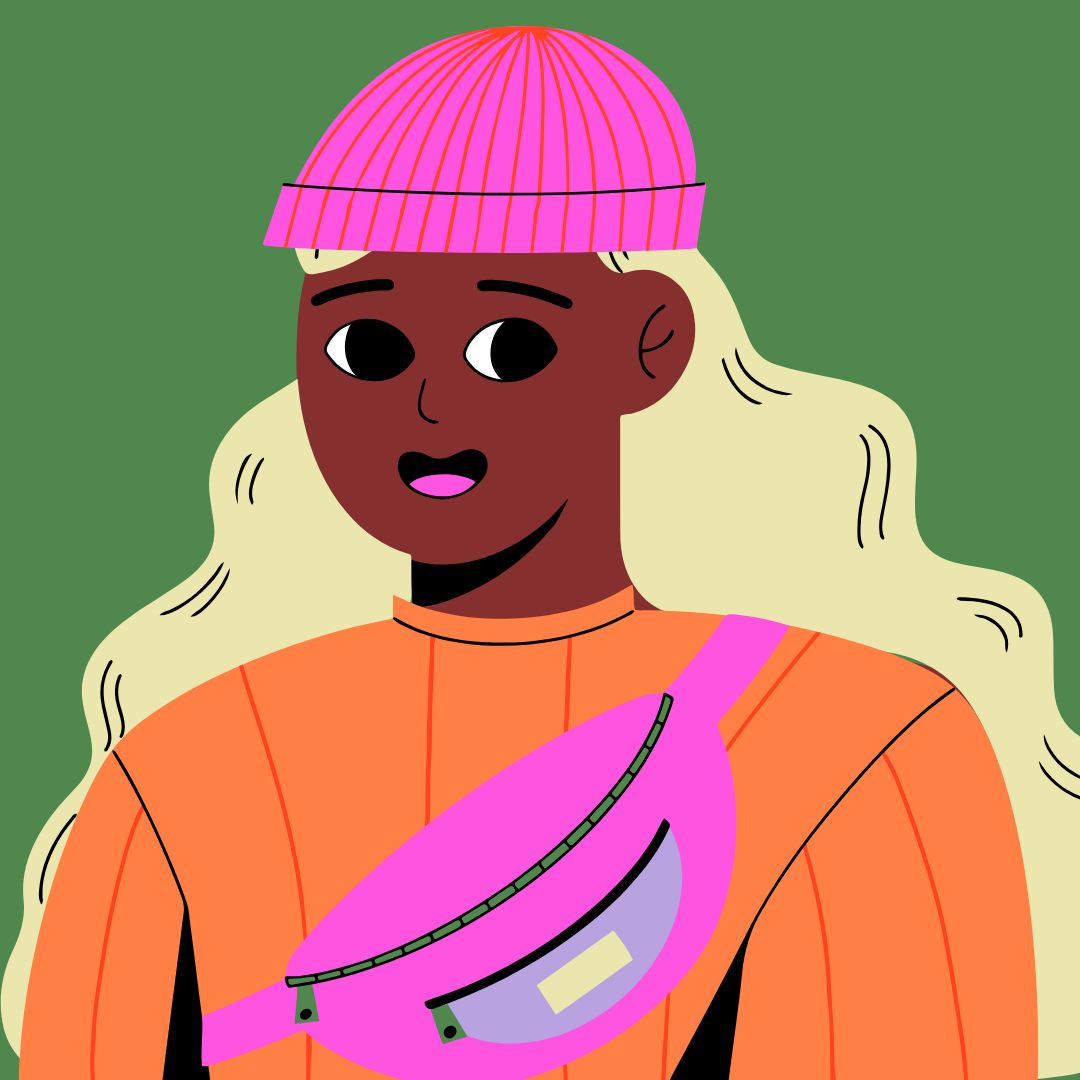
Sourav Pan
Transcript
Simple diffusion is the movement of molecules directly across a semi-permeable membrane without the help of transport proteins.
The cell membrane is composed of a phospholipid bilayer that acts as a selective barrier.
Small, nonpolar molecules like oxygen and carbon dioxide can pass through the membrane directly through a process called simple diffusion.
These small molecules can slip between the phospholipids in the membrane without requiring any additional proteins.
However, larger molecules like glucose cannot pass through the membrane via simple diffusion.
Several factors affect simple diffusion, including molecule size and polarity, concentration gradient strength, membrane properties, and temperature.
Remember, simple diffusion is a passive process that requires no energy input. Molecules naturally move from areas of high concentration to areas of low concentration.
Facilitated diffusion is a process that allows molecules to cross cell membranes with the help of transport proteins.
The cell membrane consists of a phospholipid bilayer, which creates a barrier that some molecules cannot easily cross.
Transport proteins embedded in the membrane create channels that allow specific molecules to pass through.
Small, uncharged molecules can often diffuse directly through the phospholipid bilayer.
However, larger molecules or charged particles cannot pass directly through the membrane. They require transport proteins.
Charged molecules also use these protein channels to cross the membrane, following their concentration gradient.
Importantly, facilitated diffusion does not require energy input. Molecules still move down their concentration gradient from high to low concentration.
Transport proteins are highly selective, allowing only specific molecules to pass. This process is generally faster than simple diffusion for the molecules that need assistance.
In summary, facilitated diffusion allows molecules that cannot cross the membrane directly to move across with the help of transport proteins, all without using energy.
Osmosis is a special type of diffusion that specifically involves the movement of water molecules across a semi-permeable membrane.
In osmosis, water moves from areas of lower solute concentration to areas of higher solute concentration.
This movement continues until the concentration of water molecules reaches equilibrium on both sides of the membrane. The solute particles cannot cross the semi-permeable membrane.
We can demonstrate osmosis using a U-tube separated by a semi-permeable membrane. When pure water is placed on one side and a sugar solution on the other, water molecules move toward the sugar solution.
Osmosis is crucial for cells to maintain proper water balance. It prevents cells from shrinking or bursting, and controls turgor pressure in plant cells.
Dialysis is a specialized type of diffusion where a semi-permeable membrane allows some solutes to pass through while blocking others.
The membrane contains tiny pores that act as molecular filters, allowing small waste molecules to pass while blocking larger essential molecules.
Small waste molecules can freely diffuse through the membrane pores, while larger essential molecules are too big to pass through.
This selective diffusion principle is the foundation of kidney dialysis, a life-saving medical treatment for patients with kidney failure.
In a dialysis machine, blood flows through semi-permeable tubes inside a dialyzer. A special fluid called dialysate surrounds these tubes.
Waste molecules like urea and creatinine diffuse from the blood into the dialysate due to concentration gradients, while essential proteins and cells remain in the bloodstream.
This process essentially performs the filtering function of healthy kidneys. The cleaned blood is then returned to the patient, while the waste-filled dialysate is discarded.
Dialysis demonstrates selective diffusion through a semi-permeable membrane, allowing small waste molecules to pass while retaining essential components. This principle saves countless lives every year.
Diffusion occurs because of the random movement of molecules.
At the molecular level, all particles possess kinetic energy, causing them to be in constant motion.
Let’s visualize a chamber with a high concentration of molecules on one side and a low concentration on the other.
The random motion of molecules causes them to move in unpredictable directions, continuously colliding with each other and their surroundings.
As molecules move randomly, they naturally spread from areas of high concentration to areas of low concentration.
This doesn’t mean molecules move in only one direction. Rather, the net movement of molecules is from high to low concentration areas due to statistical probability.
Eventually, the random motion leads to an even distribution of molecules throughout the container – a state of equilibrium.
It’s important to understand that diffusion is driven by this random motion – molecules don’t ‘know’ where to go, they simply move randomly, and the concentration gradient results in the net movement we observe.
Thus, the random movement of molecules due to their kinetic energy is the fundamental mechanism driving the process of diffusion.
Molecular collisions play a key role in the diffusion process.
Let’s examine a system with a higher concentration of particles on the left side, and fewer particles on the right.
As particles move randomly, they collide with each other and change direction. These collisions are fundamental to understanding how diffusion works.
In areas of higher concentration, particles are closer together, leading to more frequent collisions.
These collisions push particles toward less crowded areas, creating a net movement down the concentration gradient.
When particles collide, several effects occur: they change direction, transfer energy, and contribute to the overall movement from high to low concentration.
This molecular-level understanding of collisions helps explain why substances naturally diffuse from areas of high concentration to areas of low concentration.
Temperature plays a crucial role in how quickly molecules diffuse.
Let’s compare how diffusion happens at different temperatures.
Higher temperatures increase the kinetic energy of molecules.
Molecules at higher temperatures move much faster than those at lower temperatures.
As a result, diffusion happens much more quickly at higher temperatures.
The relationship between temperature and diffusion rate is not linear. As temperature increases, diffusion rate increases more rapidly.
Temperature change is one of the most effective ways to control diffusion rates in scientific applications and natural processes.
Particle size has a significant impact on how quickly substances diffuse through a medium.
To understand this relationship, let’s observe how particles of different sizes move through the same medium.
Here we have small particles, like oxygen molecules, which are crucial for cellular respiration.
And here are larger particles, such as glucose molecules, which provide energy for cells.
When diffusion begins, we can observe a clear difference in how quickly these particles move.
Smaller particles diffuse faster because they encounter less resistance when moving through the medium.
They also have higher mobility, allowing them to navigate more easily between molecules in the medium.
As a result, smaller particles consistently diffuse faster than larger ones in the same environment.
At the molecular level, the medium itself consists of particles that create resistance.
Larger particles, like glucose, experience more frequent collisions with medium molecules, slowing their movement.
Smaller particles, like oxygen, can navigate through the spaces between medium molecules more easily, allowing for faster diffusion.
This fundamental principle explains why gases like oxygen diffuse rapidly in tissues, while larger molecules like proteins diffuse very slowly.
The properties of the medium through which diffusion occurs significantly affect how quickly particles move.
Let’s first examine viscosity, which measures a fluid’s resistance to flow.
We can compare diffusion in a low viscosity medium like water with a high viscosity medium like honey.
Let’s observe how the same particles diffuse through these different media.
In water, with its low viscosity, particles move rapidly and freely.
But in honey, the high viscosity restricts movement, causing particles to diffuse much more slowly.
This demonstrates an important principle: as viscosity increases, the rate of diffusion decreases.
Now let’s examine how density affects diffusion. Density is the mass per unit volume of a substance.
Diffusion occurs at different rates in gases, liquids, and solids due to their different densities.
Let’s observe how the same particles diffuse through these different states of matter.
In gases, particles are widely spaced and move rapidly in random directions, allowing for very fast diffusion.
In liquids, particles are closer together, reducing their mobility and slowing diffusion.
In solids, particles are tightly packed in fixed positions, allowing for very little movement, making diffusion extremely slow.
This demonstrates another important principle: as density decreases, the rate of diffusion increases.
To summarize what we’ve learned: The properties of the medium have a significant impact on diffusion rates. Higher viscosity slows diffusion by increasing resistance to movement. Lower density speeds up diffusion by allowing for greater molecular movement. The same substance will diffuse at different rates depending on the medium it’s traveling through.
The diffusion of tea in water is a perfect example of how compounds move from areas of high to low concentration.
We start with a cup of water and a tea bag containing dried tea leaves.
The tea leaves contain color and flavor compounds at high concentration, while the water initially has none.
When we place the tea bag in hot water…
…the compounds begin to diffuse from the tea bag into the surrounding water.
As time passes, the concentration of tea compounds in the water increases.
The process continues until the tea reaches a uniform color and flavor throughout the water.
Temperature plays a crucial role in this process. Higher temperatures increase the kinetic energy of molecules, making them move faster.
In hot water, tea diffuses much more quickly than in cold water, which is why we typically use hot water for brewing tea.
This diffusion process follows the universal principle of molecules moving from areas of high concentration to low concentration until equilibrium is reached.
When sugar is added to coffee, it dissolves and diffuses throughout the liquid.
Initially, the sugar concentration is highest at the bottom where the crystals are added.
At the molecular level, sugar molecules start to disperse from areas of high concentration to areas of low concentration.
Without stirring, sugar molecules slowly diffuse through random molecular movement.
This process can take several minutes to reach an even distribution throughout the coffee.
When we stir the coffee, the diffusion process is drastically accelerated.
Stirring creates currents that rapidly carry sugar molecules throughout the liquid, achieving an even distribution in seconds rather than minutes.
Whether through natural diffusion or with the help of stirring, sugar molecules ultimately reach equilibrium and distribute evenly throughout the coffee.
Understanding this process helps explain why your coffee eventually tastes sweet throughout, even if you don’t stir it.
Cellular respiration depends heavily on diffusion for gas exchange between cells and the bloodstream.
Cells are surrounded by blood vessels that carry oxygen and remove carbon dioxide.
In this gas exchange system, diffusion drives the movement of molecules across the cell membrane.
Inside cells, mitochondria are the primary sites of cellular respiration.
The bloodstream maintains a high concentration of oxygen molecules around the cell.
Meanwhile, cellular respiration within the cell produces carbon dioxide.
Following the concentration gradient, oxygen diffuses from high concentration in the bloodstream to low concentration inside the cell.
The mitochondria use this oxygen in cellular respiration to produce ATP, the energy currency of the cell.
As cellular respiration produces carbon dioxide, it builds up inside the cell, creating a concentration gradient.
Carbon dioxide then diffuses from high concentration inside the cell to low concentration in the bloodstream.
The bloodstream carries away this carbon dioxide, which is eventually exhaled through the lungs.
This continuous exchange of oxygen and carbon dioxide through diffusion is essential for sustaining cellular energy production.
Without this diffusion-driven gas exchange, cellular respiration would cease, and cells would rapidly die from energy depletion and waste buildup.
Plants obtain essential nutrients from the soil through their root systems.
Let’s look at a cross-section of a plant root. The epidermis is the outermost layer with root hairs that increase surface area for absorption.
Inside we find the cortex, where much of the diffusion occurs, and the central stele that contains vascular tissues for transporting nutrients throughout the plant.
Nutrient uptake depends on concentration gradients. The soil solution typically has a higher concentration of mineral ions than root cells.
This concentration gradient drives diffusion. Mineral ions naturally move from an area of higher concentration in the soil to an area of lower concentration inside the root cells.
Root hair cells actively maintain this gradient by quickly moving absorbed nutrients into the inner tissues, allowing continuous diffusion to occur.
This passive diffusion of minerals from soil to roots is essential for plant nutrition, providing elements like nitrogen, phosphorus, and potassium needed for growth and development.
Diffusion plays a vital role in chemical reactions by bringing reactant molecules into contact with each other.
In a chemical reaction, molecules must first come into contact before they can react.
Through random thermal motion, molecules diffuse throughout the solution or gas, eventually coming into contact with other reactants.
When reactants meet, chemical bonds can form, creating new products. The rate of these reactions is often limited by how quickly the molecules can diffuse to the reaction site.
In many reactions, the diffusion of reactants is the rate-limiting step. This means the overall speed of the reaction depends on how quickly molecules can diffuse to each other.
Factors like temperature, molecular size, and medium viscosity affect diffusion rates.
In environments with faster diffusion, reactions proceed more rapidly as reactants meet more frequently.
Understanding diffusion is crucial in both industrial processes and laboratory settings.
Engineers design reactors to optimize diffusion by controlling temperature, increasing surface area, and reducing diffusion distances. In laboratories, techniques like stirring accelerate reactions by enhancing molecular encounters.
To summarize, diffusion is often the key limiting factor in chemical reaction rates. By understanding and controlling diffusion, scientists and engineers can optimize reaction efficiency in both laboratory and industrial settings.
In this classic diffusion experiment, we’ll observe how a drop of food coloring spreads in still water.
Let’s follow these steps to observe diffusion in action.
We start by adding a single drop of food coloring to the top of the water.
At first, the color remains concentrated in one area, creating a region of high concentration.
After thirty seconds, we can observe the color beginning to spread out slightly.
After two minutes, the diffusion continues as more molecules move randomly throughout the water.
If we observe the experiment from multiple angles, we can see how the food coloring spreads in three dimensions.
This simple experiment clearly demonstrates how molecules naturally move from areas of high concentration to areas of low concentration, without requiring any external energy.
This random molecular movement, resulting in the even distribution of particles, is the essence of diffusion.
Understanding diffusion principles has enabled countless innovations that improve our lives, from life-saving medical treatments to the devices that power our digital world.
Study Materials
No study materials available for this video.
Helpful: 0%
Related Videos
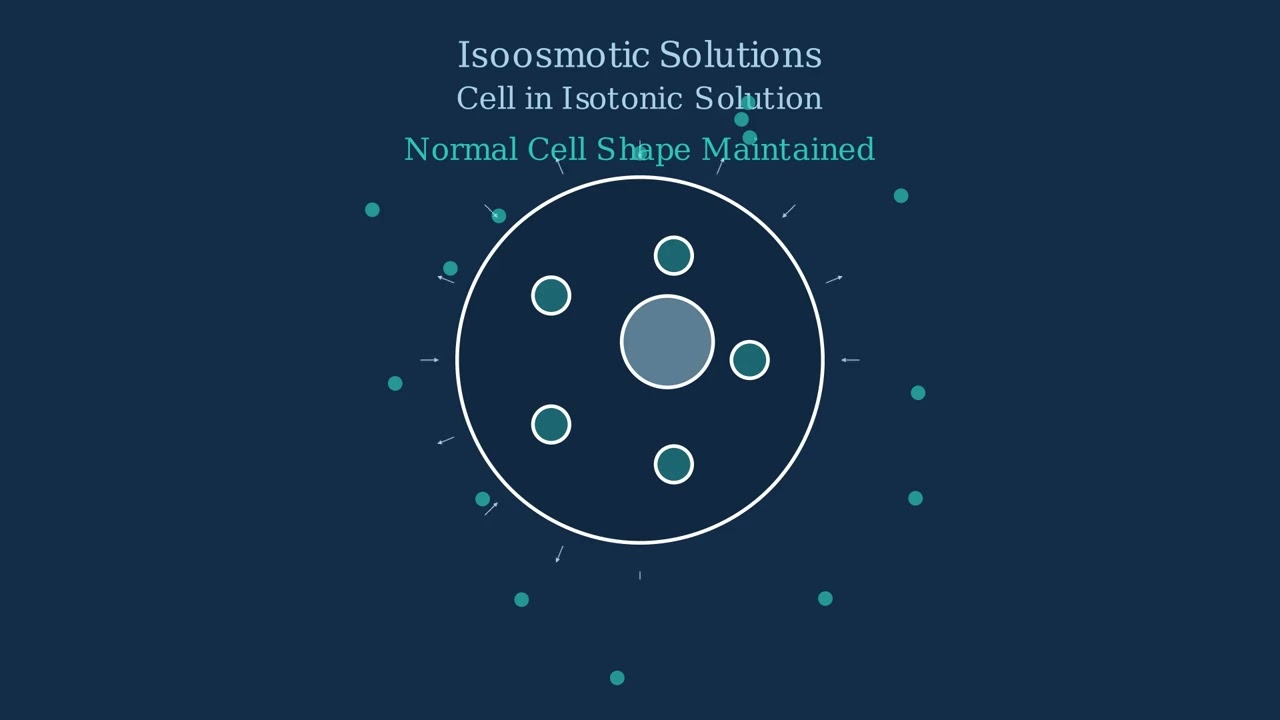
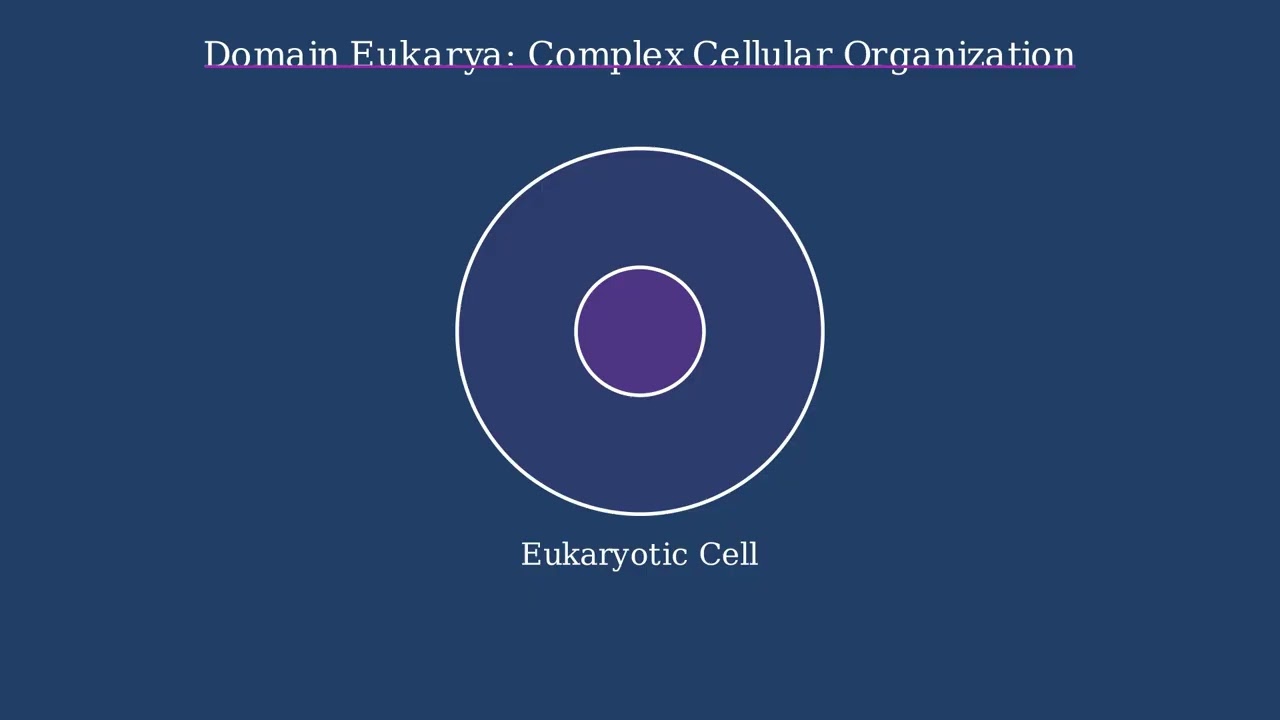
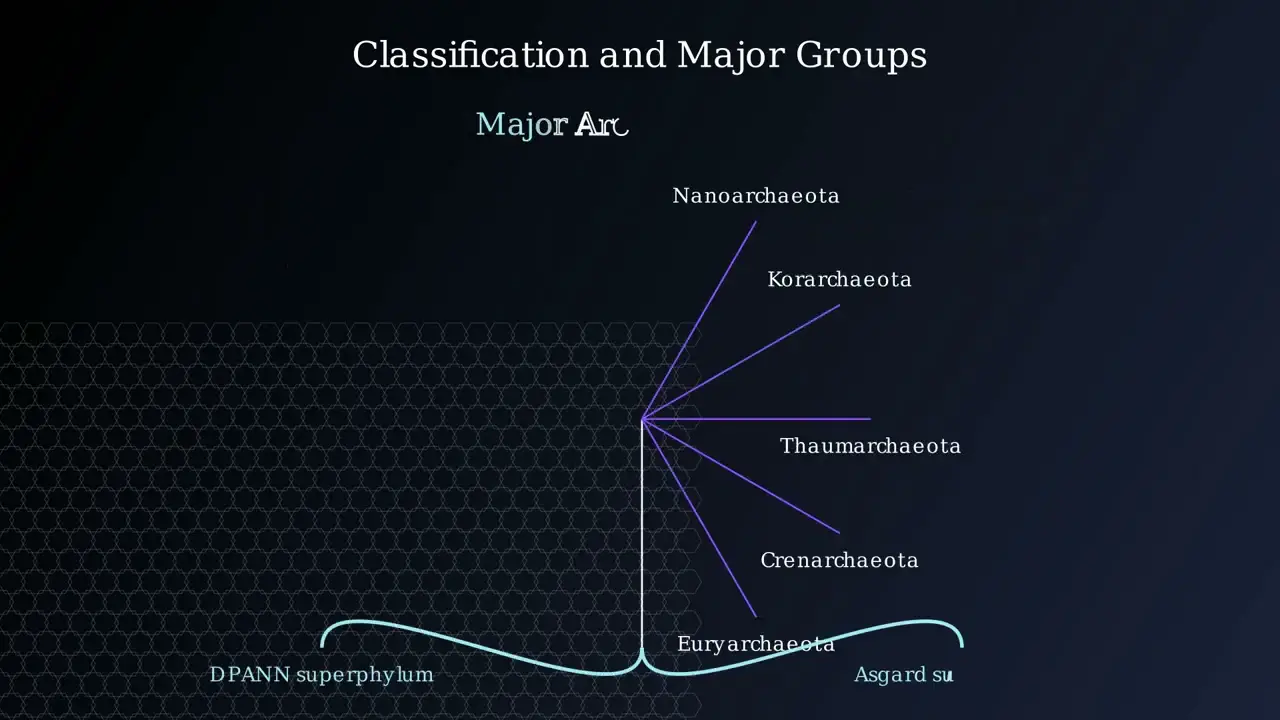
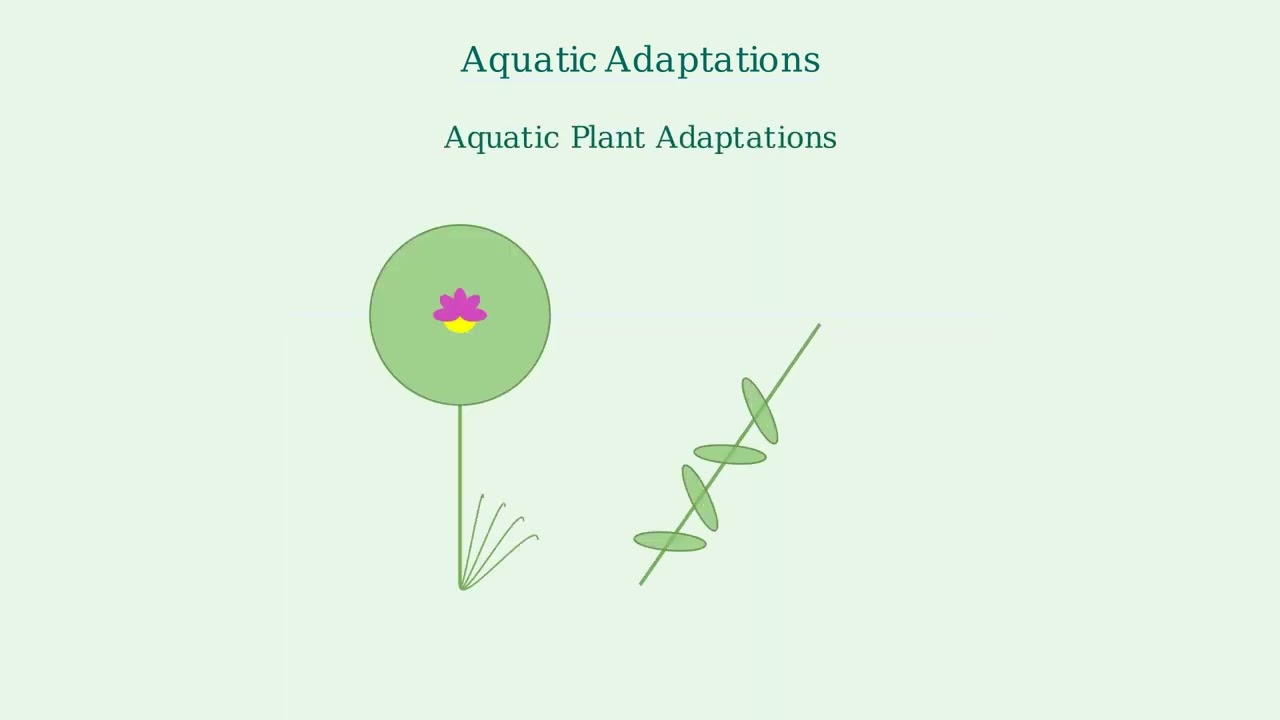
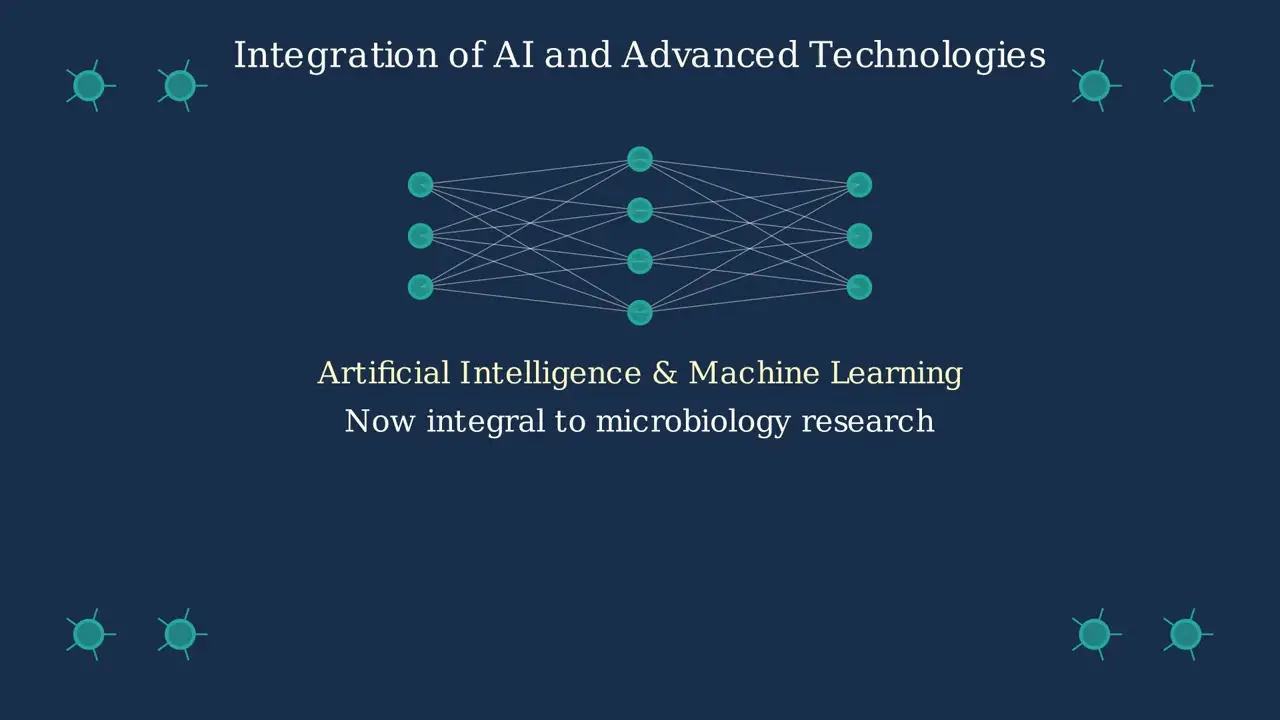
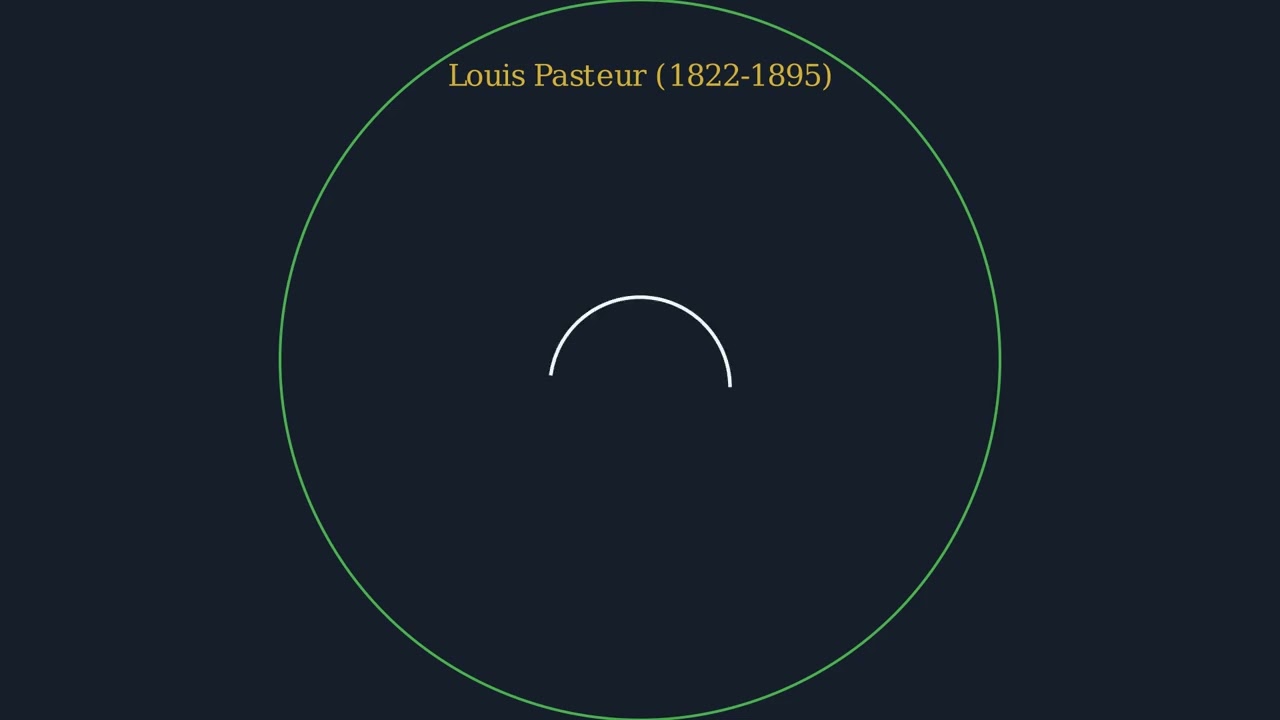
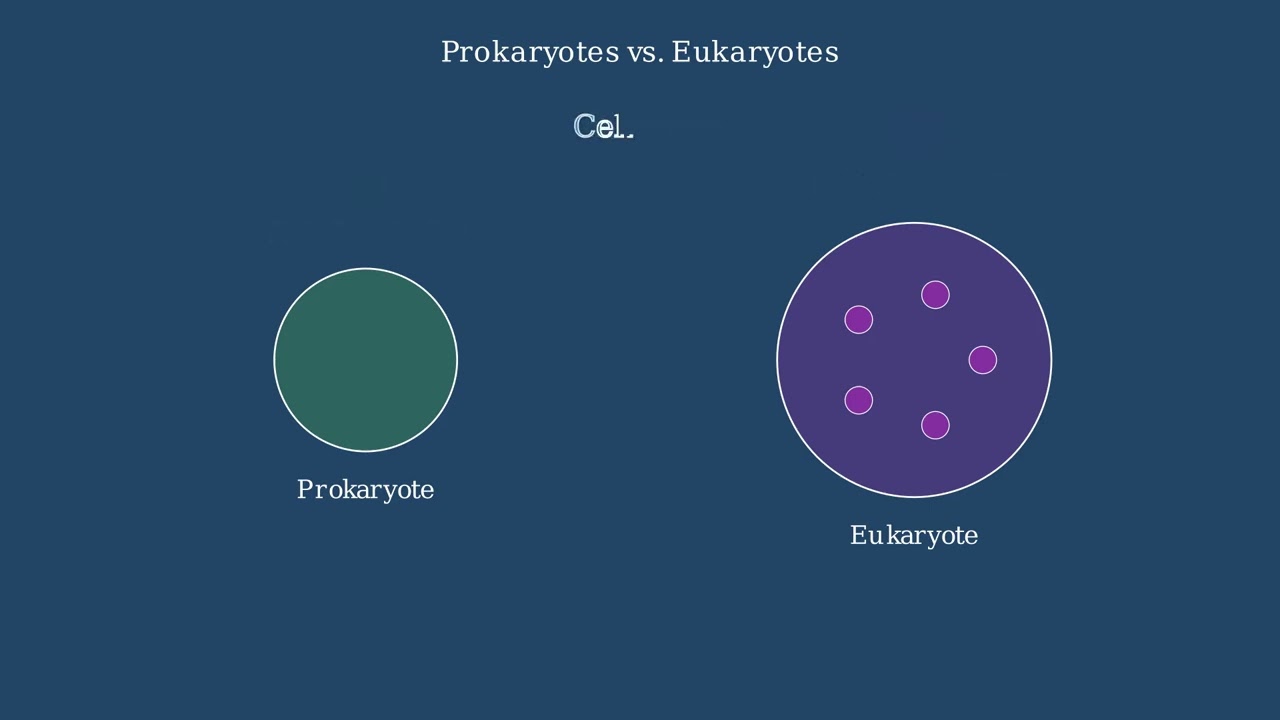
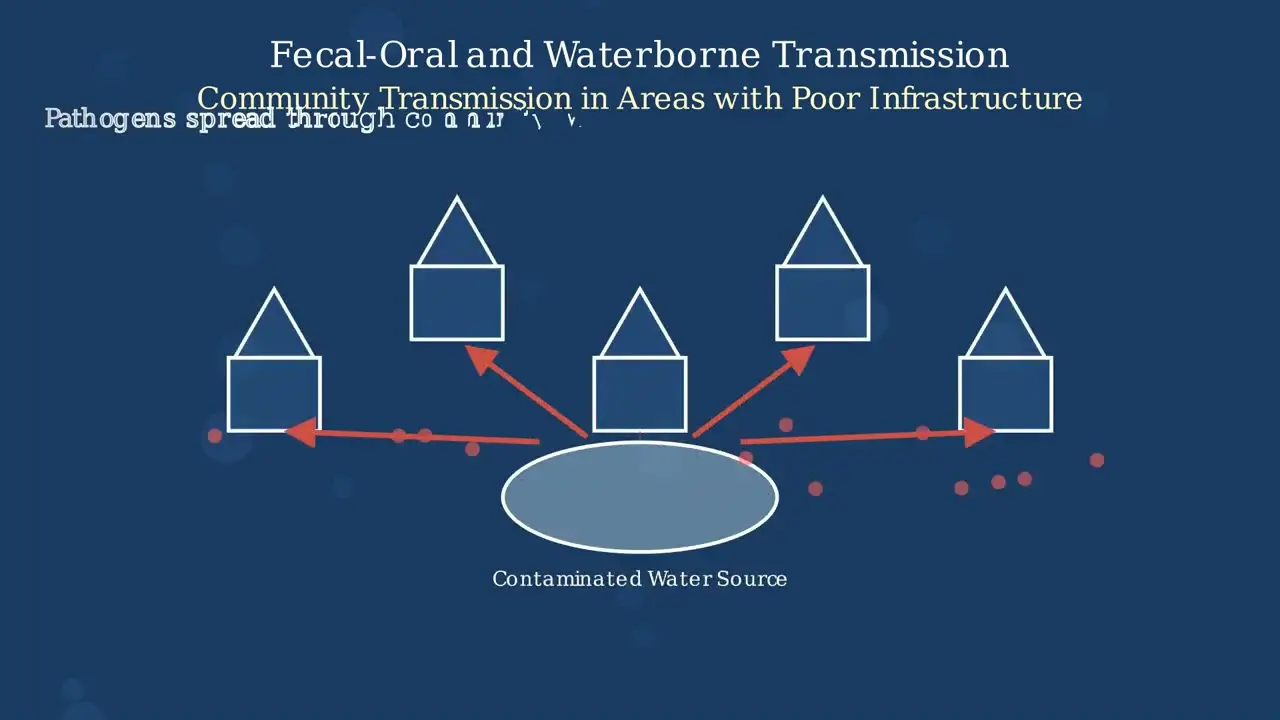
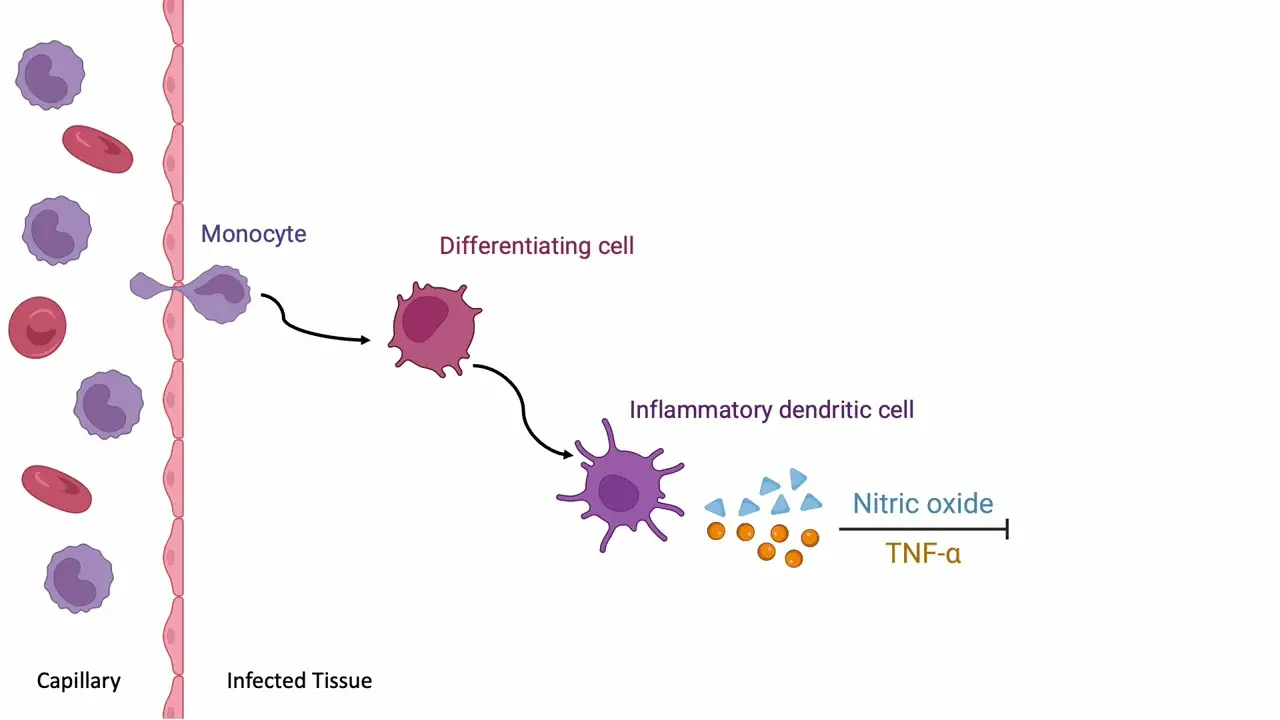