What is Agrobacterium-Mediated Gene Transfer?
- Agrobacterium-mediated gene transfer is a significant biotechnological technique employed in the field of plant transformation. This method utilizes the natural capabilities of the bacterium Agrobacterium tumefaciens, a well-known phytopathogen responsible for crown gall disease in plants. The bacterium infects host plants through wound sites, enabling the transfer of genetic material, specifically the transferred (T)-DNA, into the plant cells.
- Agrobacterium tumefaciens employs a specialized bacterial type IV secretion system to facilitate the transfer of T-DNA. This process begins when the bacterium comes into contact with a wound site on a plant. The T-DNA, which resides on a tumor-inducing plasmid (Ti plasmid), is transferred into the plant’s genome, leading to the formation of tumors that manifest as crown gall disease. The ability of Agrobacterium to integrate foreign DNA into the host plant genome makes it an invaluable tool in agricultural biotechnology.
- The genus Agrobacterium comprises various species, each associated with different disease symptomology and host range. Notable species include A. radiobacter, A. vitis, A. rhizogenes, A. rubi, and A. tumefaciens. The selection of a specific species for transformation depends on the target plant species and the desired traits to be expressed in the transformed plant. The host range of these bacteria is influenced by a combination of bacterial factors, such as virulence genes and T-DNA oncogenes, as well as plant factors, which include the necessary genes for transformation and tumor formation.
- The natural diversity observed within the Agrobacterium genus is largely dictated by the presence of the Ti or Ri plasmid, which carries the genetic information essential for the pathogenic process. Different strains of Agrobacterium can be isolated from various environments and host plants globally. Common host plants for transformation include roses, poplar, weeping fig, chrysanthemums, and numerous fruit trees.
- Morphologically, Agrobacterium is a Gram-negative, rod-shaped bacterium measuring approximately 1.5 to 3 µm in length and 0.6 to 1.0 µm in width. The bacterium is motile, equipped with one to six flagella. It is strictly aerobic and does not form spores, thriving in soil environments. The capabilities of Agrobacterium extend beyond its pathogenic nature, offering significant clinical and biotechnological applications, particularly in the development of genetically modified plants.
Agrobacterium-mediated Gene Transfer Principle
Agrobacterium-mediated gene transfer is a sophisticated process that enables the genetic modification of plants through the natural infection mechanisms of the bacterium Agrobacterium tumefaciens. The underlying principle of this transformation hinges on the transfer of a segment of DNA, referred to as T-DNA, from the bacterium into the plant cells, where it can integrate into the host genome. This technique has become a cornerstone of plant biotechnology, allowing for precise genetic alterations in various plant species.
- T-DNA and Plasmid Structure: The T-DNA is a specific segment of the Ti (tumor-inducing) plasmid that consists of 25 base pair (bp) repeats flanking the T-region. The Ti plasmid is essential for the transformation process as it carries the genes responsible for tumor formation and opine synthesis. Within the Ti plasmid, there are additional functional regions, including the virulence (vir) region composed of seven major loci, which facilitate the transfer and integration of T-DNA into the plant genome.
- Mechanism of Transformation: The transformation process initiates when Agrobacterium enters the plant through wound sites. Upon infection, the bacteria bind to the plant cells, a process significantly enhanced by phenolic compounds, particularly acetosyringone (AS), released from the injured plant tissues. The presence of AS activates VirA proteins in Agrobacterium, leading to the phosphorylation of VirG, which subsequently binds to and induces the expression of other virulence genes.
- T-strand Formation and Transfer: Once activated, VirD proteins play a crucial role in the generation of the T-strand, which is a single-stranded copy of the T-DNA. The VirD2 protein covalently binds to the 5′ end of the T-strand, marking it for transfer. Other virulence factors, including VirE2 and VirB proteins, associate with the T-strand, forming a protective T-complex that facilitates its transport into the plant cell nucleus.
- Nuclear Targeting and Integration: The T-complex, once formed, is directed to the nucleus of the plant cell, aided by nuclear targeting signals released by the Vir proteins. The T-DNA is integrated into the plant genome through recombination processes, typically occurring in transcriptionally active or repetitive regions. This integration can result in the establishment of either single-copy or multiple-copy insertions of the T-DNA.
- Opine Synthesis and Nutritional Advantage: In addition to transferring T-DNA, Agrobacterium also synthesizes opines, which are unique compounds that serve as a nutrient source for the bacteria. This nutritional relationship between the bacterium and the transformed plant cell is essential for the survival and proliferation of Agrobacterium within the plant tissue.
- Plant-Encoded Factors: Despite the detailed understanding of the molecular mechanisms governing T-DNA transfer and integration, the plant-encoded factors that facilitate this process remain less well characterized. Research into these factors is crucial, as they could play significant roles in determining the efficiency and success of Agrobacterium-mediated transformations.
AGROBACTERIUM
The genus Agrobacterium comprises several species known primarily for their role in plant pathology and genetic engineering. Although these species are often classified based on disease symptoms and host ranges, a more nuanced understanding reveals the complex relationship between the bacterial strains and their respective plasmids. The most notable species include Agrobacterium tumefaciens, A. rhizogenes, A. rubi, and the recently identified A. vitis. Each of these species is associated with specific plant diseases, such as crown gall disease caused by A. tumefaciens, hairy root disease caused by A. rhizogenes, and cane gall disease from A. rubi.
- Species and Disease Associations:
- Agrobacterium radiobacter is characterized as an “avirulent” species, while A. tumefaciens is responsible for crown gall disease.
- A. rhizogenes leads to hairy root disease, and A. rubi is associated with cane gall disease.
- The classification of A. vitis has emerged, which causes galls on grape and a few other plant species.
- Plasmid Influence on Symptoms:
- The pathogenicity of these bacteria largely hinges on the type of tumor-inducing plasmid they possess.
- For instance, A. tumefaciens strain C58, harboring the nopaline-type Ti plasmid (pTiC58), induces crown gall teratomas.
- Curing this plasmid renders the strain nonpathogenic, while introducing Ri plasmids can convert the bacterium into a rhizogenic strain.
- Notably, A. tumefaciens can be transformed into A. rhizogenes by substituting one oncogenic plasmid for another, highlighting the fluidity of species classification within this genus.
- Current Classification Challenges:
- The traditional classification based on species may not fully capture the diversity and functional differences among Agrobacterium strains.
- A more relevant classification may involve “biovars,” categorized by growth and metabolic characteristics.
- For example, biovar I primarily includes strains of A. tumefaciens and A. rubi, while biovar II encompasses A. rhizogenes, and biovar III is represented by A. vitis.
- Broad Host Range:
- A significant attribute of Agrobacterium is its ability to transfer DNA to a wide array of organisms, including numerous dicot and monocot angiosperm species and even gymnosperms.
- Furthermore, Agrobacterium has demonstrated the ability to transform various fungi, including yeasts, ascomycetes, and basidiomycetes. Remarkably, it has also been reported to transfer DNA to human cells.
- Genetic Determinants of Host Range:
- The host range for Agrobacterium strains is primarily dictated by the Ti plasmid, which contains specific virulence (vir) loci that govern the transformation efficiency across different plant species.
- For instance, virC and virF loci are critical for the development of crown gall tumors, while virH appears to influence the transformation of maize.
- However, the complexity of host range is becoming clearer, suggesting that multiple genetic factors from both the bacterium and the plant host contribute to the transformation process.
- Host Plant Interactions:
- The interaction between Ti plasmids and the bacterial chromosomal background significantly influences host range and tumorigenic potential.
- For example, the plasmid pTiBo542 exhibits different virulence based on the chromosomal background it inhabits, which affects its ability to induce tumors in various leguminous plants.
- Genetic Basis of Susceptibility:
- Susceptibility to crown gall disease has a genetic basis in various plant families, including cucurbits, peas, soybeans, and grapevines, along with ecotypes of Arabidopsis thaliana.
- This indicates that host plant genetics play a crucial role in the transformation process and can be leveraged in genetic engineering strategies.
Requirements for Agrobacterium-mediated Gene Transfer
Below are the key materials and reagents necessary for conducting Agrobacterium-mediated gene transfer effectively.
- Sterile 50 ml Plastic Tubes: These are vital for maintaining sterility and preventing contamination during the preparation and storage of solutions.
- Autoclave: This piece of equipment is essential for sterilizing media, tools, and glassware, ensuring a contamination-free environment critical for tissue culture work.
- Controlled Tissue Culture Rooms: Ideally maintained at 25°C, these rooms should have a light/dark cycle of 16 hours of light and 8 hours of darkness. Such controlled conditions promote optimal plant growth and development.
- Shaker Incubator: This device facilitates the mixing of cultures and promotes uniform growth of Agrobacterium and plant tissues, enhancing transformation efficiency.
- Vacuum Pump: A vacuum pump is utilized in various protocols, including the removal of air bubbles and improving the infiltration of Agrobacterium into plant tissues.
- Laminar Flow Hood: This equipment provides a sterile working environment essential for tissue culture procedures, minimizing the risk of contamination.
- Glassware: Various types of glassware, including beakers, cylinders, Petri dishes, Duran bottles, and flasks, are used for preparing and storing media and solutions, as well as for culturing tissues.
- Filter Paper: Used for filtering solutions, this ensures that any particulates or contaminants are removed from the culture media.
- Parafilm: This flexible film is often used to seal Petri dishes or tubes, preventing contamination and moisture loss during culture.
- Forceps and Scalpel: These tools are necessary for the precise handling and preparation of explants, ensuring clean cuts and minimal damage.
- Pipettes: Essential for accurate measurement and transfer of liquid reagents, pipettes are crucial for preparing media and solutions.
- Centrifuge: This device is used to separate components of mixtures based on density, often employed in various stages of sample preparation.
- Spectrophotometer: This instrument measures the absorbance of solutions, which is useful for quantifying nucleic acids and assessing the concentration of other substances in culture media.
- Tissue Culture Vessels: These are specifically designed containers for the growth and maintenance of plant tissues under sterile conditions.
- Surgical Blades: Used for making precise cuts in explants, these blades facilitate clean and accurate tissue preparation.
- Explant Material: Suitable explants can include stems, embryos, cotyledons, or other tissues, serving as the starting material for Agrobacterium-mediated transformation.
- Agrobacterium Strain: The specific strain of Agrobacterium utilized will depend on the plant species and transformation goals, impacting transformation efficiency.
- Sodium Hypochlorite (13%): Commonly used as a disinfectant, this reagent helps sterilize explants before transformation to prevent microbial contamination.
- B5 Medium: This nutrient medium supports plant tissue growth and provides essential macro and micronutrients.
- Agar: A gelling agent used in solid media to support the growth of tissues and microorganisms.
- Tryptone and Yeast Extract: These provide nitrogen and other nutrients, supporting the growth of Agrobacterium and enhancing its virulence.
- Sodium Chloride: Often included in culture media to maintain osmotic balance.
- Hydrochloric Acid (35%): Used for pH adjustments in culture media.
- Sterile Distilled Water: This is necessary for preparing all solutions and media, ensuring that no contaminants are introduced.
- Ethanol (75%): Utilized for surface sterilization of explants and equipment to maintain sterility.
- Sucrose: A carbohydrate source essential for energy supply in culture media.
- Abscisic Acid: This plant hormone can be used to promote specific physiological responses in plant tissues during transformation.
- Rifampicin and Kanamycin Monosulfate: These antibiotics are used to select transformed cells by suppressing the growth of non-transformed cells.
- Gellan Gum Powder: This is another gelling agent that can be used to solidify culture media.
- PCR Primer Star Mix: This reagent is crucial for amplifying specific DNA sequences during molecular analysis of transformed plants.
- Carbenicillin Disodium Salt: Employed to inhibit the growth of Agrobacterium after transformation, allowing for the selection of successfully transformed plant cells.
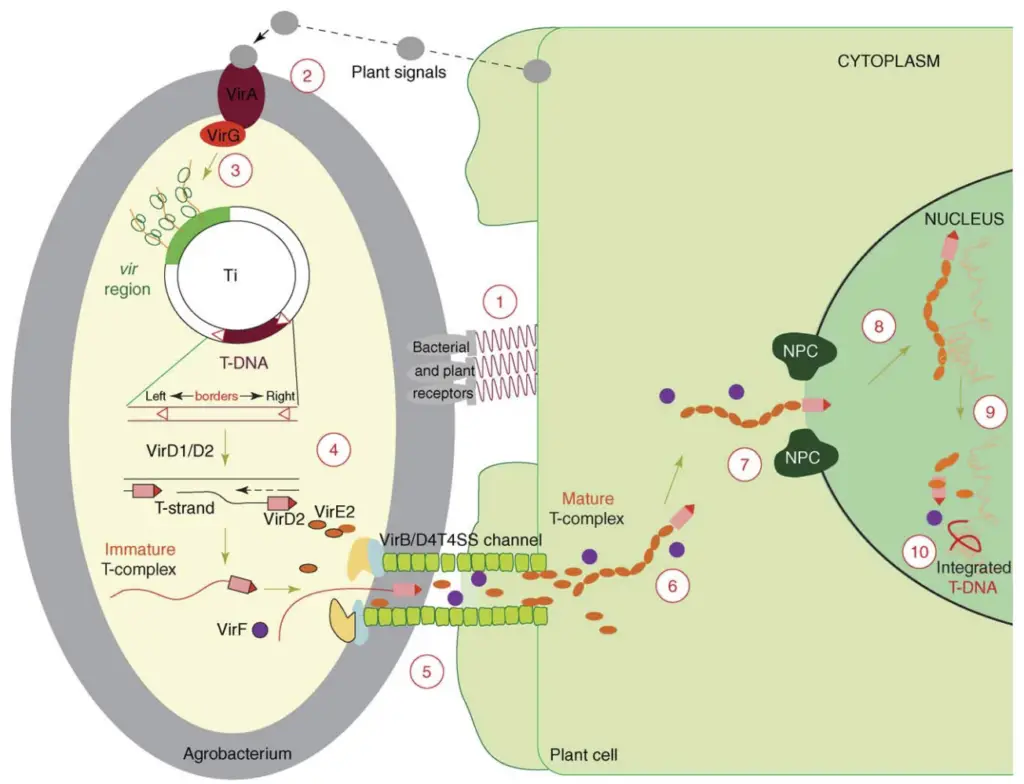
Steps for Media Preparation
The preparation of media for Agrobacterium-mediated gene transfer is a critical step in the transformation process. Each type of medium serves a specific function in supporting the growth of Agrobacterium, facilitating plant tissue culture, and ensuring the selection of transformed cells. Below is a detailed outline of the various media preparation processes necessary for successful Agrobacterium-mediated gene transfer.
- LB Medium for Agrobacterium Culture:
- Composition: The LB (Luria-Bertani) medium consists of 5 grams of yeast extract, 10 grams of tryptone, and 5 grams of sodium chloride dissolved in 1 liter of distilled water.
- Antibiotic Addition: To ensure the selection of appropriate Agrobacterium strains, 50 µl of a 100 mg/ml rifampicin stock and 50 µl of a 50 mg/ml kanamycin stock are added to 50 ml of the prepared LB medium.
- Function: This medium supports the growth of Agrobacterium, allowing it to multiply and remain viable for subsequent transformation steps.
- Murashige and Skoog Medium for Seed Germination:
- Preparation: To prepare the Murashige and Skoog (MS) basal medium, 4.43 grams of the MS basal medium powder and 3 grams of sucrose are added to 1 liter of distilled water.
- Gelling Agent: Subsequently, 2.5 grams of gellan gum powder is incorporated into the medium.
- Sterilization: The medium is then autoclaved to eliminate any contaminants.
- Plating: Finally, 25 ml of this medium is poured into sterile Petri plates under a laminar flow hood, creating a solid medium suitable for seed germination.
- Cocultivation Medium:
- Enhancement: The cocultivation medium is based on the MS basal medium. To this, 750 µl of a 2 mg/ml solution of 6-benzylaminopurine (BAP) is added to promote cell division and shoot initiation.
- Additional Hormone: Furthermore, 500 µl of a 2 mg/ml solution of abscisic acid (ABA) is included to facilitate stress responses and promote better growth conditions during the cocultivation phase.
- Preparation for Use: After mixing, 25 ml of this medium is also poured onto sterile Petri plates in a laminar flow hood to ensure sterility.
- Shooting Medium:
- Combination of Compounds: The shooting medium is prepared by adding 50 µl of a 50 mg/ml kanamycin stock and 2.5 ml of a 200 mg/ml carbenicillin stock to the cocultivation medium.
- Purpose: This medium is crucial for promoting shoot regeneration from the transformed plant tissues while simultaneously selecting for transformed cells using kanamycin.
- Rooting Medium:
- Preparation: The rooting medium involves the addition of 50 µl of a 50 mg/ml kanamycin stock and 1 ml of a 200 mg/ml carbenicillin stock to the medium used for rooting.
- Functionality: This medium supports the development of roots in regenerated shoots while maintaining selection pressure against untransformed cells.
Agrobacterium-mediated Gene Transfer Protocol
The procedure for Agrobacterium-mediated gene transfer involves a series of methodical steps that facilitate the introduction of foreign DNA into plant cells, particularly through the use of embryos as explants. Each step is designed to ensure the successful transformation of plant tissues while minimizing contamination and maximizing recovery rates. Below is a comprehensive protocol outlining the essential steps involved in this process.
- Sterilization and Germination of Seeds:
- Initial Sterilization: The seeds are sterilized by exposing them to chlorine gas (Cl₂) for a duration of 1 to 2 hours. This process eliminates surface contaminants that may interfere with subsequent steps.
- Soaking: After sterilization, the seeds are soaked in water for 2 hours at room temperature within a Petri dish to rehydrate.
- Seed Coat Removal: The seed coats are carefully removed using forceps, followed by a further sterilization with 75% ethanol for 30 seconds.
- Sodium Hypochlorite Rinse: The seeds are then rinsed with 20 ml of a 3% sodium hypochlorite solution to ensure complete disinfection.
- Germination: The sterilized seeds are placed on Petri plates containing seed germination medium and incubated at 28°C for 2 days in the dark. Each plate can accommodate approximately 15 to 25 seeds.
- Inoculum Preparation:
- Culture Inoculation: Two milliliters of LB medium containing rifampicin and kanamycin are inoculated with a single Agrobacterium colony.
- Incubation: The culture is incubated in a shaker incubator at 28°C to promote growth.
- Centrifugation: Following incubation, the Agrobacterium culture is centrifuged at 4000 g for 10 minutes. The supernatant is discarded, and the pellets are rinsed with 1 ml of MS liquid medium to prepare them for use in the transformation process.
- Preparation of Explants:
- Seed Removal: The germinated seeds are removed from the germination medium and placed on empty sterile Petri plates lined with filter paper.
- Cotyledon Preparation: The radicle is excised, and half of each cotyledon and the endopleura are removed from the seed.
- Separation and Collection: The cotyledons are separated using a sterile scalpel, thoroughly cleaned, and then collected into a sterile glass beaker containing MS liquid medium.
- Co-cultivation: The MS liquid medium with the cotyledons is combined with the Agrobacterium preparation and gently shaken.
- Vacuum Infiltration: The beaker is covered and sealed with film, then placed in a desiccator connected to a vacuum pump. The explants undergo two sessions of vacuum infiltration lasting 5 minutes each to facilitate the uptake of Agrobacterium.
- Placement on Co-cultivation Medium:
- Filter Paper Application: A sterile filter paper is positioned on the co-cultivation medium, and the infected explants are placed onto the filter paper with the adaxial surface of the cotyledons facing upwards.
- Incubation: The Petri plates are then sealed and incubated in the dark at 28°C for 2 days to allow for optimal integration of the Agrobacterium into the plant tissues.
- Shoot Initiation:
- Transfer to Shooting Medium: Following the co-cultivation period, the explants are transferred to a shooting medium that contains kanamycin and carbenicillin to inhibit the growth of Agrobacterium.
- Incubation Conditions: Each plate can accommodate 5 to 6 explants, which are then incubated under light for 2 to 3 weeks at 25°C to promote shoot development.
- Regeneration:
- Separation of Shoots: Once shoots begin to emerge, the explants are removed and placed on sterile Petri plates lined with filter paper.
- Cutting Shoots: The shoots are cut using a sterile scalpel, and the embryoid portion of the shoots is excised.
- Transferring to Rooting Medium: The shoots are then transferred to 100 ml glass flasks or tissue culture vessels containing rooting medium. Approximately 3 to 4 shoots can be placed in each vessel. The vessels are incubated under light for 1 to 2 weeks at 25°C.
- Monitoring Root Development: If roots do not appear after 2 weeks, the unfolded leaves and end portions of the shoots are cut away, and the shoots are transferred to a new rooting medium.
- Plant Acclimation:
- Initial Acclimation: Once roots start to develop, the covers on the flasks are loosened and further incubated at 25°C for 3 days.
- Transplanting: The plants are carefully removed from the medium and thoroughly washed with running water.
- Potting: Subsequently, the plants are transferred to pots filled with wet compost and watered adequately.
- Maintaining Humidity: To maintain moisture levels, the pots are covered with zip bags and incubated in a suitable growth chamber under light at 25°C for 1 to 2 weeks.
- Final Adjustment: Once the plants have established well, the zip bags are removed, and normal watering resumes.
Steps for Selection and Regeneration of Transgenic Plant
The process of selecting and regenerating transgenic plants involves several critical steps that ensure the successful integration and expression of transgenes. This method is essential for the advancement of plant biotechnology and requires meticulous planning and execution to enhance the chances of obtaining viable transgenic plants. The following outlines the sequential steps involved in the selection and regeneration process:
- Preparation of Transformation Medium:
- Begin by using antibiotics that do not adversely affect plant cells to eliminate or inhibit the growth of Agrobacterium tumefaciens. This step is crucial for ensuring that only transformed plant tissues can grow.
- Optimization of Regeneration Conditions:
- Conditions must be tailored for the adventitious organogenic regeneration of new plant tissues. This involves selecting appropriate nutrients and hormones that promote shoot formation and overall plant growth.
- Incorporation of Selective Agents:
- Introduce selective agents into the regeneration medium. These agents are designed to provide a genetic selection advantage to the transformed cells. For instance, genes encoding antibiotic resistance or herbicide resistance can be incorporated, allowing transformed cells to survive while untransformed cells perish in the presence of these selective agents.
- Significance of Genetic Selection:
- Genetic selection serves to preferentially enhance the growth of transformed cells. Without this selection, every regenerated shoot would require screening, which can be impractical, particularly in species with low transformation frequencies. Thus, genetic selection is vital for the efficiency of plant transformation protocols, often employing various marker genes to facilitate this process.
- Subculturing of Plant Disks:
- Transfer the infected disks (those exposed to A. tumefaciens) and half of the control disks (uninfected) to MSBN1.1 regeneration medium in 100 × 15 mm Petri dishes containing appropriate selective agents. This allows for the monitoring of non-transgenic regeneration under selection, providing a control for potential “escapes.”
- Control Evaluation:
- The remaining control disks should be placed in regeneration medium (MSBN1.1) that contains only the selective agent used to eliminate A. tumefaciens. This setup allows for an evaluation of overall regeneration frequency, giving insight into the effectiveness of the transformation process.
- Maintenance of Cultures:
- Cultures should be maintained at a temperature of 20°C under low light conditions (approximately 45 μE/m²s). Regular checks for contamination are essential, as any contamination can compromise the entire regeneration process. In cases of contamination, unaffected disks may be subcultured to fresh MSBN1.1 medium.
- Regular Subculturing:
- Every 2–3 weeks, all disks must be subcultured to fresh selection plates. Over time, the disks will expand and develop callus tissue, which is critical for further growth. Ensure that the expanded disks maintain good contact with the medium to promote effective nutrient uptake and growth.
- Observation of Shoot Development:
- After approximately 3–4 weeks, shoots will begin to appear from the cultured disks. These shoots indicate successful regeneration and can be further cultivated to develop into full transgenic plants.
Steps for Rooting of Transgenic Shoots to Recover Complete Plantlets
The rooting of transgenic shoots is a pivotal step in the recovery of complete plantlets from regenerated tissues. This phase not only facilitates the establishment of a stable root system but also serves as a crucial screening process to determine the success of transformation. The following outlines the systematic steps involved in rooting transgenic shoots, ensuring clarity and effectiveness throughout the procedure:
- Significance of Rooting in Transformation Screening:
- The rooting procedure is often the first meaningful test for confirming transformation because root organogenesis is typically more sensitive to selective agents than shoot regeneration. Therefore, shoots that fail to root under selection are likely not transgenic and should be discarded to maintain the integrity of the experiment.
- Preparation for Rooting:
- Carefully excise the regenerated shoots from the culture medium by using a sterile scalpel and forceps. It is essential to cut them at their base to prevent damage to the shoot tissue.
- Placement in Culture Vessels:
- Transfer the excised shoots into GA7 boxes, ensuring that about four shoots are placed per vessel. Fill each box with 50 mL of MSHF medium, supplemented with appropriate selective agents to maintain the selection pressure.
- Observation of Root Development:
- Monitor the shoots for root formation, which should become visible within approximately 10 days. This early observation is crucial for determining the health and viability of the shoots.
- Subculturing Rooted Shoots:
- Once roots are established, subculture only the rooted shoots. This involves cutting off the shoot with the top four internodes and transferring these sections individually into new GA7 boxes, again containing 50 mL of MSHF supplemented with the appropriate selective agents. These individual shoots can now be regarded as putative transformants.
- Maintenance and Propagation:
- Rooted shoots can be maintained and propagated to establish individual lines at monthly intervals. This process allows for the generation of a stable transgenic line that can be used for further research or breeding.
- Acclimatization and Transfer to Greenhouse:
- Alternatively, acclimatization of the rooted plants can be initiated. Once acclimatized, the plants can be transferred to a greenhouse environment, where they will have the opportunity to grow and produce seeds. The duration for seed setting can take about three months, depending on the environmental conditions and plant species.
Analysis of Transgenic Plants
The analysis of transgenic plants is a critical step in verifying that genetic modifications have been successfully incorporated and expressed within the plant tissues. This multi-faceted approach ensures that the desired traits have been effectively introduced and that the plants exhibit the expected characteristics. The following outlines the systematic procedures involved in the analysis of transgenic plants:
- Growth Stage for Analysis:
- Recovered transgenic plants are typically analyzed after they have grown sufficiently to provide ample tissue samples for testing. This stage is crucial to ensure that the analysis does not compromise the health and viability of the plants.
- Assay for Transgene Expression:
- Once the plants have reached an appropriate size, assays are conducted to evaluate transgene expression. This analysis is performed using methods tailored to the specific coding sequences of the transgenes and the anticipated outcomes. Various techniques can be employed, depending on the nature of the transgene and the type of expression desired.
- Polymerase Chain Reaction (PCR):
- In instances where direct assays for gene expression may be impractical or inconvenient, polymerase chain reactions (PCR) serve as a reliable alternative. PCR is a powerful technique that amplifies specific DNA sequences, allowing for the detection of the presence of transgenes within the plant’s genetic material.
- DNA Blotting Procedure:
- Following a positive PCR result, further confirmation is obtained through Southern blot analysis. This technique involves transferring DNA samples from an agarose gel onto a membrane, followed by hybridization with labeled probes specific to the transgene sequences. Southern blotting not only confirms the presence of transgenes but also provides information on their abundance within the plant’s genome.
- Analysis of Transgenic Characteristics:
- In addition to molecular analyses, it is essential to evaluate the phenotypic characteristics of the transgenic plants. Observations regarding growth patterns, resistance to specific pathogens, and other traits linked to the introduced genes are made to assess the effectiveness of the genetic modification.
- Comprehensive Validation:
- The combination of molecular analyses (PCR and Southern blotting) with phenotypic assessments provides a comprehensive validation of the transgenic plants. This thorough evaluation ensures that the plants not only contain the desired transgenes but also express them appropriately, contributing to the overall success of the genetic engineering project.
Factors influencing the efficiency of agrobacterium-mediated gene transfer
Agrobacterium-mediated gene transfer is a widely utilized technique for creating transgenic plants, relying on the natural abilities of the bacterium Agrobacterium tumefaciens. The efficiency of this transformation process is influenced by several critical factors that can enhance or inhibit successful gene transfer. Understanding these factors is essential for optimizing transformation protocols in agricultural biotechnology.
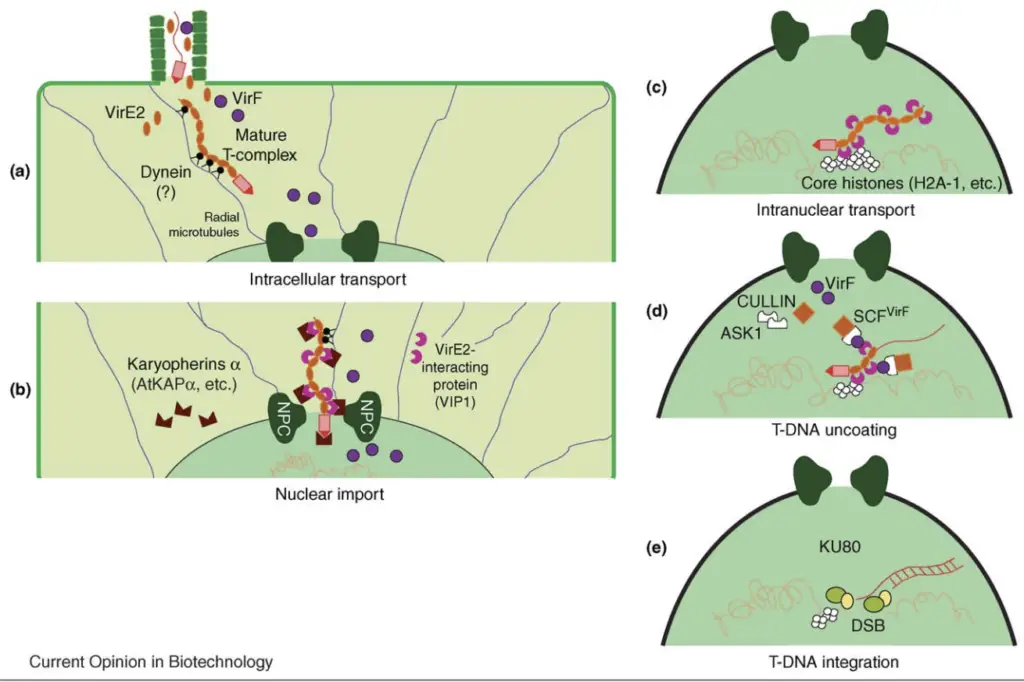
- Explants: Explants serve as the target material for transformation and can include embryonic cultures, immature embryos, mature seed derivatives, leaf blades, and stem segments. The selection of suitable explants is vital for recovering whole transgenic plants. Research has shown that embryonic callus derived from mature seeds is among the best explants for Agrobacterium-mediated transformation in specific plant species. Additionally, desiccating explants before or after infection with Agrobacterium has been found to improve transformation rates. Variations in transformation efficiency across different plant tissues may be attributed to the bacteria’s attachment capability and the T-DNA transfer mechanism.
- Explants Wounding: Wounding of explants is necessary for efficient Agrobacterium-mediated transformation. The method and extent of wounding can vary, encompassing simple cuts during preparation to more sophisticated techniques like particle gun-mediated micro-wounding. Other methods include using Agrobacterium-filled syringes or sonication. The creation of micro-wounds on the explants enhances transformation by facilitating the release of phenolic compounds, which can further support the process. The use of additional phenolic substances can also promote transformation efficiency.
- Plant Species and Genotype: The transformation efficiency varies significantly among different plant species due to differences in inducer molecules necessary for Agrobacterium infection. The chromosomal and plasmid genomes of the plant must encode all materials essential for bacterial attachment and DNA transfer. Variability in virulence gene expression can affect Agrobacterium’s ability to infect different plant species. Even among cultivars within the same species, susceptibility to Agrobacterium can differ, impacting tumorigenesis. Although traditionally more successful in dicotyledonous species, advancements have increased gene transfer frequencies in monocotyledonous species.
- Antibiotics: After co-cultivation with Agrobacterium, it is crucial to suppress bacterial growth to prevent interference with the development of the host plant. This is typically achieved through the application of antibiotics, such as carbenicillin and cefotaxime, in the culture medium. The choice of antibiotics must align with the specific plant species and Agrobacterium strains used, necessitating the determination of an optimal antibiotic ratio to balance selection and tumorigenesis rates.
- Plant Growth Regulators (PGR): The incorporation of plant growth regulators into the transformation process is essential for success. The presence of PGRs can significantly enhance the competence and susceptibility of explants to Agrobacterium infection. For instance, the inclusion of 2,4-D in the growth medium during co-cultivation is known to boost transformation efficiency. Growth regulators facilitate essential cellular processes, including division and differentiation, but their application must align with specific stages in the plant cell cycle.
- Light: Light conditions significantly influence the efficiency of Agrobacterium-mediated transformation by affecting various physiological factors, including hormone levels, cell proliferation, and the cell cycle. Light exposure can increase the production of phenolic compounds, which serve as virulence inducers for Agrobacterium, thereby enhancing T-DNA transfer. Consequently, many transformation protocols utilize dark co-culture conditions to optimize explant morphology, although the overall effect of light may depend on the specific photoperiod employed.
- Temperature: Temperature plays a critical role in the efficiency of Agrobacterium-mediated transformation. Elevated temperatures have been found to inhibit tumor development, with optimal transfer temperatures typically ranging from 19°C to 22°C for many plant species. Research indicates that temperatures around 32°C can suppress tumor development due to conformational changes in virulence genes. However, the ideal temperature may vary among different plant species, necessitating careful calibration.
- Agrobacterium Strains: The infectivity of various Agrobacterium strains differs based on the presence of specific plasmids. The most effective transformation results are achieved using a combination of a standard binary vector in a super-virulent strain alongside a super-binary vector in a regular strain. Consequently, selecting appropriate combinations of Agrobacterium strains is crucial for enhancing transformation efficiency across different plant cell types.
Applications
The following outlines some of the key applications of Agrobacterium-mediated transformation.
- Production of Biologically Active Compounds:
- Agrobacterium-mediated transformation is utilized to genetically modify plants for the production of valuable substances, including proteins, antibodies, and vaccines.
- This method facilitates the creation of transgenic plants that can express complex proteins which may otherwise be difficult to produce in traditional systems.
- Development of Pharmaceutical Products:
- Many plants have been genetically engineered to produce life-saving pharmaceutical products such as anticoagulants, human epidermal growth factors, and interferons.
- These transgenic plants serve as biofactories, allowing for the sustainable production of important therapeutic agents that can be used in medical treatments.
- Bioremediation and Environmental Monitoring:
- Transgenic plants generated through Agrobacterium-mediated transformation can function as biomonitors to detect toxic compounds in the environment.
- Additionally, they can be designed to detoxify contaminated soil and water, thus contributing to bioremediation efforts and enhancing environmental health.
- Enhancing Crop Yields:
- The technique has led to significant increases in crop yields through modifications that improve the shelf life and biosynthesis of important agricultural plants.
- For example, transgenic crops may exhibit enhanced resistance to environmental stresses, which can contribute to greater agricultural productivity.
- Improving Stress Tolerance:
- Agrobacterium-mediated transformation allows for the enhancement of plant tolerance against various biotic and abiotic stress factors.
- Genetic modifications can lead to improved nutrient capture and increased resistance to pests, which is crucial for sustainable agriculture.
- Insect Resistance Development:
- One of the most notable applications of Agrobacterium-mediated transformation is the production of insect-resistant crops through the incorporation of genes encoding toxic proteins, such as those derived from the bacterium Bacillus thuringiensis (Bt toxin).
- The implementation of these modifications results in crops that are more resilient to insect pests, thereby reducing the need for harmful agrochemicals and herbicides.
- Simplicity and Versatility:
- Agrobacterium-mediated transformation is recognized as one of the less complicated genetic engineering techniques, offering ease of use compared to other methods.
- Its versatility extends beyond plant systems, indicating potential for future applications in genetic modifications of other organisms.
Limitations
The following outlines some key limitations associated with this technique:
- Narrow Host Range:
- One of the primary limitations of Agrobacterium-mediated transformation is its narrow host range.
- The technique is restricted to specific plant species, which may limit its applicability in broader agricultural contexts.
- Limited Understanding of Plant Factors:
- Despite advances in understanding the mechanism of T-DNA transfer by Agrobacterium, the role of plant-encoded factors that influence the efficiency of this process remains inadequately understood.
- This gap in knowledge can hinder efforts to optimize transformation protocols across various plant species.
- Labor-Intensive Procedures:
- The technique is inherently labor-intensive, requiring the development of robust plant regeneration protocols.
- These protocols often involve detailed and time-consuming processes that can be prone to variations when conducted in vitro, potentially leading to unfavorable or inconsistent results.
- Dependence on Specific Explants:
- In the case of monocotyledonous plants, the success of Agrobacterium-mediated transformation is often contingent upon the availability of embryos as explants.
- However, embryos are typically available for a limited duration during the growing season, which can constrain the timing of transformation efforts.
- Limitations in DNA Size Transfer:
- Agrobacterium-mediated transformation is restricted in its capacity to transfer large DNA molecules into plants.
- This limitation suggests the need for the development of alternative vector systems that can accommodate the introduction of larger genetic constructs, which may be necessary for certain applications in genetic engineering.
- Gelvin SB. Agrobacterium-mediated plant transformation: the biology behind the “gene-jockeying” tool. Microbiol Mol Biol Rev. 2003 Mar;67(1):16-37, table of contents. doi: 10.1128/MMBR.67.1.16-37.2003. PMID: 12626681; PMCID: PMC150518.
- Hau-Hsuan Hwang, Manda Yu, Erh-Min Lai “Agrobacterium-Mediated Plant Transformation: Biology and Applications,” The Arabidopsis Book, 2017(15), (20 October 2017)
- Lacroix B, Citovsky V. Pathways of DNA Transfer to Plants from Agrobacterium tumefaciens and Related Bacterial Species. Annu Rev Phytopathol. 2019 Aug 25;57:231-251. doi: 10.1146/annurev-phyto-082718-100101. Epub 2019 Jun 21. PMID: 31226020; PMCID: PMC6717549.
- Tzfira, T., & Citovsky, V. (2006). Agrobacterium-mediated genetic transformation of plants: biology and biotechnology. Current Opinion in Biotechnology, 17(2), 147–154. doi:10.1016/j.copbio.2006.01.0
- https://mgcub.ac.in/pdf/material/202004260029504668c533f4.pdf
- https://www.davuniversity.org/images/files/study-material/Agrobacterium-mediated%20gene%20transfer.pdf
- https://www.slideshare.net/slideshow/agrobacterium-mediated-gene-transfer-77002058/77002058
- https://goldbio.com/articles/article/a-quick-overview-of-agrobacterium-for-plant-transformation
- https://www.scielo.cl/scielo.php?script=sci_arttext&pid=S0717-34581998000300002
- https://www.scielo.cl/fbpe/img/ejb/v1n3/1/bip/
- https://www.mybiosource.com/learn/testing-procedures/agrobacterium-mediated-gene-transfer/