What is Axon?
- An axon, also known as a nerve fiber, is a slender projection that extends from a neuron, or nerve cell, in vertebrates. It plays a crucial role in the nervous system by conducting electrical impulses, called action potentials, away from the neuron’s cell body. The primary function of an axon is to transmit information to other neurons, as well as to muscles and glands. This process enables communication between different parts of the body and is essential for the nervous system’s operation.
- Axons vary in structure depending on the type of neuron. They are one of the two major types of protrusions from a neuron’s cell body, the other being dendrites. While dendrites receive signals from other neurons, axons transmit them. Structurally, axons maintain a consistent diameter throughout their length, unlike dendrites, which typically taper off. Axons can also be much longer than dendrites, allowing them to connect neurons over considerable distances. Some neurons, particularly in invertebrates, have axons that branch and function independently, allowing complex signal transmission.
- Axons are covered by a membrane known as the axolemma, and their internal fluid is referred to as axoplasm. Most axons branch at their ends, forming multiple connections with other neurons. The ends of these branches are called telodendria, which terminate at synaptic connections, enabling communication between neurons. These synapses may occur at the end of an axon or along its length, forming “en passant boutons,” which facilitate signal transmission at multiple points.
- Axons are grouped based on their myelination and function. Group A and B nerve fibers are myelinated, meaning they are covered by a fatty sheath that increases the speed of impulse transmission. Group C nerve fibers are unmyelinated and transmit signals more slowly. Sensory fibers are further classified into types I through IV, depending on their structure and function. Dysfunction in axons can lead to various neurological disorders, affecting both the central and peripheral nervous systems.
- The structure and function of axons are critical to their role in the brain and nervous system. For instance, in the human brain, the corpus callosum, composed of about 200 million axons, facilitates communication between the two hemispheres. Axons are also vital in sensory neurons, such as those responsible for touch and warmth, transmitting impulses from the periphery of the body to the spinal cord.
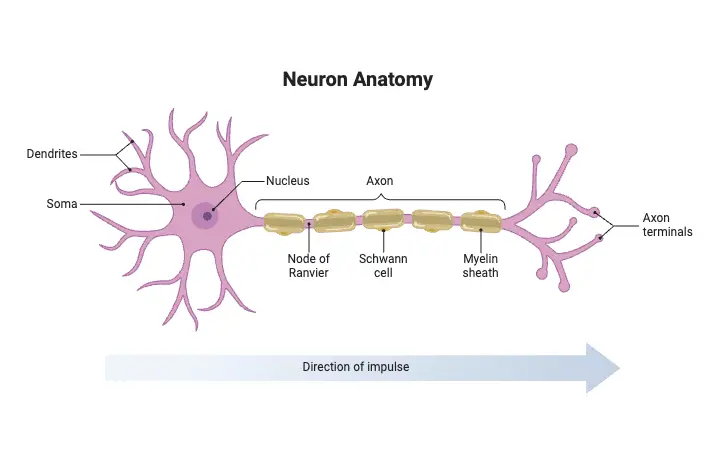
Definition of Axon
An axon is a long, slender projection of a nerve cell (neuron) that transmits electrical impulses, known as action potentials, away from the neuron’s cell body to other neurons, muscles, or glands, facilitating communication within the nervous system.
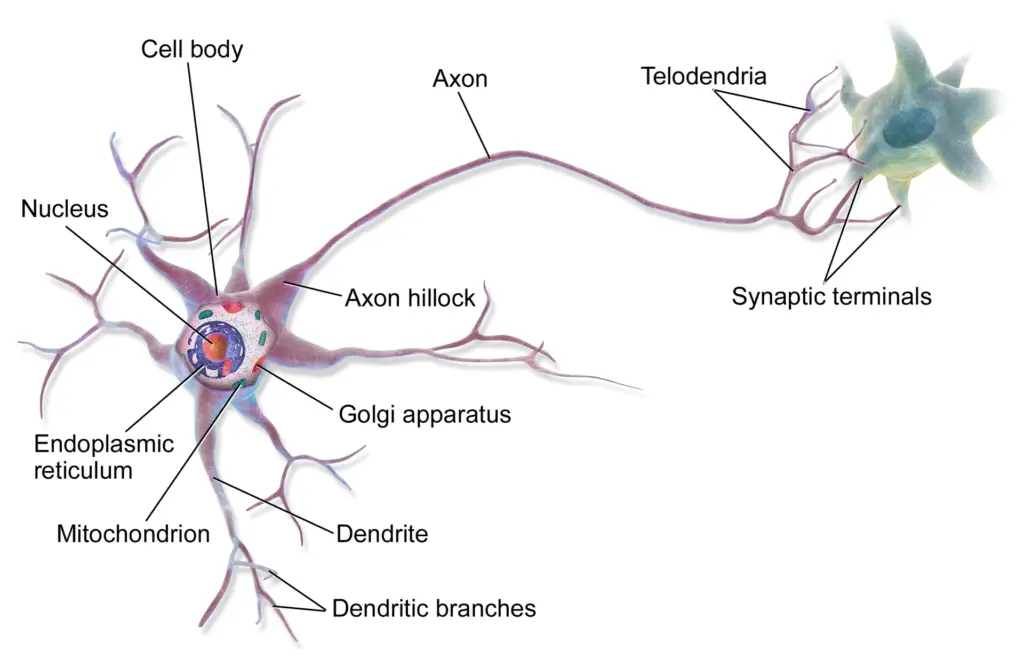
Characteristics features of Axon
Axons are vital structures in the nervous system, responsible for transmitting signals from neurons to other cells, such as muscles and glands. These long, slender projections have distinct characteristics that enable them to efficiently conduct electrical impulses, known as action potentials. Below are the key features of axons:
- Projections from Neuron Cell Bodies
- Axons are one of two types of cytoplasmic protrusions from the neuron cell body, the other being dendrites.
- Transmit Signals
- Axons conduct electrical impulses away from the neuron’s cell body, transmitting information to other neurons, muscles, or glands.
- Shape and Size
- Axons maintain a constant diameter, unlike dendrites, which taper.
- Their length varies, with some axons being just a few millimeters long, while others, such as those in the sciatic nerve, can extend over a meter.
- Branching
- Though axons are less branched than dendrites, they may give off large collateral branches.
- Near their ends, axons split into terminal branches called telodendria.
- Synaptic Knobs
- At the tip of each terminal branch is a synaptic knob, also called an axon terminal or end bulb, where communication with other cells occurs at the synapse.
- Synaptic Vesicles
- Synaptic vesicles are present within synaptic knobs and contain neurotransmitters, which are released when an action potential reaches the terminal.
- Axon Hillock
- The axon hillock is a cone-shaped region where the axon attaches to the neuron’s cell body. It plays a key role in initiating action potentials.
- Axon Initial Segment
- Located immediately after the axon hillock, this region is structurally distinct and helps generate action potentials. It precedes the myelin sheath.
- Trigger Zone
- The axon’s initial segment serves as the trigger zone for action potential generation due to its high concentration of voltage-gated ion channels.
- Axolemma
- The axon is covered by a membrane called the axolemma, which houses proteins necessary for conducting action potentials.
- Axoplasm
- The axoplasm is the cytoplasm within the axon. It contains neurofilaments that help transport materials along the axon.
- Myelination
- Some axons are covered by a myelin sheath, which is formed by Schwann cells in the peripheral nervous system and oligodendrocytes in the central nervous system.
- The myelin sheath facilitates faster signal transmission through saltatory conduction.
- Nodes of Ranvier
- These are gaps in the myelin sheath where action potentials are regenerated in myelinated axons, aiding in rapid signal propagation.
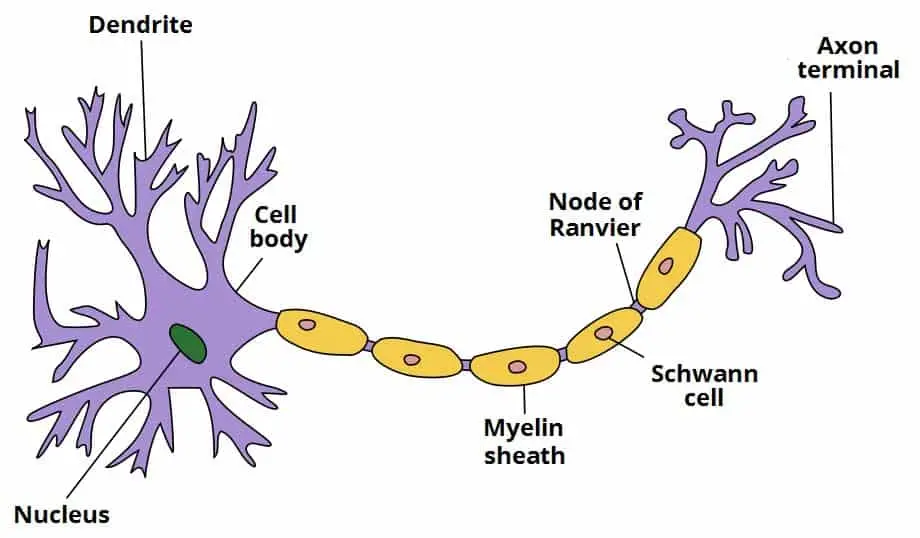
Axon Structure (Components of Axon)
The axonal region contains several specialized structures that work together to transmit electrochemical signals, known as action potentials, efficiently across long distances. Each component plays a specific role in ensuring accurate signal conduction and communication between neurons or other target cells.
- Axon Hillock
- Located at the junction between the neuron’s soma (cell body) and the axon, the axon hillock plays a crucial role in initiating action potentials. It integrates incoming signals from the dendrites, and if the summed signals reach a threshold, it generates an action potential that travels down the axon.
- Axon Initial Segment (AIS)
- Positioned immediately after the axon hillock, the AIS is rich in voltage-gated sodium channels, which are essential for starting action potentials. The AIS also maintains neuronal polarity, ensuring that signals move in one direction—dendrites receive signals, and the axon transmits them away from the cell body.
- Axon Proper
- This is the main, elongated portion of the axon, responsible for conducting action potentials over long distances. Its uniform structure is designed to promote efficient signal transmission.
- Axolemma
- The axolemma is the membrane that surrounds the axon. It contains ion channels and pumps critical for maintaining the electrochemical gradients necessary for action potential propagation. These proteins control the flow of ions in and out of the axon, which is key to signal conduction.
- Axoplasm
- The axoplasm is the cytoplasm inside the axon. It contains structural elements like neurofilaments and microtubules, which support the axon and facilitate intracellular transport of materials, such as proteins and organelles, between the soma and the axon terminal.
- Myelin Sheath (in Myelinated Axons)
- Many axons are wrapped in a fatty layer called the myelin sheath, which insulates the axon and significantly increases the speed of action potential conduction. In the peripheral nervous system, Schwann cells produce the myelin sheath, while in the central nervous system, it is formed by oligodendrocytes.
- Nodes of Ranvier (in Myelinated Axons)
- These are small, regularly spaced gaps in the myelin sheath. They contain clusters of voltage-gated ion channels that allow for saltatory conduction, a process where action potentials jump from node to node, greatly increasing the speed of signal transmission.
- Telodendria
- As the axon nears its target, it divides into smaller branches called telodendria. This branching allows one axon to communicate with multiple target cells at once.
- Axon Terminals (Synaptic Knobs)
- Located at the ends of telodendria, axon terminals form synapses with target cells. These specialized structures enable the transfer of signals from the neuron to another neuron, muscle, or gland.
- Synaptic Vesicles (within Axon Terminals)
- Synaptic vesicles within the axon terminals contain neurotransmitters. When an action potential reaches the terminal, it triggers the release of these neurotransmitters into the synapse. The neurotransmitters bind to receptors on the target cell, allowing the signal to be transmitted across the synapse.
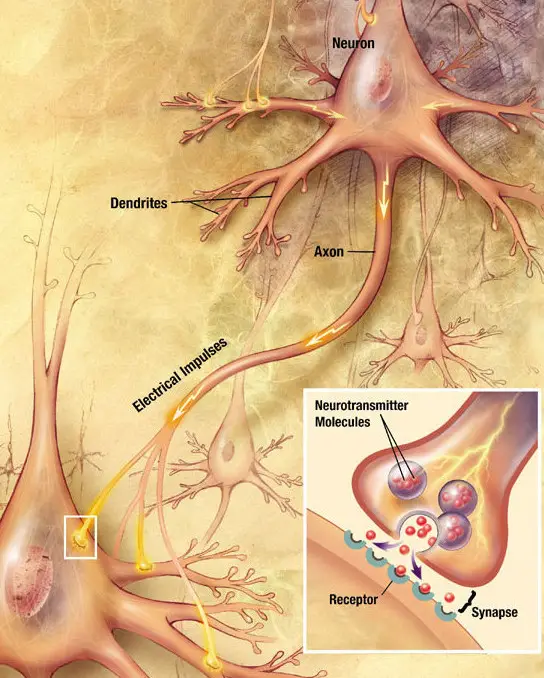
Types of Axon
Axons, as essential components of neurons, can be classified based on their function, structure, and signal conduction properties. Each type of axon is adapted to perform specific roles in the nervous system. Understanding these types is crucial for comprehending how information is processed and transmitted in the body. Here is a detailed breakdown of the various types of axons:
- Classification by Function and Signal Conduction
Axons can be categorized according to the type of signals they carry and their role in the nervous system:- Motor Axons
- Motor axons originate from lower motor neurons.
- They transmit signals from the central nervous system to muscles, causing contraction and movement.
- Sensory Axons
- Sensory axons relay sensory information from the body to the central nervous system.
- Different types of sensory fibers, with varying diameters and conduction speeds, innervate sensory receptors.
- Autonomic Axons
- These axons are part of the autonomic nervous system, which regulates involuntary functions such as heart rate, digestion, and respiration.
- Autonomic axons are divided into preganglionic and postganglionic fibers, depending on their location and function.
- Motor Axons
- Classification by Myelination
Axons can also be classified based on whether they are myelinated or unmyelinated:- Myelinated Axons
- Myelinated axons are wrapped in a fatty insulating layer called the myelin sheath.
- This sheath speeds up signal transmission and is produced by Schwann cells in the peripheral nervous system and oligodendrocytes in the central nervous system.
- Unmyelinated Axons
- These axons lack a myelin sheath, leading to slower signal transmission compared to myelinated axons.
- They are present in both the central and peripheral nervous systems.
- Myelinated Axons
- Classification by Conduction Velocity and Diameter
Erlanger and Gasser developed a classification system based on an axon’s conduction speed, diameter, and myelination. This system organizes axons into groups:- Group A
- These are large-diameter, myelinated axons with high conduction velocities.
- They are further subdivided into Aα, Aβ, Aγ, and Aδ, each serving different functions related to motor control and sensory processing.
- Group B
- Group B axons are myelinated but have smaller diameters and slower conduction velocities than group A fibers.
- These fibers are typically involved in autonomic functions.
- Group C
- These are unmyelinated axons with small diameters and slow conduction velocities.
- They primarily carry pain and temperature sensations.
- Group A
Development and growth of Axon
The development and growth of an axon are critical processes in the formation of functional neural circuits. Axons extend from neurons and play a key role in establishing communication with target cells, such as other neurons, muscles, or glands. The following provides a detailed breakdown of the stages and mechanisms involved in axon development and growth:
- Development
- Initial Stage: During early neuronal development, cells produce several neurites that are indistinguishable at first. Eventually, one of these neurites is selected to become the axon.
- Axon Specification: It is now understood that axon specification occurs after the elongation of the neurite. The factors guiding this specification process are still being investigated.
- Factors Influencing Axon Development:
- Extracellular Signaling:
- Netrin: A secreted protein essential for axon formation; mutations in its receptor (UNC-5) can prevent proper axon formation.
- Neurotrophic Factors: Molecules like NGF (nerve growth factor), BDNF (brain-derived neurotrophic factor), and NT-3 (neurotrophin-3) are crucial for axonal growth and act through Trk receptors.
- Plasma Membrane Ganglioside Sialidase (PMGS): This enzyme facilitates axon elongation by activating TrkA receptors at the neurite tip.
- Intracellular Signaling: Increased PI3K activity at the tip of the future axon is necessary for its development.
- Cytoskeletal Dynamics: Neurites contain varying levels of actin filaments. The neurite with the least amount of actin typically becomes the axon.
- Extracellular Signaling:
- Growth
- Growth Cone: The growth cone, located at the tip of the growing axon, plays a pivotal role in axonal navigation and environment sensing.
- Lamellipodia and Filopodia:
- Filopodia: Finger-like extensions that adhere to surfaces and probe the environment.
- Lamellipodia: Sheet-like regions that lie between the filopodia, densely packed with actin.
- Guidance Cues: The growth cone is influenced by a variety of guidance cues, which may attract or repel it. These cues include:
- Netrin, ephrins, and semaphorins: Molecules that interact with receptors on the growth cone and guide its path.
- Guidepost Cells: These immature neurons release guidance signals that direct axonal growth either by attraction or repulsion.
- Cell Adhesion Molecules (CAMs): CAMs provide a surface for the axon to grow along, including:
- Neural CAMs: Proteins like N-CAM, TAG-1, and MAG facilitate axon guidance.
- Extracellular Matrix Molecules: Laminin, fibronectin, tenascin, and perlecan assist in axon pathfinding.
- Axon Diameter Increase: After the axon reaches its target, its diameter increases significantly, sometimes up to five times, enhancing the speed of signal transmission.
- Neuroregeneration: In the event of damage, axons have the potential to regenerate and form new synaptic connections, provided the neuron’s cell body remains intact. However, certain factors in the CNS, such as Nogo-A, can inhibit regeneration.
- Length Regulation
- Axon Length Variation: Axons can vary greatly in length, from micrometers to over a meter, depending on the type and function of the neuron.
- Cellular Mechanisms: Specific mechanisms ensure that axons grow to appropriate lengths and form correct connections.
- Motor Proteins: Motor proteins regulate axon length by transporting signaling molecules between the soma and the growth cone. The frequency of these transports is linked to the axon’s length.
How does myelination affect the speed of action potential conduction?
Myelination plays a crucial role in enhancing the speed of action potential conduction along axons. Understanding how myelin affects this process provides insight into the efficiency of neural communication.
- Insulation
- Myelin is a fatty substance produced by Schwann cells in the peripheral nervous system (PNS) and oligodendrocytes in the central nervous system (CNS). This myelin sheath wraps around axons in segmented layers, functioning as an electrical insulator. By preventing the leakage of electrical current across the axonal membrane, myelination facilitates faster and more efficient action potential propagation. This can be likened to the insulation around an electrical wire, which minimizes signal loss.
- Saltatory Conduction
- Myelination enables a specialized conduction mechanism known as saltatory conduction. In myelinated axons, action potentials do not propagate continuously along the membrane. Instead, they “jump” between the Nodes of Ranvier, which are the small gaps in the myelin sheath rich in voltage-gated ion channels. This jumping mechanism significantly accelerates signal transmission, as action potentials are quickly generated at each node, allowing for rapid depolarization and repolarization.
- Increased Efficiency
- By confining the areas of ion exchange to the Nodes of Ranvier, myelination reduces the overall capacitance of the membrane. This allows for quicker changes in membrane potential, further enhancing the speed of action potential conduction. The combined insulating effect of myelin and the concentrated activity at the nodes leads to a highly efficient transmission of electrical signals along the axon.
- Clinical Significance
- The critical nature of myelination is underscored in conditions such as multiple sclerosis (MS), where the immune system targets and damages the myelin sheath. This demyelination disrupts saltatory conduction, resulting in a significant slowing or complete blockage of nerve impulse transmission. The consequences can manifest as various neurological symptoms, including muscle weakness, visual disturbances, and coordination issues, highlighting the essential role of myelin in maintaining normal neurological function.
How do axons contribute to the transmission of information within the nervous system?
Axons play a vital role in the transmission of information within the nervous system, serving as the primary conduits for electrical impulses. Their structure and function are intricately designed to facilitate rapid and effective communication between neurons and other target cells.
- Action Potential Generation and Transmission
- Axons generate and transmit electrical impulses known as action potentials. This process begins at the axon hillock, which functions as a critical trigger zone. It integrates incoming signals from dendrites and the soma, and if these signals surpass a specific threshold, an action potential is initiated. This all-or-nothing response is essential for reliable communication between neurons.
- Synaptic Transmission
- Upon reaching the axon terminal, the action potential induces the release of neurotransmitters at synapses. These neurotransmitters act as chemical messengers, binding to receptors on adjacent neurons or target cells and effectively transmitting the signal across the synaptic gap. The axon terminal, or synaptic bouton, contains synaptic vesicles that store these neurotransmitters, ensuring that they are readily available for release during synaptic transmission.
- Myelination and Saltatory Conduction
- Many axons are wrapped in a fatty insulating layer known as myelin, produced by Schwann cells in the peripheral nervous system and oligodendrocytes in the central nervous system. This myelin sheath acts as an electrical insulator, significantly increasing the speed of action potential conduction through a mechanism called saltatory conduction. In this process, action potentials “jump” between the Nodes of Ranvier, which are gaps in the myelin sheath containing a high density of voltage-gated ion channels. This arrangement allows for rapid and efficient transmission of information across long distances.
- Axonal Transport
- Axons depend on axonal transport to maintain their functionality. Lacking ribosomes, axons cannot synthesize proteins independently. Therefore, proteins and other essential materials are transported from the neuron’s cell body to the axon terminal via anterograde transport. Conversely, waste materials are sent back to the cell body for degradation through retrograde transport. This continual movement of materials is crucial for preserving the structural integrity of the axon, facilitating neurotransmitter delivery, and supporting overall neuronal health.
Factors contribute to the speed of action potential conduction
The conduction velocity of action potentials, or the speed at which electrical impulses travel along an axon, is influenced by several critical factors. Understanding these factors is essential for grasping how the nervous system functions effectively.
- Myelination
- The presence of a myelin sheath is a key factor determining conduction velocity. Myelinated axons transmit action potentials significantly faster than unmyelinated ones due to a mechanism known as saltatory conduction. In myelinated axons, the action potential “jumps” from one Node of Ranvier to the next, minimizing the area where ion exchange occurs. This process is facilitated by the insulating properties of myelin and the high concentration of voltage-gated ion channels at the nodes, which leads to efficient signal transmission. Demyelination, as seen in diseases like multiple sclerosis, can severely impair this rapid conduction and result in various neurological symptoms.
- Axon Diameter
- The diameter of the axon plays a substantial role in conduction speed. Larger diameter axons exhibit lower resistance to current flow, allowing action potentials to propagate more easily. This relationship can be analogized to a wider pipe facilitating a smoother flow of water compared to a narrower one. Thus, axons with larger diameters are able to conduct impulses faster.
- Temperature
- The speed of nerve conduction is also influenced by temperature, typically increasing within physiological limits. Elevated temperatures enhance the kinetic energy of ions, resulting in quicker opening and closing of ion channels, which are vital for generating and propagating action potentials. However, extreme temperatures can adversely affect protein structures essential for conduction, potentially disrupting the process.
- Ion Channel Properties
- The characteristics of ion channels, particularly voltage-gated sodium and potassium channels, significantly affect conduction speed. Channels with faster kinetics—meaning they open and close more rapidly—contribute to quicker action potential propagation. This efficiency is crucial for maintaining the speed of electrical signals.
- Axon Length
- Although not a direct determinant of conduction speed, the length of the axon can introduce slight delays in signal transmission over long distances. As the action potential travels the entire length of the axon, time is required for this propagation. However, in healthy neurons, this delay is typically negligible compared to the other factors influencing conduction speed.
Functions of Axon
Below is a detailed breakdown of the functions of the axon:
- Action Potential Conduction
- The primary function of the axon is to conduct action potentials. These electrical impulses travel rapidly along the axon’s length, starting at the axon hillock.
- The axolemma, the membrane surrounding the axon, contains specialized ion channels that allow for the generation and propagation of action potentials.
- Synaptic Transmission
- Once an action potential reaches the axon terminal, it triggers the release of neurotransmitters.
- Synaptic vesicles in the axon terminal fuse with the axonal membrane, releasing neurotransmitters into the synapse, the gap between neurons.
- These neurotransmitters then bind to receptors on the target cell, influencing its activity through either excitation or inhibition.
- Signal Amplification
- Axons are capable of amplifying action potentials as they travel long distances.
- Voltage-gated sodium channels play a role in this amplification, ensuring that the electrical signal maintains its strength over large distances in the body.
- Axonal Transport
- Axons rely on a transport system to move materials to and from the cell body.
- Anterograde transport moves nutrients and organelles from the soma to the axon terminal.
- Retrograde transport carries waste products or signaling molecules back to the soma for processing or degradation.
- Maintaining Neuron Health
- By regulating the flow of proteins, lipids, and neurotransmitters through axonal transport, the axon plays a crucial role in maintaining the overall health of the neuron and ensuring efficient cellular communication.
- Clinical Significance of Axon Function
- Demyelination: Diseases like multiple sclerosis damage the myelin sheath, impairing signal transmission and causing neurological deficits.
- Axonal Injury: Physical trauma to axons can disrupt communication between neurons, leading to sensory or motor impairments.
- Neurodegenerative Diseases: Progressive axonal degeneration, as seen in conditions like Parkinson’s and Alzheimer’s, results in the loss of cognitive and motor functions.
What are the key differences between an axon and a dendrite?
Both axons and dendrites are essential components of neurons, serving different roles in the transmission and reception of signals. Understanding their differences in structure and function provides insight into how neurons communicate within the nervous system.
- Shape and Size
- Dendrites are typically shorter and tapering, forming a complex, branching network around the neuron’s soma. In contrast, axons are elongated structures that maintain a consistent diameter and can extend over considerable distances, sometimes more than a meter.
- Structure
- Rough Endoplasmic Reticulum and Ribosomes: Dendrites contain rough endoplasmic reticulum and ribosomes, allowing for local protein synthesis. Axons, however, lack these structures, relying on the cell body for the production of proteins necessary for their function.
- Cytoskeleton: Both axons and dendrites possess cytoskeletal elements, but their compositions differ. Dendrites contain microtubule-associated protein 2 (MAP2), while axons do not, reflecting their distinct functional requirements.
- Membrane Composition: The membranes of dendrites and axons vary significantly in ion channel types. Axons predominantly feature voltage-gated ion channels, crucial for action potential generation and propagation. In contrast, dendrites mainly contain ligand-gated ion channels, which respond to neurotransmitters, facilitating signal reception.
- Function
- Signal Reception and Transmission: Dendrites serve as the primary input regions for neurons, receiving signals from numerous presynaptic neurons. They process and integrate these signals within the soma. Conversely, axons are responsible for transmitting action potentials, which are rapid electrical impulses that travel away from the neuron to communicate with other cells.
- Action Potential Generation and Conduction: Axons are specialized for generating and conducting action potentials. Their structural adaptations, such as myelin sheaths (in myelinated axons) and nodes of Ranvier, enhance the speed and efficiency of signal transmission.
- Number and Structure
- Neurons typically have a single axon that may branch into multiple telodendria, each ending in axon terminals. This singular axonal structure ensures focused transmission of output to target cells. In contrast, neurons can possess numerous dendrites, significantly increasing their receptive surface area and enabling the reception of input from multiple sources.
- Direction of Information Flow
- Dendrites receive incoming signals and direct them toward the soma, indicating an inward flow of information. In contrast, axons convey signals away from the soma, demonstrating an outward flow of information. This unidirectional communication is vital for the orderly functioning of neural circuits.
Facts
- Did you know that axons are responsible for transmitting electrical impulses away from the neuron’s cell body?
- Did you know that the axon hillock is often referred to as the “trigger zone” for generating action potentials?
- Did you know that myelinated axons can conduct action potentials up to 100 times faster than unmyelinated ones?
- Did you know that saltatory conduction allows action potentials to “jump” between Nodes of Ranvier, enhancing speed?
- Did you know that Schwann cells in the peripheral nervous system and oligodendrocytes in the central nervous system create the myelin sheath around axons?
- Did you know that the presence of Nodes of Ranvier facilitates rapid depolarization at specific points along the axon?
- Did you know that axons primarily contain voltage-gated ion channels that are essential for action potential propagation?
- Did you know that axonal transport is crucial for delivering proteins and materials from the neuron’s cell body to the axon terminal?
- Did you know that synaptic terminals at the end of axons release neurotransmitters to communicate with other neurons or target cells?
- Did you know that larger diameter axons have lower resistance, allowing for faster conduction of electrical signals?
- Did you know that diseases like multiple sclerosis can impair action potential transmission by damaging the myelin sheath?
- Did you know that the conduction velocity of action potentials increases with temperature due to heightened ion activity?
- Did you know that the axoplasm contains neurofilaments that support the structure of the axon and facilitate transport?
- Did you know that an axon typically branches into multiple telodendria, enabling communication with several target cells?
- Did you know that the length of an axon can affect the timing of signal transmission, particularly over long distances?
Take a Quiz
- Muzio MR, Fakoya AO, Cascella M. Histology, Axon. [Updated 2022 Nov 14]. In: StatPearls [Internet]. Treasure Island (FL): StatPearls Publishing; 2024 Jan-. Available from: https://www.ncbi.nlm.nih.gov/books/NBK554388/
- Waxman, Stephen G., Jeffery D. Kocsis, and Peter K. Stys (eds), The Axon: Structure, Function and Pathophysiology (New York
, 1995; online edn, Oxford Academic, 1 May 2009), https://doi.org/10.1093/acprof:oso/9780195082937.001.0001, accessed 4 Sept. 2024. - https://www.getbodysmart.com/nerve-cells/axon/
- https://teachmeanatomy.info/the-basics/ultrastructure/nerves/
- https://qbi.uq.edu.au/brain/brain-anatomy/axons-cable-transmission-neurons
- https://www.verywellhealth.com/what-is-an-axon-5190652
- https://www.physio-pedia.com/Axons