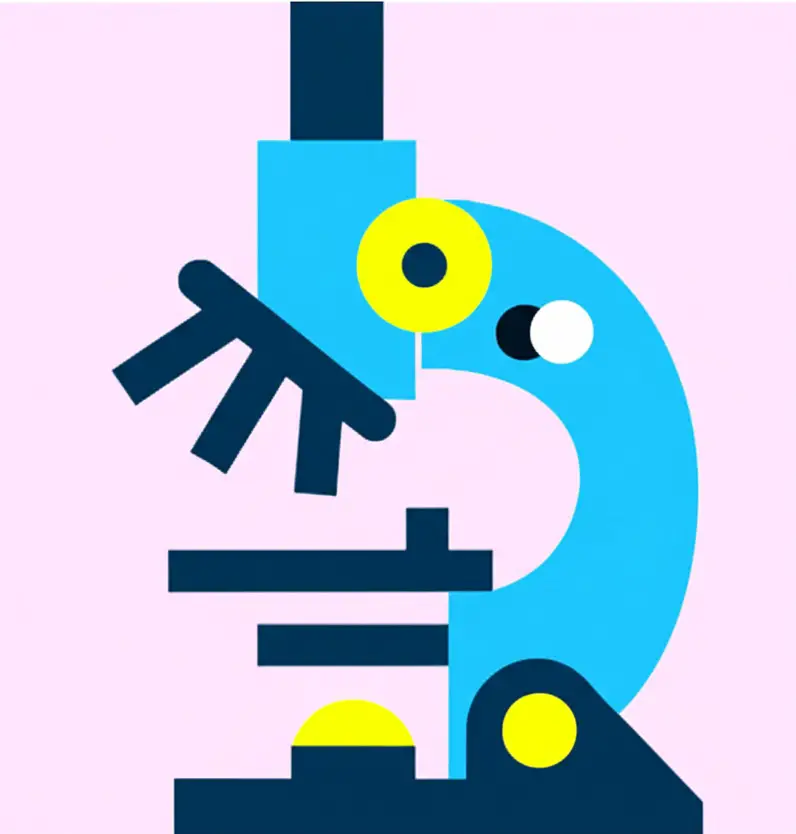
Sourav Pan
Transcript
The process of osmosis describes how water molecules move across semipermeable membranes.
A semipermeable membrane allows some molecules like water to pass through, but blocks others like solutes.
Let’s visualize this with water molecules in blue and solute particles in yellow.
During osmosis, water molecules move from areas of lower solute concentration to areas of higher solute concentration.
Water molecules can pass through the small pores in the membrane, while larger solute molecules remain on their original side.
This movement continues until equilibrium is reached, where the concentration of solutes is balanced on both sides, or until it’s actively prevented by cellular mechanisms.
In a hypotonic environment, the solution surrounding a cell has a lower solute concentration than inside the cell.
Water molecules from the hypotonic solution flow into the cell through the process called endosmosis.
This happens because cells typically have a higher solute concentration than their surroundings in a hypotonic environment.
As water continues to flow in, the cell swells and may eventually burst if the process continues unchecked.
Plant cells respond differently to hypotonic environments compared to animal cells due to their specialized structure.
The key difference is the presence of a rigid cell wall surrounding the cell membrane.
When a plant cell is placed in a hypotonic solution, water moves into the cell through osmosis.
As water continues to enter the cell through osmosis, it creates pressure as the cell membrane pushes against the rigid cell wall.
This internal hydrostatic pressure is called turgor pressure. It’s what gives plants their structural rigidity and support.
A plant cell in an isotonic solution may be flaccid, with the cell membrane partially pulled away from the cell wall.
But when placed in a hypotonic solution, water flows in, and the cell becomes turgid as the membrane pushes tightly against the cell wall.
Unlike animal cells, which can swell and potentially burst in hypotonic solutions, plant cells are protected by their rigid cell wall.
Turgor pressure provides essential structural support for plants, allowing them to maintain their upright position.
When plant cells lose water and become flaccid, plants wilt. When cells are turgid, the plant stands upright and firm.
To summarize, plant cells in hypotonic solutions absorb water through osmosis, creating turgor pressure as the cell membrane pushes against the cell wall. This provides essential structural support and prevents the cells from bursting.
Hypotonic environments play a crucial role in plant health and function.
When a plant cell is in a hypotonic environment, water molecules move into the cell through osmosis.
As water enters the cell, it creates turgor pressure, pushing outward against the rigid cell wall.
This turgor pressure is essential for maintaining plant rigidity and upright growth.
When plants cannot maintain adequate turgor pressure, such as in drought conditions or hypertonic environments, they begin to wilt.
Unlike animals with skeletal systems, plants rely entirely on turgor pressure to support their structures.
Hypotonic conditions are also crucial for nutrient uptake through plant roots.
Root cells create a hypotonic environment that draws water and dissolved nutrients from the soil into the plant.
Once absorbed by the roots, water and nutrients are transported throughout the plant through vascular tissues.
The continuous flow of water through plant tissues, made possible by hypotonic conditions, is essential for photosynthesis, nutrient distribution, and overall plant health.
Osmoregulation is the process by which animals maintain proper water and solute balance in their bodies.
This process is critical for survival as it prevents cell damage, maintains cellular function, and is essential in varying environments.
In hypotonic environments, where the surrounding water has fewer solutes than the animal’s cells, water constantly enters the cells through osmosis.
Single-celled organisms like protists have evolved specialized structures called contractile vacuoles to manage excess water.
Water enters the protist through osmosis because the cell has a higher solute concentration than its surroundings.
The contractile vacuole collects this excess water, gradually expanding as it fills.
When full, the vacuole contracts, forcefully expelling the water outside the cell. This cycle repeats continuously to maintain proper cell volume.
Freshwater fish face a similar challenge. Since their bodies contain more solutes than the surrounding water, water constantly enters through their gills and skin.
Water flows into the fish primarily through the thin membranes of the gills, which are designed for gas exchange but also allow water to enter through osmosis.
To counteract this constant influx of water, the fish’s kidneys play a crucial role in osmoregulation.
Unlike terrestrial animals, freshwater fish produce large volumes of very dilute urine. Their kidneys filter out salts to be reabsorbed while allowing excess water to be excreted.
Osmoregulation is an energy-intensive process. Both the contractile vacuoles in protists and the kidney functions in fish require cellular energy in the form of ATP.
In summary, osmoregulation is a vital process for animals living in hypotonic environments. Through specialized structures and mechanisms, animals maintain proper water balance despite the constant challenge of osmosis.
The kidneys play a crucial role in regulating the body’s osmotic balance through the formation of hypotonic urine.
This process occurs in specialized structures called nephrons, which are the functional units of the kidney.
Each nephron consists of several key components: the glomerulus, proximal tubule, loop of Henle, distal tubule, and collecting duct.
The process begins with filtration. Blood enters the nephron through the afferent arteriole and reaches the glomerulus.
In the proximal tubule and loop of Henle, selective reabsorption occurs. Essential solutes like sodium and water are reabsorbed back into the bloodstream.
In the loop of Henle and collecting duct, a concentration gradient allows for more water to be reabsorbed without solutes. This process creates hypotonic urine.
This selective reabsorption process results in urine that is hypotonic compared to blood. It contains fewer solutes relative to water.
This ability to create hypotonic urine is crucial for maintaining proper blood concentration, regulating fluid volume, and ensuring optimal cellular function.
Marine organisms face very different environmental challenges compared to freshwater species.
In freshwater environments, the surrounding water is hypotonic – meaning it has a lower salt concentration than the animal’s body fluids.
In contrast, marine environments are hypertonic – with a higher salt concentration than the organism’s internal fluids.
Marine fish have developed specialized adaptations to prevent dehydration in their salty environment.
Unlike freshwater fish, marine fish actively drink seawater to replace water lost through osmosis.
Their specialized kidneys filter this seawater, retaining water while removing excess salts.
They then excrete highly concentrated salt solutions through specialized cells in their gills and in their urine.
Marine birds and reptiles have evolved specialized salt glands as additional adaptation mechanisms.
Marine birds, like seagulls and albatrosses, have salt glands located above their eyes.
These glands extract excess salt from the bloodstream and secrete a highly concentrated salt solution that drips out through the nostrils.
Similarly, marine reptiles like sea turtles and marine iguanas have salt glands that excrete excess salt as ‘tears’.
These secretions can be remarkably concentrated – up to five times saltier than seawater itself.
These specialized adaptations in marine organisms provide critical survival advantages in salty environments.
First, they prevent dehydration by maintaining proper water balance despite living in a hypertonic environment.
Second, these adaptations conserve energy by efficiently regulating salt levels without expending excessive metabolic resources.
Finally, these adaptations have allowed species to expand into marine habitats, enabling them to thrive in oceans worldwide.
Cells have evolved sophisticated mechanisms to regulate their volume despite osmotic challenges from the environment.
In a hypotonic solution, water flows into the cell due to osmosis, causing it to swell.
If left unchecked, this swelling could lead to cell damage or even rupture.
To counteract swelling, cells employ a mechanism called Regulatory Volume Decrease, or RVD.
This involves activating specific ion channels in the cell membrane.
During RVD, cells release potassium and chloride ions to the outside environment.
As ions flow out, water follows due to osmosis, reducing cell volume back to normal.
For example, red blood cells use this regulatory volume decrease mechanism to prevent rupturing when exposed to hypotonic blood conditions.
Beyond RVD, cells use multiple mechanisms to regulate their volume, including specialized ion transporters, cytoskeletal rearrangement, and organic osmolytes.
These volume regulation mechanisms are crucial for cell survival and maintaining normal physiological function in changing osmotic environments.
Understanding hypotonic solutions has important applications in medicine and everyday life.
In medical settings, hypotonic IV solutions like half-normal saline and D5W are commonly used.
However, these solutions must be administered carefully, as they can cause cells to swell due to water moving into cells by osmosis.
Hypotonic eye drops present another medical application. When applied to the eye, they can cause corneal cells to swell.
This swelling occurs as water moves into corneal cells by osmosis, leading to blurred vision and discomfort.
Sports drinks are carefully formulated to prevent hypotonic conditions during intense exercise.
Unlike plain water, sports drinks contain electrolytes and carbohydrates that help maintain proper osmotic balance in the body.
Drinking only water during prolonged exercise can lead to hyponatremia, a potentially dangerous condition where sodium levels in the blood become too diluted.
Maintaining proper osmotic balance is critical for cellular health and function.
Healthcare providers must carefully consider fluid tonicity when treating patients, as improper use of hypotonic solutions can lead to serious complications.
Understanding hypotonic solutions is essential for medical safety and optimal health.
Study Materials
No study materials available for this video.
Related Videos
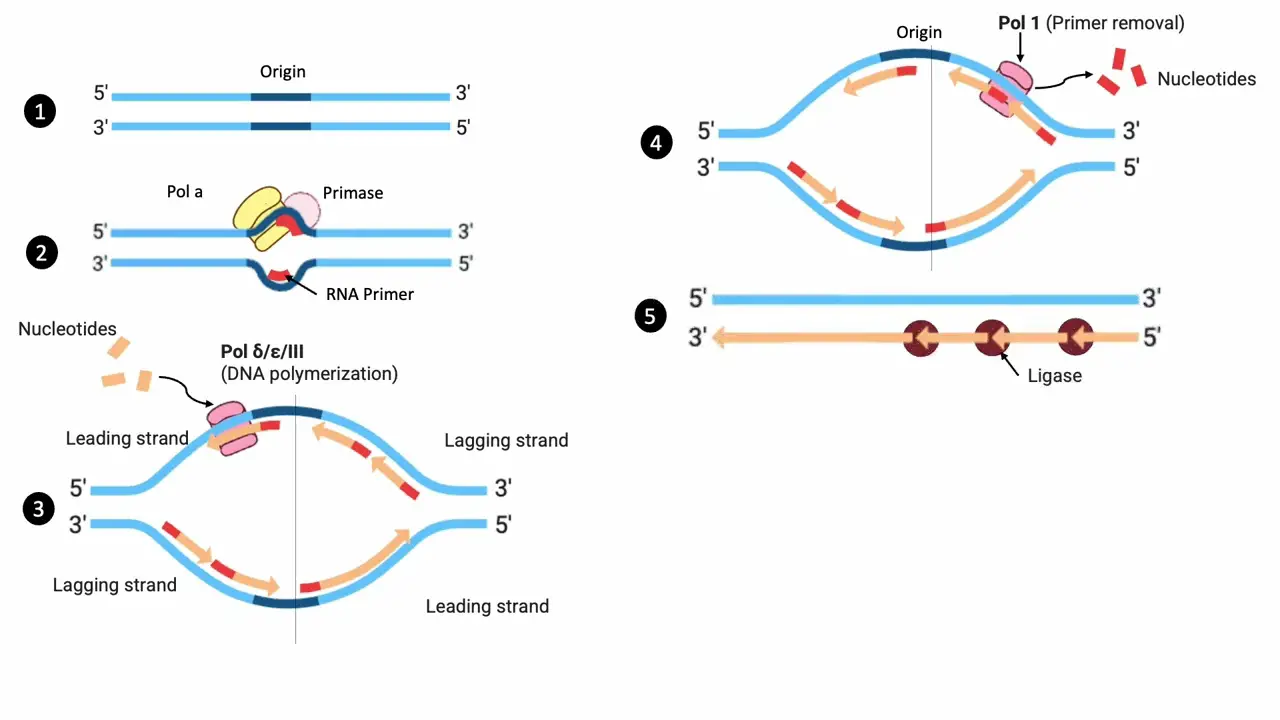
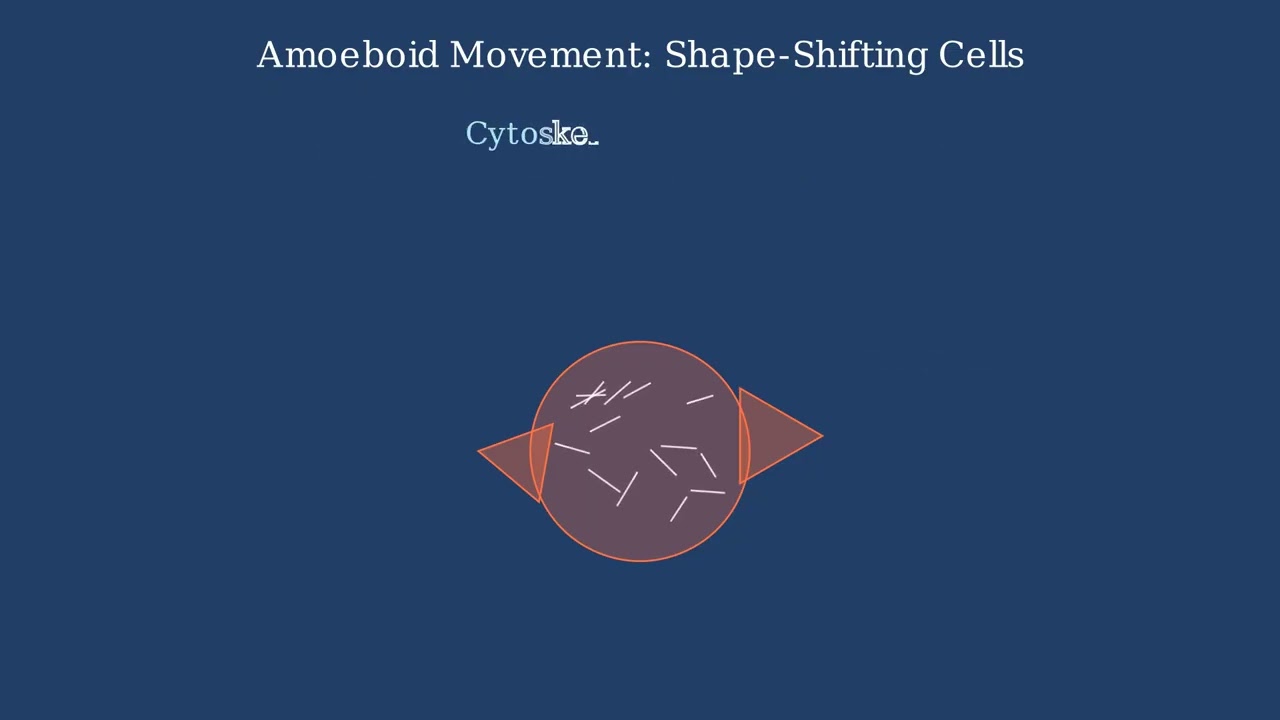
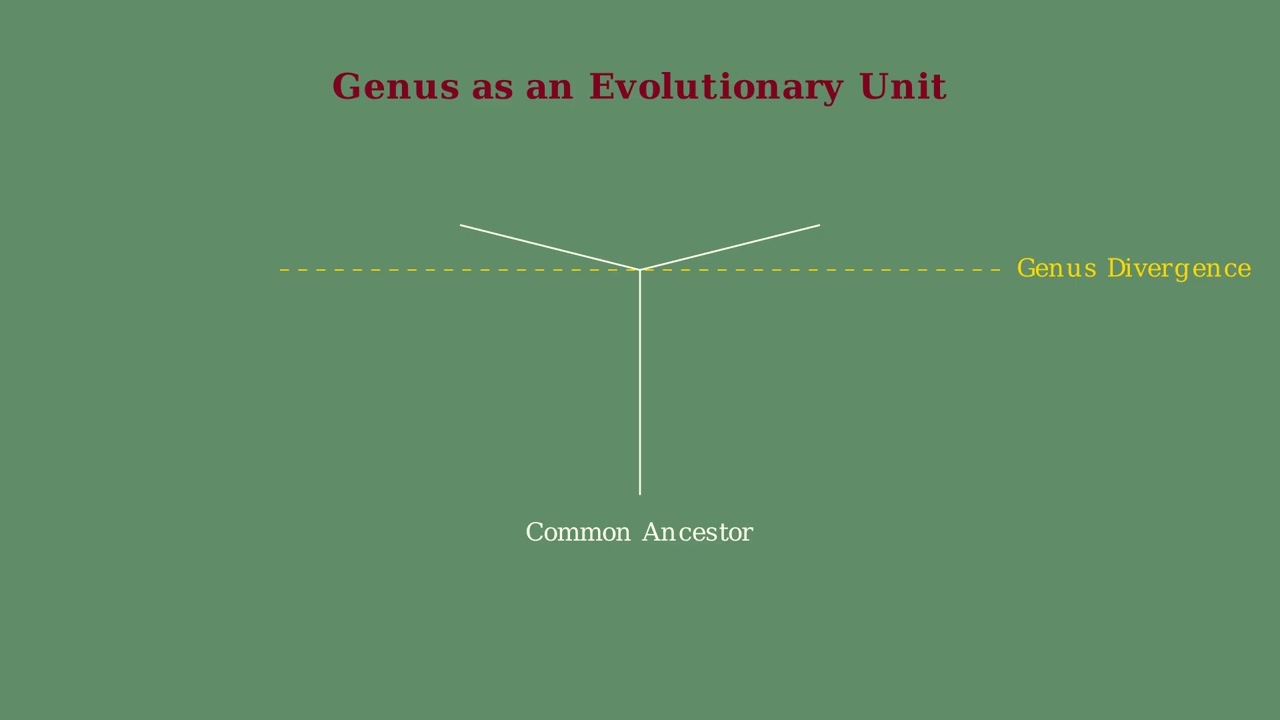
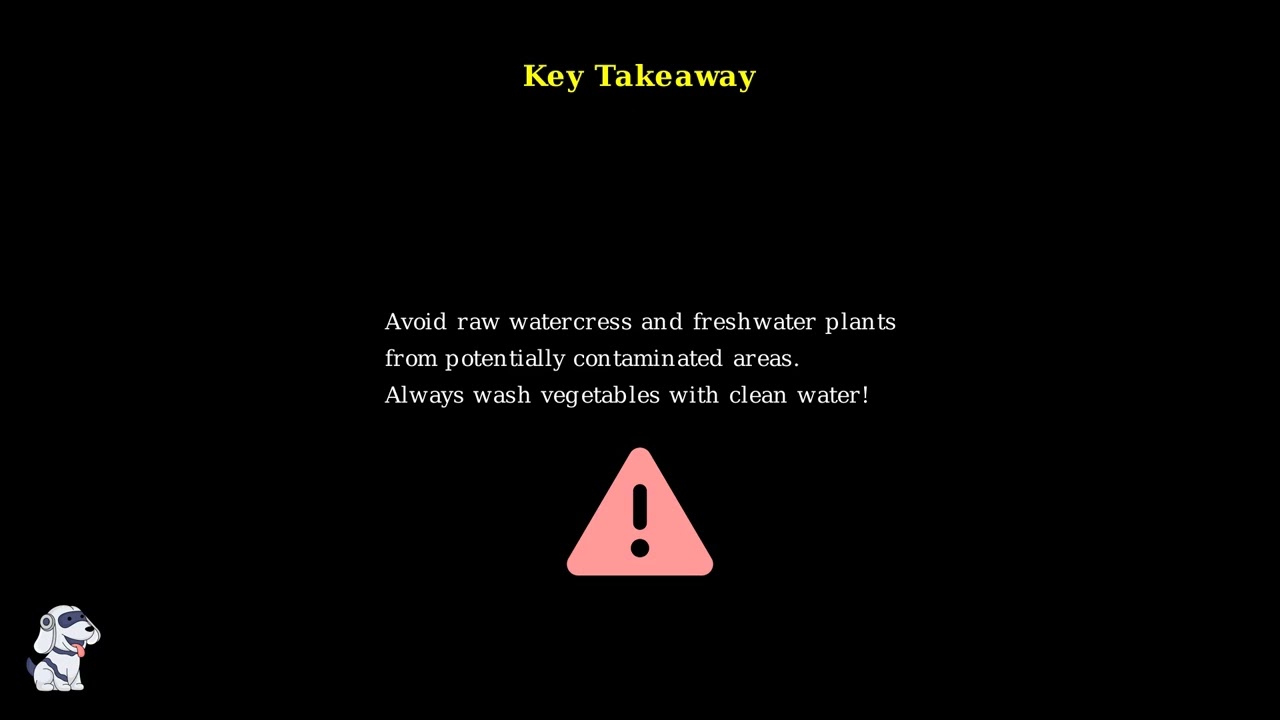
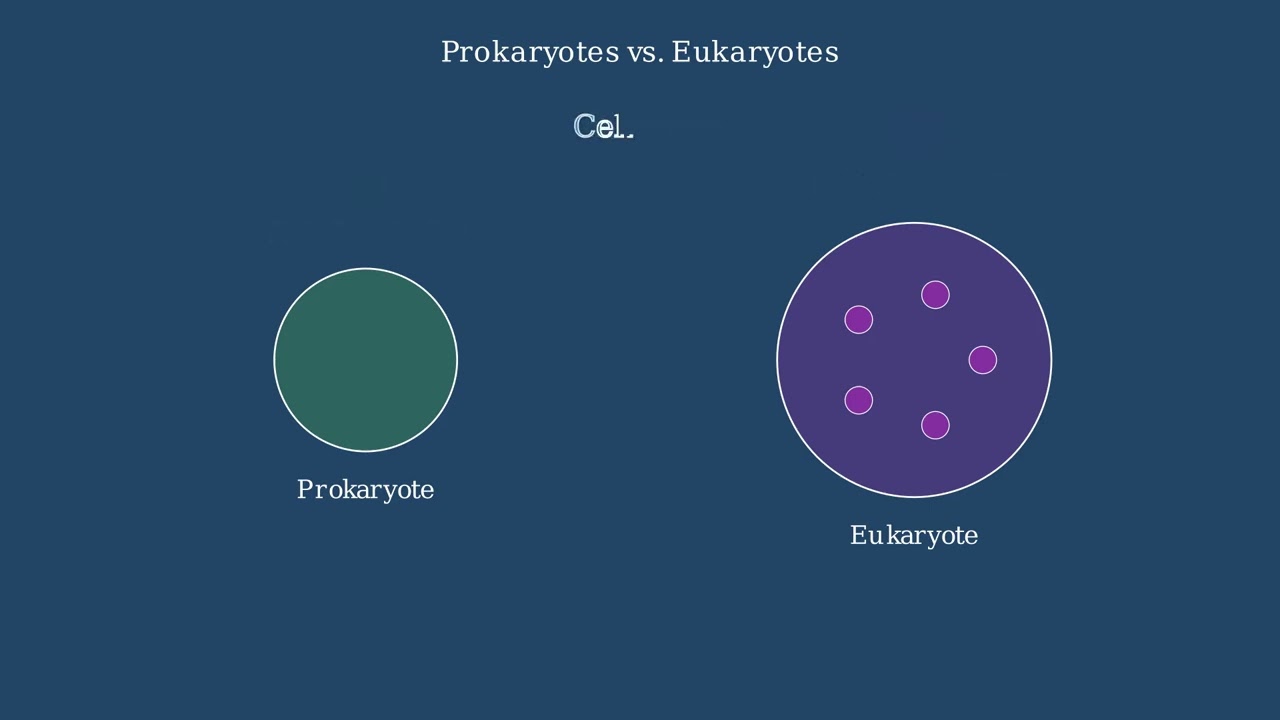
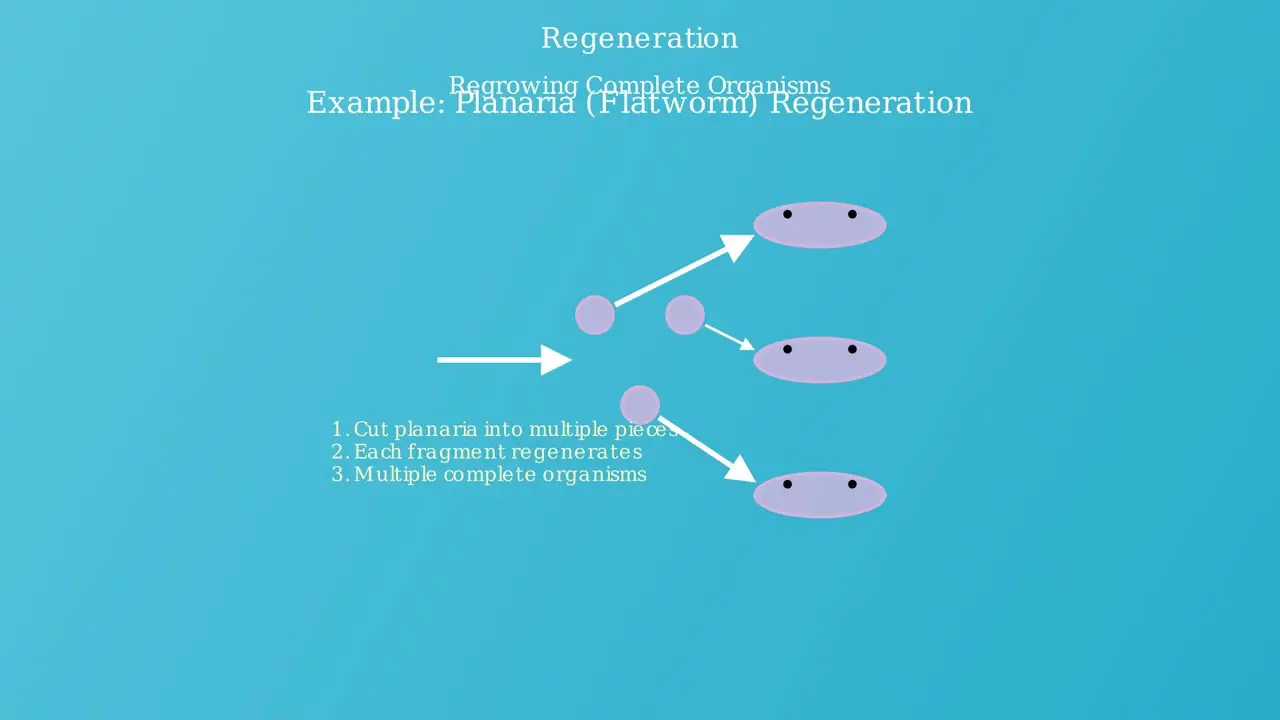
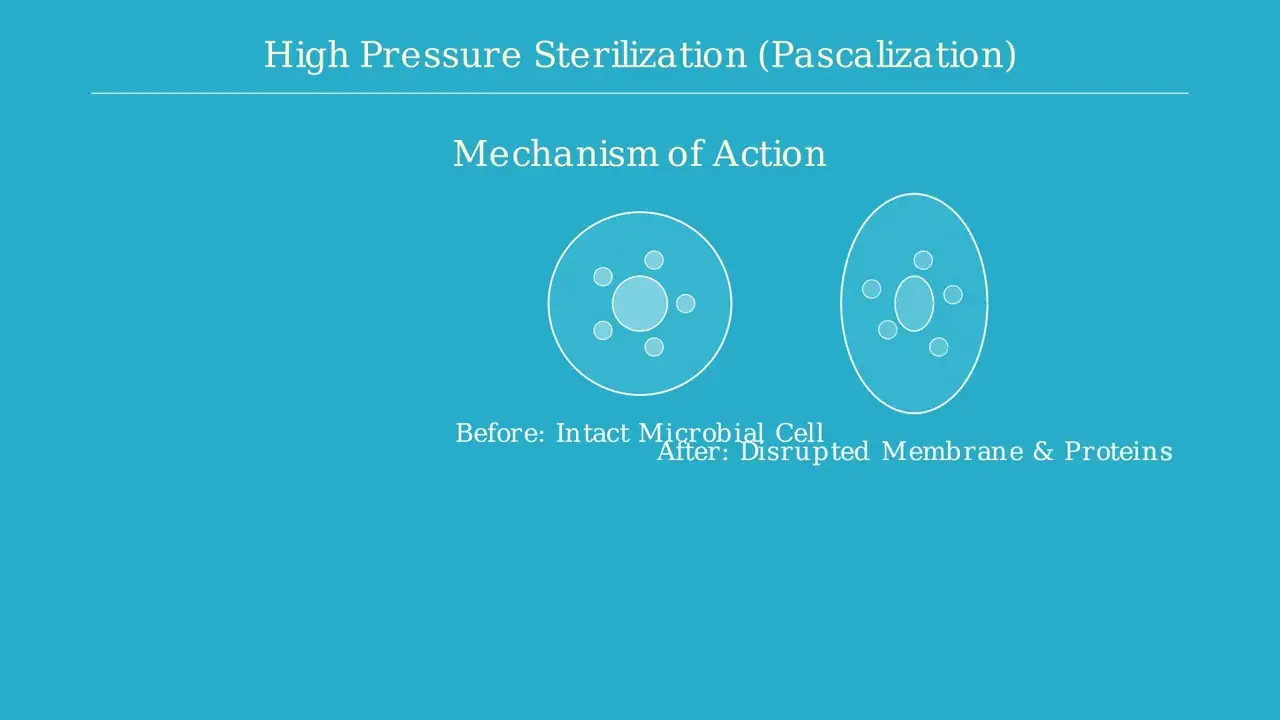
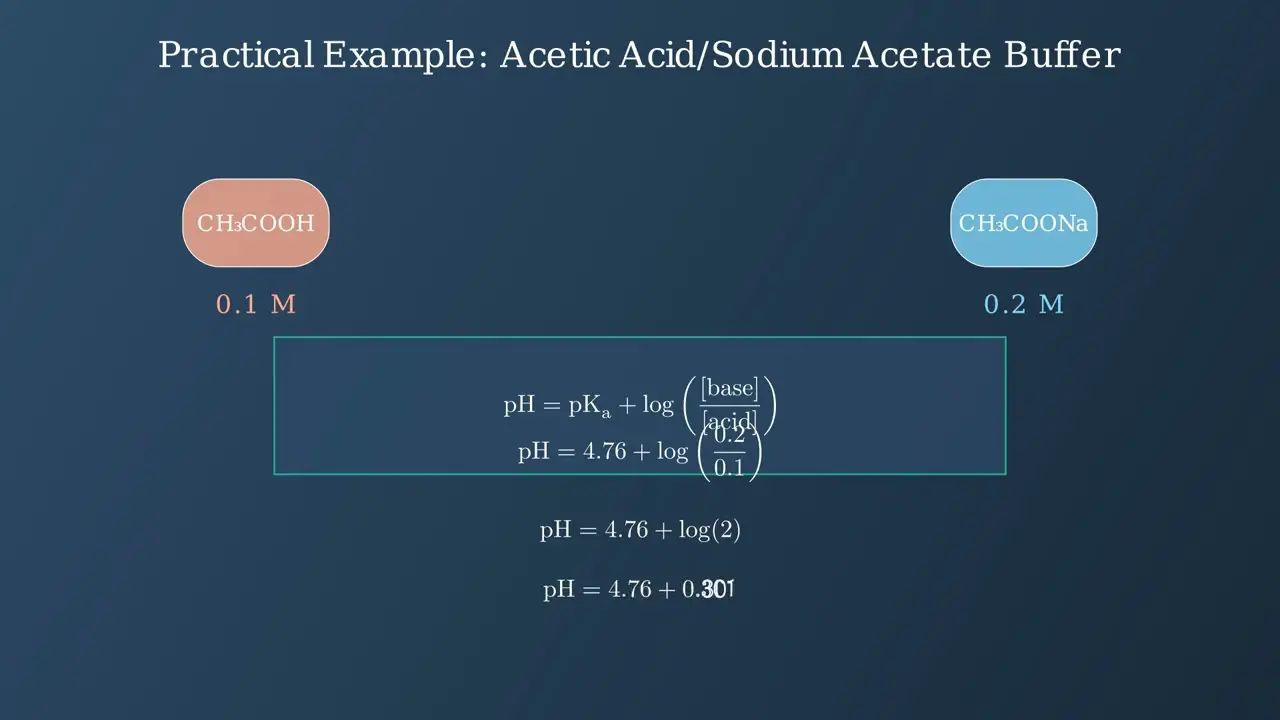
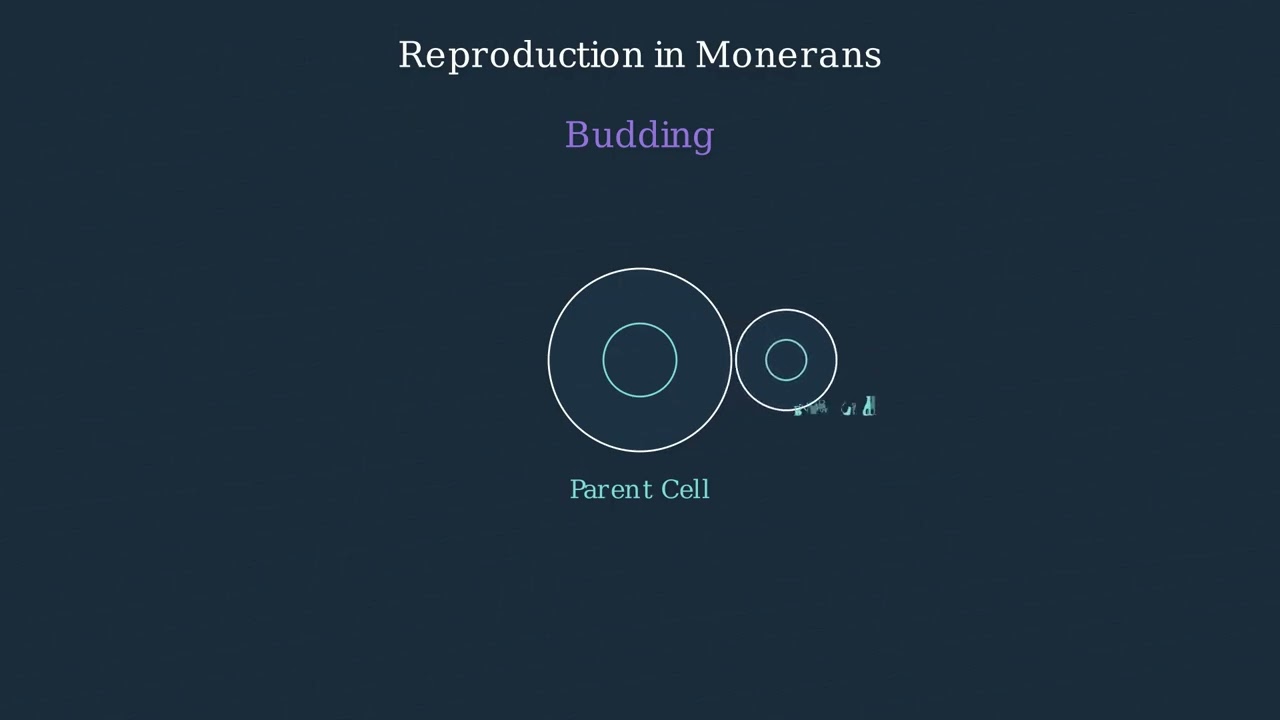