulariaceae.
- Characteristics: This type features specific patterns of cell division and organization leading to a mature embryo.
- Asterad Type:
- Examples: Includes families such as Asteraceae, Balsamiaceae, Violaceae, and Vitaceae.
- Characteristics: Distinguished by unique developmental sequences and cellular arrangements.
- Solanad Type:
- Examples: Seen in families like Campanulaceae, Linaceae, Solanaceae, and Theaceae.
- Characteristics: Exhibits particular patterns of embryonic development and differentiation.
- Caryophyllad Type:
- Examples: Found in Caryophyllaceae, Crassulaceae, and Haloragaceae.
- Characteristics: Characterized by specific developmental processes and structural features.
- Chenopodiad Type:
- Examples: Includes Boraginaceae and Chenopodiaceae.
- Characteristics: Features distinct embryogenic patterns and cell functions.
- Piperad Type:
- Examples: Observed in families such as Loranthaceae and Piperaceae.
- Characteristics: Defined by unique developmental stages and cellular dynamics.
- Onagrad or Crucifer Type:
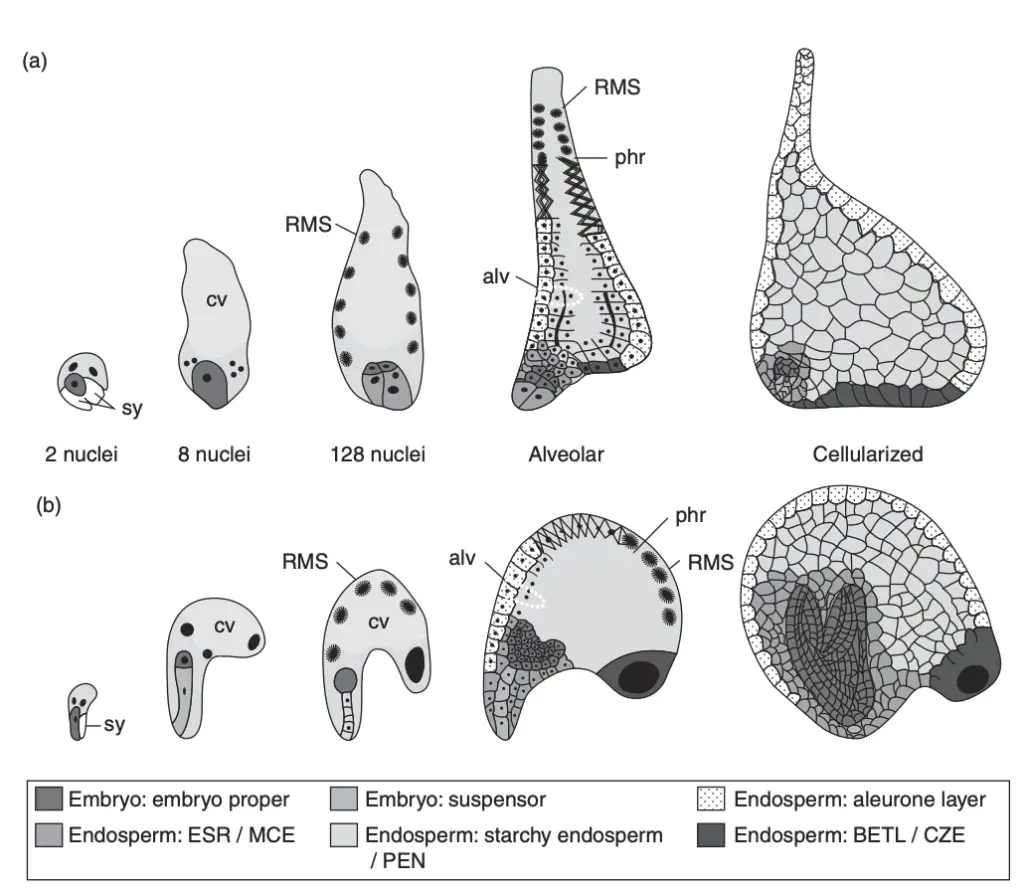
Types of embryogeny
Embryogenesis in flowering plants exhibits considerable diversity, reflected in the various types of embryogeny observed across different plant families. These types are classified based on the role and contribution of the basal and terminal cells during the early stages of embryo development. The following are the key types of embryogeny:
- Crucifer or Onagrad Type:
- Characteristics: In this type, the basal cell has minimal or no involvement in the development of the embryo. The embryogenesis is primarily driven by the terminal cell.
- Examples: This type is observed in families such as Brassicaceae (Cruciferae), Annonaceae, Scrophulariaceae, Ranunculaceae, and Onagraceae.
- Function: The basal cell often does not contribute significantly to embryo development, which simplifies the developmental process to the terminal cell’s actions.
- Asterad Type:
- Characteristics: Both the basal and terminal cells play significant roles in the embryo’s development. The terminal cell undergoes division through a transverse wall, and the basal cell contributes minimally or not at all.
- Examples: This type is found in Asteraceae, Balsaminaceae, and Vitaceae.
- Function: The involvement of both cell types allows for a more complex embryo formation, though the basal cell’s role is comparatively minor.
- Solanad Type:
- Characteristics: The basal cell usually forms a suspensor consisting of two or more cells, which aids in anchoring the embryo and facilitating nutrient transfer.
- Examples: This type is typical in Solanaceae, Campanulaceae, and Linaceae.
- Function: The suspensor formed by the basal cell is crucial for supporting the embryo and ensuring its proper development.
- Caryophyllod Type:
- Characteristics: In this type, the basal cell continues to divide, contributing more actively to the development of the embryo.
- Examples: Found in Caryophyllaceae, Crassulaceae, and Fumariaceae.
- Function: The continued division of the basal cell plays a significant role in shaping the embryo and its surrounding structures.
- Chenopodiad Type:
- Characteristics: Both basal and terminal cells are involved in the development of the embryo. This collaborative involvement results in a more intricate formation process.
- Examples: This type is observed in Chenopodiaceae and Boraginaceae.
- Function: The combined contribution of both cells facilitates a comprehensive development of the embryo, integrating their roles into the formation of various embryonic structures.
Structure of Dicot Embryo
The structure of a dicot embryo is a well-organized system that sets the stage for the plant’s development. The following are the key components of a typical dicot embryo, presented in a detailed and sequential manner:
- Embryonal Axis:
- The embryonal axis is the central structure of the embryo, connecting various parts. It serves as the framework from which the main organs of the plant develop.
- Cotyledons:
- Two Embryonic Leaves: These are the cotyledons, which are the first leaves of the plant. They are attached to the embryonal axis and serve as the primary source of nutrition for the young seedling.
- Reserve Food Material: The cotyledons store nutrients that support the growth of the seedling during germination and early development.
- Plumule:
- Embryonic Shoot Apex: The plumule is the part of the embryonal axis above the cotyledons. It is the embryonic shoot apex, which will eventually develop into the plant’s shoot system, including the stems and leaves.
- Radicle:
- Embryonic Root Apex: The radicle is located below the cotyledons on the embryonal axis. It is the embryonic root apex and will give rise to the plant’s root system upon germination.
- Hypocotyl:
- Axis Below Cotyledons: The hypocotyl is the section of the embryonal axis below the cotyledons and above the radicle. This region is crucial in positioning the radicle during germination, allowing it to penetrate the soil and establish the root system.
- Epicotyl:
- Axis Above Cotyledons: The epicotyl is the part of the embryonal axis above the cotyledons and below the plumule. It plays a vital role in the early elongation of the shoot during seedling emergence.
- Root Cap:
- Protective Structure: The root cap is found at the tip of the radicle. It protects the delicate root tip as it grows and pushes through the soil.
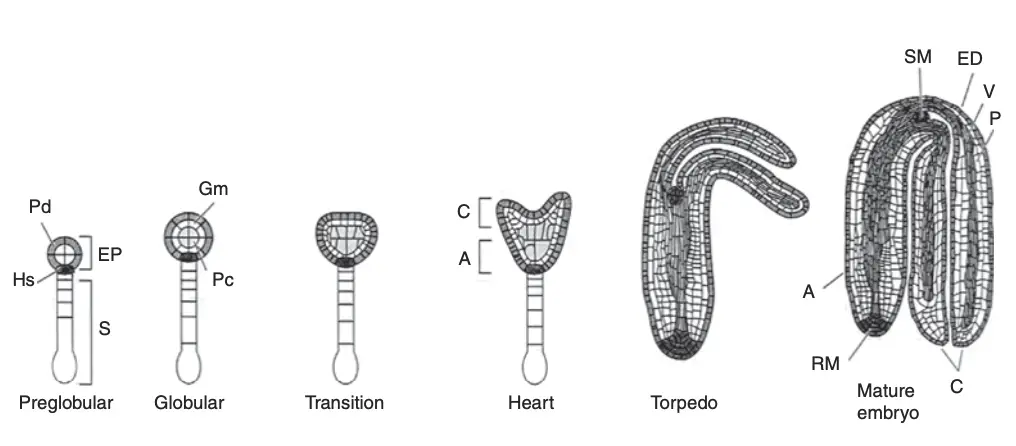
Arabidopsis thaliana. Initially this cell divides to form an apical and a basal cell (not shown); the former
divides to become the embryo (EP) and the latter the suspensor (S), as is evident at the preglobular stage. The protoderm (Pd) develops into the epidermal layer (Ed) of the mature embryo, the ground meristem (Gm) into the storage parenchyma (P), the procambium (Pc) into the vascular tissue (V) and the hypophysis (Hs) into the root and shoot meristems (RM, SM). A, axis; C, cotyledons. (From Bewley et al., 2000.) Reproduced with permission of the American Society of Plant Biologists. | Image Source: DOI: 10.1079/9781780641836.0001
Development Stages of Dicot Embryo (Development (Crucifer type)
The development of the crucifer type embryo, often observed in plants belonging to the family Brassicaceae, follows a well-defined sequence of cellular divisions and differentiation. This process is characterized by the following stages:
- Asymmetrical Zygote Division:
- Initial Division: The first division of the zygote is typically asymmetrical, occurring transversely to the long axis of the cell. This division produces two distinct cells: a small apical cell and a larger basal cell.
- Formation of the Proembryo:
- Cell Division: The apical cell undergoes vertical division, while the basal cell divides transversely. These divisions result in a four-celled structure known as the proembryo, which adopts a T-shaped configuration.
- Further Division: The three upper cells of the proembryo continue to divide through both anticlinal and periclinal divisions, leading to the formation of a 32-celled globular proembryo. It is within this structure that organogenesis, or the development of various embryo organs, begins.
- Dermatogen Formation:
- Epidermis Development: The outermost layer of cells in the globular proembryo differentiates into a distinct layer called the dermatogen. This layer will eventually develop into the epidermis of the plant, serving as the protective outer covering.
- Differentiation of Embryonic Structures:
- Cotyledons and Plumule: The inner cells of the upper region of the proembryo differentiate into cotyledons and the plumule, which will form the first leaves and the shoot apex, respectively.
- Radicle Development: The lower cells of the proembryo contribute to the formation of the radicle, which will develop into the plant’s root system.
- Heart-Shaped Embryo Formation:
- Rapid Cell Division: The cells destined to form the cotyledons divide more rapidly than those forming the plumule. As a result, the embryo gradually assumes a heart-shaped structure, with the cotyledons enclosing the plumule at the base as they fully develop.
- Suspensor Formation:
- Role of the Basal Cell: The basal cell, although crucial in the early stages, does not contribute to the final structure of the embryo. Instead, it may undergo a few transverse divisions to form a filamentous structure called the suspensor. This suspensor plays a vital role by anchoring the embryo to the wall of the embryo sac and facilitating nutrient absorption from the surrounding tissues.
Development Stages of the endosperm of a dicot seed (Arabidopsis)
The development of the endosperm in a dicot seed, such as Arabidopsis, involves several key stages that are distinct from those in monocots. Here are the main stages of endosperm development in Arabidopsis:
- Pre-Fertilization Stage: Similar to monocots, the endosperm cell in Arabidopsis contains two nuclei prior to fertilization. After fertilization, these nuclei fuse with a sperm nucleus.
- Nuclear Division: Following fertilization, the endosperm nucleus undergoes multiple rounds of mitotic divisions without cytokinesis, resulting in a coenocytic (syncytial) structure with many nuclei. In Arabidopsis, this can lead to the formation of an endosperm with over 200 nuclei.
- Cellularization: Cellularization begins after the final mitosis of the endosperm nuclei. This process starts in the micropylar region and progresses as a wave through the peripheral and chalazal endosperm. The formation of cell walls occurs, leading to the development of individual endosperm cells.
- Differentiation: As cellularization progresses, the endosperm differentiates into various cell types. In Arabidopsis, the endosperm is typically ephemeral, meaning it is resorbed as the embryo develops. The endosperm cells may include the basal endosperm transfer layer (BETL), which is involved in nutrient transfer to the embryo.
- Maturation: At maturity, the endosperm in Arabidopsis is largely resorbed, and only a single layer of living cells remains in the mature seed. These cells are crucial for the embryo’s development and nutrient supply during germination.
Structure of Monocot embryo
The structure of a monocot embryo is distinct from that of dicots, characterized by a single large cotyledon and several specialized protective structures. Below is a detailed explanation of the key components of a monocot embryo:
- Scutellum (Single Cotyledon):
- Single Cotyledon: Monocots are distinguished by having only one cotyledon, known as the scutellum. This large cotyledon is located on the lateral side of the embryonal axis.
- Function: The scutellum plays a crucial role in nutrient absorption during germination, helping to transfer stored food from the endosperm to the developing embryo.
- Embryonal Axis:
- Central Framework: The embryonal axis serves as the main structure connecting the various components of the embryo. It is divided into different regions that give rise to the plant’s primary organs.
- Radicle:
- Root System Initiation: The radicle is positioned at the lower end of the embryonal axis and is the precursor to the plant’s root system.
- Protection: The radicle is enclosed in a protective sheath known as the coleorhiza, which safeguards it during germination.
- Root Cap:
- Protection of Radicle Tip: The root cap is a protective structure that covers the tip of the radicle, helping it penetrate the soil as the root grows.
- Epicotyl:
- Above the Cotyledon: The part of the embryonal axis above the attachment point of the scutellum is called the epicotyl.
- Shoot Development: The epicotyl contains the shoot apex and a few leaf primordia, which are the early stages of leaf formation.
- Plumule:
- Lateral Position: The plumule, or the embryonic shoot apex, is laterally positioned in the monocot embryo.
- Coleoptile Protection: The plumule is protected by a hollow, leaf-like structure called the coleoptile, which shields the young shoot during germination and helps it emerge through the soil.
- Coleorhiza:
- Sheath Around Radicle: The coleorhiza is a sheath that surrounds the radicle, providing additional protection as the root begins to grow.
- Epiblast:
- Rudimentary Second Cotyledon: The epiblast is a small structure that represents the remnants of a second cotyledon, though it is not fully developed in monocots.
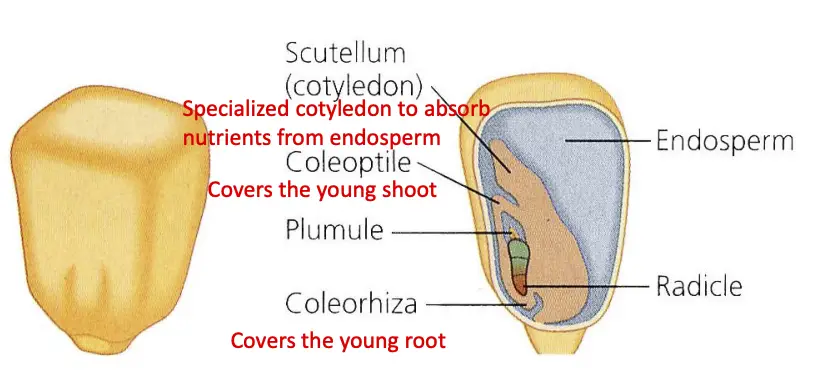
Development Stages of the endosperm of a monocot grain (maize)
The development of the endosperm in a monocot grain, such as maize, occurs through several distinct stages:
- Pre-Fertilization Stage: The endosperm cell prior to fertilization contains two nuclei. After fertilization, these nuclei fuse with a sperm nucleus.
- Nuclear Division: Following fertilization, the endosperm nucleus undergoes multiple rounds of mitotic divisions without cytokinesis, resulting in a coenocytic (free-nuclear) structure with many nuclei. For example, in maize, this can lead to stages with over 200 nuclei.
- Cellularization: After the completion of nuclear divisions, cellularization begins. This process starts at the micropylar end of the endosperm, where cell wall formation initiates and spreads from the periphery inward, leading to the formation of individual cells.
- Maturation: As cellularization progresses, the endosperm develops into a cellular structure that surrounds the embryo. At this stage, the endosperm is primarily composed of starchy cells, which serve as a major storage reserve for the developing seed.
- Final Development: At maturity, the endosperm of maize consists of four major cell types: starchy endosperm cells, aleurone layer cells, transfer cells, and cells in the embryo-surrounding region. The starchy endosperm expands rapidly and is filled with starch granules, while the aleurone layer remains viable and plays a role in seed germination and nutrient mobilization.
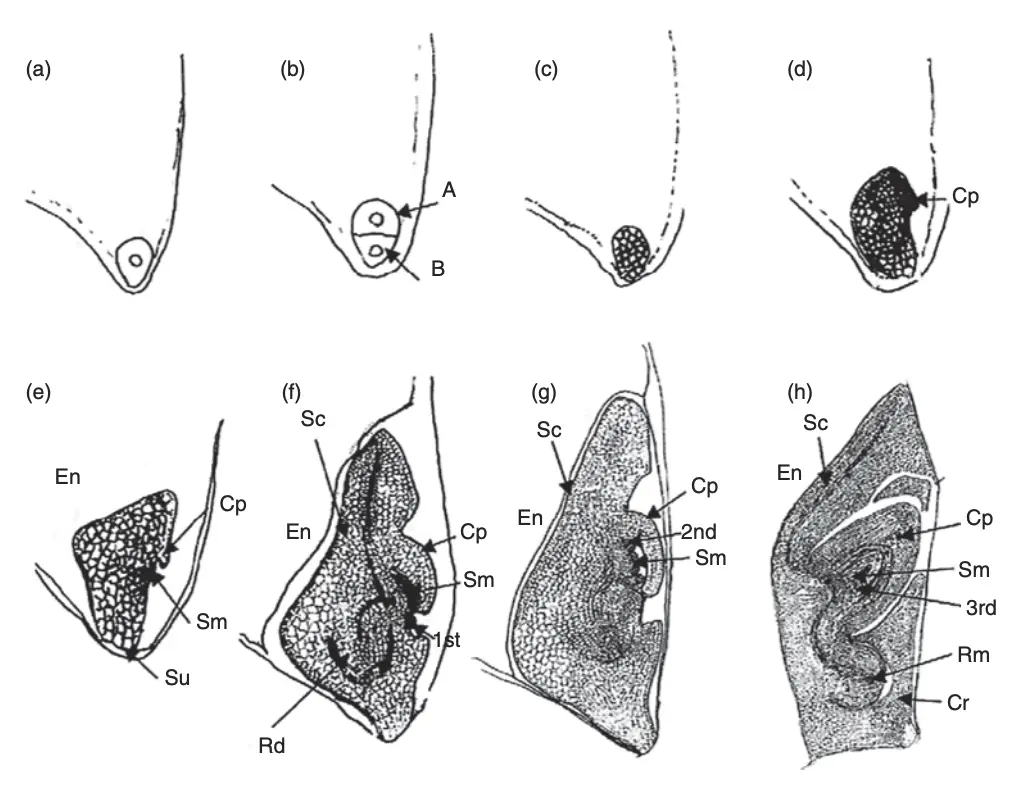
undergoes (b) mitosis to form an axial (A) and a basal (B) cell. (c) A multicellular proembryo is produced from the former, and then (d) an early coleoptile (Cp) stage. Further cell divisions result in (e) a late coleoptile stage when the shoot meristem (Sm) is visible. (f) By the first leaf (1st) stage other tissues are differentiated including the scutellum and the radicle, and by (g) the second leaf (2nd) stage differentiation is more advanced. In the mature embryo (h) all of the tissues are evident, with the addition of a third leaf (3rd), root meristem (Rm) and the structure covering this, the coleorhiza (Cr). En, endosperm; Su, suspensor. (From Black et al., 2006.) | Image Source: DOI: 10.1079/9781780641836.0001
Development Stages of Monocot embryo (Development (Grass type))
The development of the monocot embryo involves a series of carefully orchestrated stages, each contributing to the formation of the various structures necessary for the plant’s early growth. Below is a step-by-step outline of the key stages in monocot embryo development:
- First Zygote Division:
- Asymmetrical and Oblique Division: The initial division of the zygote is oblique and transverse, resulting in the formation of two cells of unequal size: a small apical cell and a larger basal cell.
- Formation of the Four-Cell Proembryo:
- Right-Angle Divisions: Both the apical and basal cells undergo further divisions at right angles to the first division. This leads to the formation of a four-celled structure known as the proembryo.
- Progression to 16-Cell and 32-Cell Stages:
- Successive Divisions: Continued cell division at various planes results in the formation of a 16-celled proembryo, which further divides to form a 32-celled proembryo. It is at this stage that the foundation for the various embryonic structures begins to take shape.
- Organogenesis in the 32-Cell Proembryo:
- Development of Embryo Organs: In the 32-celled proembryo, organogenesis—the process of organ formation—takes place. Specific regions of the proembryo begin to differentiate into distinct structures that will become crucial parts of the mature plant.
- Formation of the Scutellum:
- Cotyledon Development: Cells in the lateral-apical region of the proembryo develop into the single cotyledon, known as the scutellum. This structure is essential for nutrient absorption during the early stages of germination.
- Development of the Plumule and Radicle:
- Shoot and Root Initiation: Below the scutellum, the plumule and radicle begin to form. The plumule will eventually develop into the shoot system, while the radicle will give rise to the root system.
- Formation of Protective Structures:
- Coleoptile and Coleorhiza: The outer layer of cells differentiates into protective structures such as the coleoptile, which encloses the plumule, and the coleorhiza, which surrounds the radicle. Additionally, the root cap develops to protect the radicle as it grows into the soil.
Alternative Forms of Embryogenesis
Alternative forms of embryogenesis, including somatic embryogenesis and androgenesis, offer unique pathways for embryo development beyond the traditional processes observed in plants. These methods provide valuable techniques for plant propagation, genetic manipulation, and research.
Somatic Embryogenesis
Somatic embryogenesis involves the formation of embryos from somatic cells, which are not normally involved in embryo development. This process is distinct from typical plant embryogenesis, as it does not require a seed coat or endosperm.
- Formation of Somatic Embryos:
- Source of Embryos: Somatic embryos originate from plant cells that do not usually form embryos. These cells, often derived from tissue cultures, lack the typical seed structures.
- Callus Formation: Initially, plant tissue cells develop into a callus, which is a mass of undifferentiated cells.
- Role of Plant Growth Regulators: The culture medium used in somatic embryogenesis contains plant growth regulators that facilitate the formation of the callus and its subsequent differentiation into embryos. The types and concentrations of these regulators vary depending on the plant species.
- Development Process:
- Asymmetrical Cell Division: Somatic embryo development typically involves asymmetrical cell division. This division creates structures similar to those in normal embryogenesis.
- Absence of Suspensor Cell: Unlike conventional embryogenesis, somatic embryogenesis may not involve the formation of a specific cell type known as the suspensor cell. However, the embryo still develops successfully despite this difference.
- Applications:
- Cloning and Genetic Transformation: Somatic embryogenesis is utilized for cloning plants, performing genetic transformations, and producing synthetic seeds. It allows for the generation of genetically identical plants from single cells (protoplasts) and facilitates virus removal.
Androgenesis
Androgenesis is a form of embryogenesis where an embryo develops from an immature pollen grain, usually under stressful conditions.
- Formation from Pollen:
- Initial Cell Composition: The process starts with a single pollen grain containing three cells. These cells undergo specific divisions to form an embryo.
- Specific Conditions: Androgenesis occurs under particular conditions that trigger the pollen grain to develop into an embryo.
- Stages of Development:
- Acquiring Embryonic Potential: The initial stage involves the pollen grain acquiring the potential to develop into an embryo.
- Initiating Cell Divisions: Following this, cell divisions commence to form the basic structures of the embryo.
- Pattern Formation: The embryo then undergoes pattern formation, which is similar to the regular stages of embryogenesis.
- Limitations and Timing:
- Vegetative Cell Development: Androgenesis must occur before the pollen grain’s vegetative cells start producing starch and proteins. Once these processes begin, androgenesis is no longer possible.
What are the stages of seed development in angiosperms?
The stages of seed development in angiosperms can be broadly categorized into three main phases:
- Phase I: Histodifferentiation:
- This phase involves the formation of different tissues within the embryo and surrounding structures. It is characterized by extensive cell divisions and the establishment of the basic architecture of the seed. Key processes include:
- Fertilization, where the zygote forms.
- Development of the embryo, which includes the formation of the cotyledons, hypocotyl, and radicle.
- Formation of the endosperm, which provides nutrients to the developing embryo.
- This phase involves the formation of different tissues within the embryo and surrounding structures. It is characterized by extensive cell divisions and the establishment of the basic architecture of the seed. Key processes include:
- Phase II: Cell Enlargement and Reserve Accumulation:
- In this phase, cell enlargement and expansion predominate, with little cell division occurring. The dry weight of the seed increases due to the deposition of storage reserves (such as starch, proteins, and oils) in the endosperm and cotyledons. Key features include:
- Accumulation of nutrients and energy reserves that will support the embryo during germination.
- Reduction in water content as the seed matures.
- In this phase, cell enlargement and expansion predominate, with little cell division occurring. The dry weight of the seed increases due to the deposition of storage reserves (such as starch, proteins, and oils) in the endosperm and cotyledons. Key features include:
- Phase III: Maturation and Desiccation:
- This final phase involves the slowing and eventual cessation of dry matter accumulation as the seed reaches physiological maturity. Key processes include:
- Maturation drying, where the seed loses water content to about 7-15%, becoming desiccation-tolerant (orthodox seeds).
- The seed enters a quiescent state, exhibiting minimal metabolic activity, and may also enter dormancy in some species.
- In some cases, seeds may develop as recalcitrant seeds, which do not undergo maturation drying and are sensitive to desiccation.
- This final phase involves the slowing and eventual cessation of dry matter accumulation as the seed reaches physiological maturity. Key processes include:
Differences Between Monocot and Dicot Embryo Development
- Number of Cotyledons:
- Dicot Embryo: Contains two cotyledons attached to the embryonal axis. These cotyledons often play a crucial role in nutrient storage and initial seedling development.
- Monocot Embryo: Features a single cotyledon, known as the scutellum, attached to the embryonal axis. This cotyledon primarily functions in nutrient absorption.
- Position of Plumule:
- Dicot Embryo: The plumule, which develops into the shoot, is located distally, meaning it is positioned at the far end of the embryonal axis from the cotyledons.
- Monocot Embryo: The plumule is positioned laterally, situated beside the single cotyledon.
- Position of Cotyledons:
- Dicot Embryo: The cotyledons are arranged laterally, flanking the embryonal axis.
- Monocot Embryo: The single cotyledon occupies the terminal position at the tip of the embryonal axis.
- Coleoptile:
- Dicot Embryo: Lacks a coleoptile.
- Monocot Embryo: Features a coleoptile, which is a protective covering surrounding the plumule, aiding in its emergence from the soil.
- Coleorhiza:
- Dicot Embryo: The coleorhiza is absent.
- Monocot Embryo: Contains a coleorhiza, a protective sheath encasing the radicle, which facilitates its growth into the root system.
- Scutellum:
- Dicot Embryo: The scutellum is absent.
- Monocot Embryo: The cotyledon is referred to as the scutellum, serving as the primary site for nutrient storage and absorption.
- Suspensor:
- Dicot Embryo: Features a relatively large suspensor, which anchors the embryo and aids in nutrient transfer from the surrounding tissues.
- Monocot Embryo: The suspensor is comparatively smaller, reflecting different developmental needs and processes.
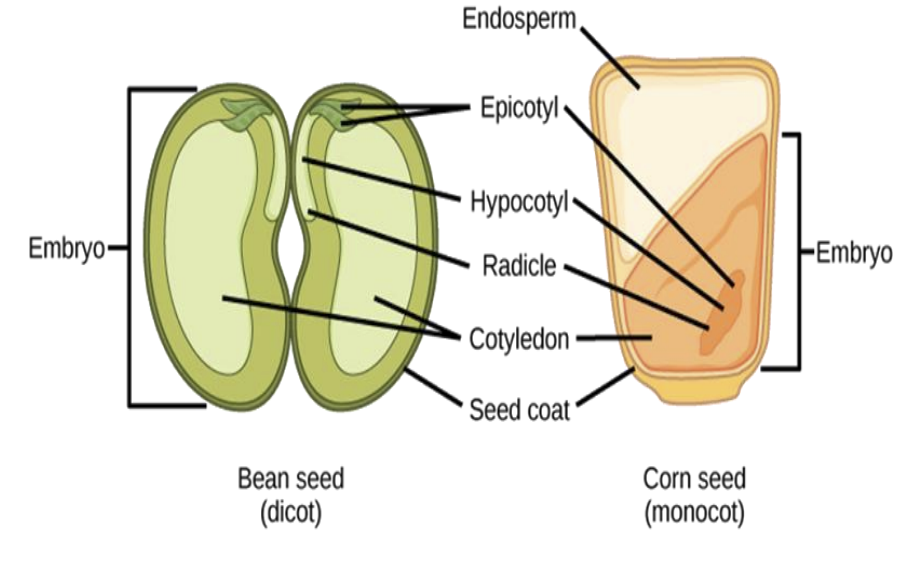
How does seed development differ between monocots and dicots?
Seed development in monocots and dicots exhibits several key differences, reflecting their distinct evolutionary adaptations and anatomical structures. Here are the main differences:
- Number of Cotyledons:
- Monocots: Seeds have a single cotyledon (e.g., grasses, lilies). The cotyledon is often reduced and functions primarily as a scutellum, which aids in nutrient absorption.
- Dicots: Seeds typically have two cotyledons (e.g., beans, sunflowers). The cotyledons are usually more developed and serve as storage organs for nutrients.
- Embryo Structure:
- Monocots: The embryo consists of a single cotyledon, a hypocotyl, and a radicle. The cotyledon is often elongated and forms a protective structure called the coleoptile that covers the first leaves.
- Dicots: The embryo has two cotyledons, a hypocotyl, and a radicle. The cotyledons may be fleshy and store nutrients, which are utilized during germination.
- Endosperm Development:
- Monocots: The endosperm is typically persistent and remains as a significant part of the mature seed, providing nutrients to the developing embryo during germination.
- Dicots: The endosperm is often ephemeral, meaning it is largely consumed by the developing embryo, which relies on the cotyledons for nutrient storage.
- Seed Structure and Anatomy:
- Monocots: The seed structure is generally simpler, with a more uniform distribution of tissues. The endosperm is usually the main storage tissue.
- Dicots: The seed structure is more complex, with distinct layers including the seed coat, endosperm, and cotyledons. The cotyledons often store significant reserves.
- Germination Process:
- Monocots: Germination typically involves the coleoptile emerging first, pushing through the soil to establish the first leaves.
- Dicots: Germination often involves the hypocotyl elongating and pushing the cotyledons above the soil, where they can begin photosynthesis.
- Seed Size and Weight:
- Monocots: Seeds can vary in size but are often smaller and lighter compared to many dicot seeds.
- Dicots: Seeds can be larger and heavier, with some species producing seeds that are significantly larger than those of monocots.
How is nutrient transfer facilitated between the endosperm and the embryo?
Nutrient transfer between the endosperm and the embryo in seeds is a critical process that ensures the developing embryo receives the necessary resources for growth and development. This transfer is facilitated through several mechanisms:
- Transfer Cells:
- In many seeds, specialized cells known as transfer cells develop in the basal endosperm. These cells have increased surface area due to their wall ingrowths, which enhance their ability to transport nutrients. They facilitate the movement of amino acids, sugars, and other nutrients from the endosperm to the embryo through active transport mechanisms.
- Endosperm Structure:
- The endosperm itself is structured to support nutrient transfer. In grasses, for example, the endosperm consists of different cell types, including starchy endosperm cells that store carbohydrates, aleurone layer cells that can produce enzymes, and transfer cells that connect to the vascular tissue of the mother plant. This organization allows for efficient nutrient mobilization and transfer to the embryo.
- Vascular Connections:
- The endosperm is often connected to the embryo through vascular tissues. These connections allow for the direct transport of nutrients and water from the maternal plant to the endosperm and then to the embryo. The vascular system plays a crucial role in maintaining the flow of nutrients during seed development and germination.
- Enzymatic Activity:
- The aleurone layer, which is located just beneath the seed coat and surrounding the endosperm, can produce enzymes that break down stored nutrients in the endosperm. This enzymatic activity helps convert complex carbohydrates and proteins into simpler forms that can be easily absorbed by the embryo.
- Physiological Signals:
- The embryo can also send signals to the endosperm to regulate nutrient transfer. For instance, the embryo may release hormones or other signaling molecules that stimulate the endosperm to mobilize its reserves and facilitate nutrient transfer.
- Cellular Transport Mechanisms:
- Nutrient transfer involves various cellular transport mechanisms, including diffusion, facilitated diffusion, and active transport. These processes ensure that essential nutrients are efficiently moved from the endosperm to the embryo as it develops.
How do genetic factors influence seed development?
Genetic factors play a crucial role in influencing seed development in angiosperms. These factors can affect various aspects of seed formation, including the growth and differentiation of the embryo, endosperm development, and the overall morphology of the seed. Here are some key ways in which genetic factors influence seed development:
- Gene Expression Regulation:
- Specific genes are responsible for regulating the expression of proteins and enzymes that are critical for seed development. For example, genes involved in hormone biosynthesis (such as auxins, gibberellins, and abscisic acid) can influence processes like cell division, elongation, and differentiation during seed development.
- Embryo Development:
- Genetic factors determine the pattern of embryo development, including the formation of cotyledons, hypocotyl, and radicle. Mutations in genes that control these developmental pathways can lead to abnormal embryo morphology or even embryo lethality.
- Endosperm Formation:
- The genetic makeup of both the maternal and paternal contributions can influence endosperm development. For instance, the balance of parental genomes can affect the ploidy level of the endosperm, which in turn influences its growth and nutrient storage capabilities. Imbalances can lead to issues such as endosperm failure or abnormal development.
- Seed Size and Weight:
- Genetic factors can dictate the size and weight of seeds. Certain genes are associated with the regulation of nutrient accumulation in the endosperm and cotyledons, which directly impacts seed size. For example, genes that control starch biosynthesis can influence the amount of starch stored in the endosperm, affecting overall seed weight.
- Dormancy and Germination:
- Genetic factors also play a role in seed dormancy and germination traits. Genes involved in the synthesis of abscisic acid (ABA) can promote seed dormancy, while those involved in gibberellin (GA) synthesis can promote germination. The balance of these hormones, regulated by genetic factors, determines the timing of germination.
- Response to Environmental Cues:
- Genetic factors influence how seeds respond to environmental signals such as light, temperature, and moisture. For example, certain genes may regulate the sensitivity of seeds to light, which can trigger germination in some species. This adaptability is crucial for survival in varying environmental conditions.
- Genetic Interactions:
- Seed development is also influenced by interactions between multiple genes (epistasis) and the genetic background of the plant. The combined effects of various genes can lead to phenotypic variations in seed traits, such as color, shape, and nutrient content.
- Transcription Factors:
- Transcription factors are proteins that regulate the expression of other genes during seed development. Specific transcription factors are known to control key developmental processes, including the transition from embryonic to seedling stages.
What environmental conditions affect seed germination and development?
Seed germination and development are significantly influenced by various environmental conditions. These factors can either promote or inhibit the germination process and subsequent seedling growth. Key environmental conditions affecting seed germination and development include:
- Moisture Availability:
- Water is essential for seed germination. Seeds absorb water through a process called imbibition, which activates metabolic processes necessary for germination. Insufficient moisture can lead to seed dormancy or failure to germinate, while excessive moisture can cause seed rot or fungal infections.
- Temperature:
- Temperature plays a critical role in seed germination. Each species has an optimal temperature range for germination. High temperatures can accelerate germination but may also lead to heat stress, while low temperatures can slow down or inhibit germination. Some seeds require specific temperature fluctuations (e.g., stratification) to break dormancy.
- Light:
- Light can influence germination in some species. Certain seeds require light to germinate (photoblastic seeds), while others may germinate in darkness. The presence of light can trigger specific hormonal responses that promote germination, particularly in seeds with light-sensitive photoreceptors.
- Oxygen Availability:
- Oxygen is necessary for aerobic respiration during germination. Seeds in waterlogged or anaerobic conditions may experience reduced germination rates due to insufficient oxygen, leading to poor seedling establishment.
- Soil Conditions:
- Soil type, structure, and nutrient content can affect seed germination and seedling growth. Well-aerated, well-drained soils promote better germination, while compacted or poor-quality soils can hinder root development and nutrient uptake.
- pH Levels:
- Soil pH can influence nutrient availability and microbial activity in the soil. Most seeds prefer a neutral to slightly acidic pH for optimal germination. Extreme pH levels can inhibit germination and affect seedling health.
- Salinity:
- High salinity levels in the soil can create osmotic stress, making it difficult for seeds to absorb water. Salinity can also lead to ion toxicity, which can inhibit germination and negatively affect seedling growth.
- Nutrient Availability:
- The availability of essential nutrients (such as nitrogen, phosphorus, and potassium) in the soil can impact seedling development. Nutrient deficiencies can lead to poor growth and increased susceptibility to diseases.
- Environmental Stressors:
- Environmental stressors such as drought, flooding, extreme temperatures, and competition from other plants can affect seed germination and seedling survival. Seeds may have evolved mechanisms to cope with these stressors, such as dormancy or delayed germination.
- Microbial Activity:
- Soil microorganisms can influence seed germination and development. Beneficial microbes can enhance nutrient availability and promote healthy root development, while pathogenic microbes can lead to seed rot and disease.
- Sliwinska, Elwira & Bewley, J.. (2014). Overview of seed development, anatomy and morphology. 10.1079/9781780641836.0001.
- https://www.slideshare.net/slideshow/embryogenesis/8551333
- https://sbmrajgurunagar.ac.in/uploads/department/Sy5.pdf
- https://www.slideshare.net/slideshow/embryo-development-241686754/241686754
- https://www.pw.live/exams/neet/embryo/
- https://passel2.unl.edu/view/lesson/9191f241bb92/1
- https://propg.ifas.ufl.edu/04-seeds/01-development/04-seedsdevelopment-embryogenesis.html
- https://www.srnbdc.org/admin/uploads/110922042020173140-MANA_TV_Embryology.pdf
- https://littleflowercollege.edu.in/upload/e_contents/files/a762afbdfaea19233b45b8d53d558b12.pdf
- http://courseware.cutm.ac.in/wp-content/uploads/2021/02/Embryogenesis.pdf