What are Transgenic Plants?
- A transgenic plant is a genetically modified organism in which genetic material from another species is introduced using techniques of genetic engineering. This process allows for the insertion of specific genes that confer desired traits, which may include increased yield, improved quality, resistance to pests, diseases, or environmental stresses such as drought or extreme temperatures.
- The development of transgenic plants typically involves sophisticated methods such as biolistics, where DNA-coated particles are propelled into plant cells, or Agrobacterium-mediated transformation, where a naturally occurring bacterium transfers DNA into the plant’s genome. These techniques enable scientists to introduce genes from unrelated organisms, altering the plant’s characteristics in ways that are not achievable through traditional breeding methods.
- Various crops have been successfully engineered as transgenic plants, including maize, rice, eggplant, cabbage, cauliflower, potato, and tomato. Each of these plants has been modified to express specific traits that enhance their agricultural value. For instance, crops like Bt-cotton incorporate genes from the bacterium Bacillus thuringiensis, which produce proteins toxic to certain insect pests, thereby reducing the need for chemical insecticides.
- One notable example of transgenic plant application is Golden Rice, which has been engineered to produce beta-carotene, a precursor of vitamin A. This modification aims to address nutritional deficiencies in regions where rice is a dietary staple.
- The primary goal of creating transgenic plants is to enhance agricultural productivity and sustainability. These plants offer several advantages, including increased resistance to pests and diseases, improved tolerance to environmental stresses, and enhanced nutritional content. Additionally, transgenic plants can serve as bioreactors for producing valuable proteins used in pharmaceuticals and industrial processes.
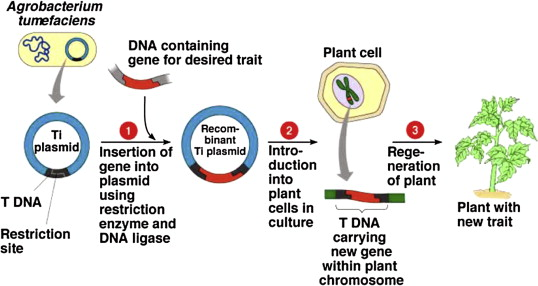
Definition of Transgenic Plants
Transgenic plants are genetically modified organisms in which DNA from another species is inserted into their genome through genetic engineering techniques to confer new traits, such as pest resistance or improved nutritional content.
Different Methods to Create Transgenic Plant
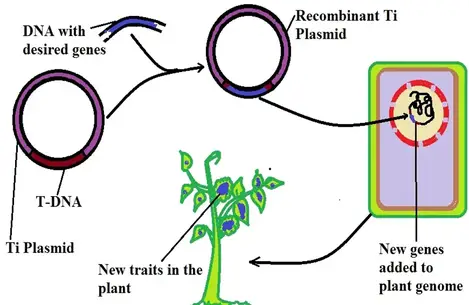
Creating transgenic plants involves various techniques to introduce the gene of interest into the plant’s genetic material. Here are some of the main methods used in this process:
- Agrobacterium-Mediated Transformation: This method uses the bacterium Agrobacterium tumefaciens, which naturally transfers DNA into plants. The bacterium’s Ti-plasmid (Tumor-inducing plasmid) is modified to carry the desired gene. When the bacterium infects the plant, the plasmid integrates the gene into the plant genome, sometimes resulting in crown gall disease, characterized by tumor-like growths.
- Biolistic Transformation (Gene Gun Method): This technique involves coating microscopic particles of gold or tungsten with the gene of interest. These particles are then shot into plant cells using a gene gun. The high-speed particles penetrate the cell walls and membranes, delivering the genetic material directly into the cell nucleus.
- Electroporation: In electroporation, plant cells are exposed to an electric field that creates temporary pores in the cell membrane. These pores allow the genetic material to enter the cell. Once inside, the genetic material can integrate into the plant’s DNA.
- Microinjection: This highly precise technique involves directly injecting foreign DNA into the plant cell nucleus using a fine needle. While effective, microinjection is technically challenging and requires specialized equipment and skills.
- Virus-Mediated Gene Transfer: Plant viruses can be engineered to carry the gene of interest. When these modified viruses infect plant cells, they introduce the foreign gene, which can then be expressed by the plant.
- Polyethylene Glycol (PEG) Transformation: PEG is a chemical that, when applied to plant cells, disrupts the cell wall, making it more permeable. This allows the foreign DNA to enter the cell and integrate into the plant’s genome.
- Whisker-Mediated Transformation: This method uses tiny needle-like structures made of tungsten, known as whiskers, which are coated with the gene of interest. These whiskers mechanically penetrate plant cells, delivering the genetic material.
- Liposome-Mediated Transformation: Liposomes are lipid vesicles that can encapsulate DNA. When these liposomes fuse with the plant cell membrane, they deliver the enclosed genetic material into the cell.
- Protoplast Transformation: Protoplasts are plant cells that have had their cell walls removed. These cells are more amenable to genetic manipulation and can be transformed using various methods such as electroporation, PEG, or microinjection.
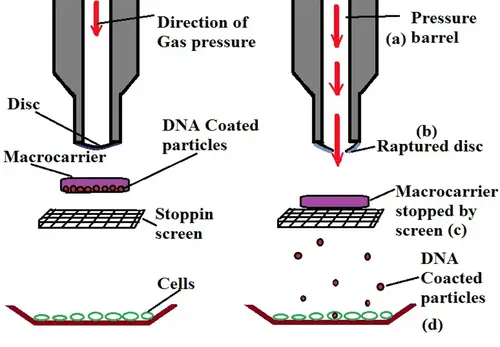
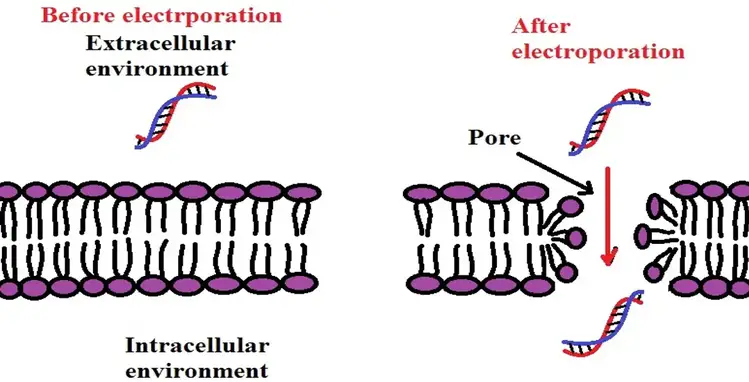
Components of a Gene Construct
- Promoter Region: The promoter is a DNA sequence located upstream of the gene. It plays a pivotal role in regulating gene expression by acting as an on/off switch. Promoters are recognized by transcription factors, which bind to them and recruit RNA polymerase to initiate transcription. Promoters can be classified into:
- Constitutive Promoters: Active throughout various developmental stages, maintaining a consistent level of gene expression.
- Tissue-Specific Promoters: Restricted to certain tissues or developmental stages.
- Inducible Promoters: Regulated by environmental stimuli, allowing gene expression to be controlled by external factors.
- Enhancers and Silencers: Enhancers are short DNA sequences that can significantly increase the transcription of a gene by binding to activator proteins. These proteins form a complex that interacts with the promoter to enhance its accessibility to transcription machinery. Conversely, silencers bind to repressor proteins, which downregulate gene transcription.
- Gene Coding Region: This region contains the sequence that codes for the protein of interest. It includes:
- Exons and Introns: Exons are coding sequences, while introns are noncoding regions that are removed during RNA splicing.
- Start Codon (ATG): Marks the beginning of translation.
- Stop Codon (TAA/TAG): Signals the end of translation.
- Terminator/Stop Region: Located downstream of the gene, the terminator sequence signals the end of transcription and includes a polyadenylation site (poly-A tail), which stabilizes the mRNA transcript.
Regulatory Elements in Gene Constructs
- Reporter Genes: These genes are used to monitor the expression and localization of the transgene. Common reporter genes include Green Fluorescent Protein (GFP), beta-glucuronidase (GUS), and luciferase (Luc). They help differentiate transformed cells from non-transformed ones and provide insights into gene regulation.
Issues and Solutions in Gene Constructs
- Antibiotic Resistance Genes: While effective for selecting transformed cells, these genes pose environmental and health risks. The transfer of antibiotic resistance genes to bacteria or neighboring plants can lead to increased antibiotic resistance. Alternatives to antibiotic markers include reporter genes like GFP, which do not have the same environmental impact.
- Advanced Genome Editing Tools: Technologies such as TALEN and CRISPR/Cas9 offer potential solutions for removing antibiotic resistance genes from transgenic plants. These tools enable precise editing of the genome, reducing the risks associated with gene escape.
Vectors for the production of transgenic plants
Components of Vectors
- Origin of Replication: Located on the vector, this region consists of AT-rich sequences that initiate replication. It binds to protein complexes, unwinds the DNA, and facilitates replication with the help of polymerases.
- Multicloning Site or Recombination Site: This region contains multiple unique sequences, also known as restriction sites, where specific restriction enzymes can cut the DNA. These sites allow for the insertion of genes of interest into the vector.
- Selectable Marker: Genetic markers that validate the successful insertion of the vector into the target organism. They aid in selecting cells that have taken up the vector during transformation.
Types of Vectors Used in Plant Transformation
1. Plasmid Vectors
1.1. Ti Plasmid
The Ti plasmid is the most commonly used vector for producing transgenic plants. It typically ranges in size from 200 to 800 kilobase pairs (kbp) and consists of several key regions:
- Transfer DNA (T-DNA) Region: Approximately 24 kbp in size, this region contains genes of interest and is bordered by repeat sequences known as left and right borders. The right border is essential for T-DNA transfer into plant cells.
- Virulence Region: Encodes vir genes that facilitate T-DNA transfer.
- Opine Catabolism Region: Contains genes for opine synthesis, which serves as a nutrient source for Agrobacterium tumefaciens.
The Ti plasmid induces crown gall disease in plants due to the presence of oncogenes like those involved in cytokinin and auxin biosynthesis.
1.2. Binary Vector System
To overcome limitations of large Ti plasmids, the binary vector system was developed. It consists of two plasmids:
- Mini Vector: Contains the T-DNA region and replication origins for both E. coli and A. tumefaciens.
- Helper Vector: A wild-type Ti plasmid without T-DNA, providing essential genes for T-DNA transfer and integration.
Both vectors are introduced into Agrobacterium together, facilitating efficient plant transformation.
1.3. Co-integrative Vector
This vector results from homologous recombination between an intermediate vector (usually an E. coli plasmid) containing the gene of interest and a disarmed Ti plasmid. It merges both plasmids into a larger co-integrative vector, which is subsequently used for plant transformation. However, handling large co-integrative vectors can be challenging.
2. Plant Virus Vectors
Plant viruses have been modified for use as vectors in plant transformation. Commonly used plant virus vectors include Cauliflower mosaic virus (CaMV), Tobacco mosaic virus (TMV), and Potato virus X (PVX).
- Advantages: Efficient delivery of genetic material due to their natural ability to infect plants.
- Modified Viral Vectors: ‘Deconstructed’ viruses where undesired genes (e.g., coat protein genes) are removed and replaced with genes of interest or selectable markers for transgenic screening.
Challenges and Future Directions
- Vector Size and Handling: Large plasmid sizes can complicate manipulation and maintenance in bacterial hosts. The development of smaller, efficient vectors continues to be an area of focus.
- Environmental and Regulatory Considerations: Concerns over gene flow and environmental impact necessitate careful selection and design of vectors, particularly regarding selectable markers.
- Emerging Technologies: Advances in genome editing tools, such as CRISPR/Cas9, offer promising alternatives to traditional vector-based approaches by enabling precise gene editing in plants.
Integration and inheritance of the transgenes
Integration of Transgenes
Transgenes are integrated into plant cells through methods that can result in stable or transient transformation, each affecting how the gene is inherited and expressed:
- Stable Transformation:
- In stable transformation, transgenes are introduced into the nucleus of plant cells and integrate into the plant genome.
- Integrated transgenes become part of the plant’s genetic material and are replicated along with the host DNA during cell division.
- This integration enables transgenes to be passed on to subsequent generations of plants, where they continue to express their traits.
- Methods such as Agrobacterium-mediated transformation and biolistic methods can achieve stable transformation.
- Transient Transformation:
- Transiently transformed plants express transgenes temporarily without integrating them into the genome.
- Transgenes in transient transformation are not replicated during cell division and are eventually lost over time.
- This method is useful for short-term gene expression studies and does not lead to heritable changes in subsequent generations.
- Techniques like Agrobacterium-mediated transformation with non-integrated T-DNA and direct gene transfer methods often result in transient transformation.
Inheritance of Transgenes
Understanding how transgenes are inherited involves considerations of both Mendelian and non-Mendelian genetics:
- Mendelian Inheritance:
- According to Mendel’s laws, genes segregate and assort independently during gamete formation.
- Traits controlled by transgenes can follow Mendelian patterns if the integration and expression do not disrupt normal genetic principles.
- Non-Mendelian Inheritance:
- Transgene inheritance may deviate from Mendelian patterns due to various factors:
- Location of Integration: Position effects can influence transgene expression and inheritance.
- Copy Number: Multiple copies or rearrangements of transgenes can affect inheritance patterns.
- Epigenetic Factors: Transgene silencing or activation can alter inheritance outcomes.
- Genetic Interactions: Interactions between transgenes and recipient genome factors can lead to non-Mendelian inheritance.
- Examples of non-Mendelian patterns include incomplete dominance, codominance, and interactions with multiple alleles or polygenic traits.
- Transgene inheritance may deviate from Mendelian patterns due to various factors:
Analysis and confirmation of transgene integration
To verify the successful integration of transgenes into plant genomes, several methods are employed, each tailored to the specific characteristics of the transgene constructs and the desired outcomes:
1. Selection Marker and Reporter Gene Screening
Transgenic plant cells are initially screened using selectable markers and reporter genes:
- Selectable Marker Screening:
- Antibiotic resistance or herbicide tolerance genes are incorporated into transgene constructs.
- Plant cells expressing these markers survive in media containing antibiotics or herbicides, distinguishing them from non-transformed cells.
- Drawbacks include high costs and risks of environmental contamination through horizontal gene transfer.
- Reporter Gene Expression Screening:
- Reporter genes such as GFP, GUS, and Luc produce detectable signals (fluorescent, colorimetric, or luminescent) when expressed.
- Visual observation or microscopic examination confirms gene expression.
- Quantification using spectrophotometry measures the level of reporter gene expression.
- Techniques like histochemical assays reveal spatial expression patterns, enhancing localization studies.
- Enzyme assays, such as CAT and LacZ activity tests, provide additional confirmation through enzymatic reactions.
2. Southern Blotting
Southern blotting is a molecular technique used for detailed analysis of transgene integration:
- Principle:
- DNA samples are digested with restriction enzymes, separating them by size through gel electrophoresis.
- Fragments are transferred onto a membrane (nitrocellulose or nylon) and probed with labeled DNA sequences specific to the transgene.
- Autoradiography or chromogenic detection visualizes hybridization patterns.
- Applications:
- Determines transgene copy number and integrity.
- Identifies transgene rearrangements within the host genome.
3. Polymerase Chain Reaction (PCR)
PCR is a versatile and sensitive method for detecting specific DNA sequences:
- Procedure:
- Uses gene-specific primers to amplify DNA fragments corresponding to transgene sequences.
- Successful amplification indicates presence of the transgene.
- Confirmation through DNA sequencing ensures accuracy.
- Real-Time PCR (qPCR):
- Provides quantitative and qualitative analysis in real-time.
- High-throughput capability enhances efficiency compared to traditional methods like Southern blotting.
- Useful for determining transgene copy number and zygosity in transgenic plants.
4. Next-Generation Sequencing (NGS)
NGS technologies offer advanced capabilities for comprehensive transgene analysis:
- Advantages:
- Enables rapid, cost-effective sequencing of entire genomes.
- Facilitates identification of precise transgene insertion sites, even in complex genomes with repetitive sequences.
- Provides insights into transgene integrity, orientation, and interactions with host genomic elements.
- Applications:
- Overcomes limitations of PCR-based methods, such as non-specific amplification and inability to detect large or complex transgenes.
- Enhances molecular characterization and regulatory compliance in genetically modified crops.
Applications/Importance of Transgenic Plants
Transgenic plants play a crucial role in advancing sustainable agriculture, providing numerous benefits that address various agricultural challenges. Here are some key aspects highlighting the importance of transgenic plants:
- Pest and Disease Resistance: One of the primary advantages of transgenic plants is their enhanced resistance to pests and diseases. By incorporating genes that produce natural insecticides or confer resistance to specific pathogens, these plants can significantly reduce crop losses due to infestations and infections. This, in turn, reduces the reliance on chemical pesticides, promoting a healthier environment and safer food products.
- Herbicide Tolerance: Transgenic plants can be engineered to tolerate specific herbicides, allowing farmers to control weeds more effectively without harming the crops. This trait simplifies weed management, reduces the need for tillage, and can lead to higher crop yields by minimizing competition for resources.
- Increased Yield: To meet the growing global demand for food, crops have been genetically modified to increase their yield potential. By enhancing traits such as growth rate, nutrient uptake, and resistance to environmental stresses, transgenic plants can produce more food per unit of land, contributing to food security and agricultural productivity.
- Nutritional Enhancement: In regions where malnutrition is prevalent, transgenic plants can be engineered to provide enhanced nutritional content. For example, Golden Rice is fortified with beta-carotene, a precursor of vitamin A, to combat vitamin A deficiency in developing countries. Such biofortified crops can help address micronutrient deficiencies and improve public health.
- Abiotic Stress Tolerance: Transgenic plants can be designed to withstand various abiotic stresses, such as drought, salinity, and extreme temperatures. By incorporating genes that enhance stress tolerance, these plants can maintain productivity under adverse environmental conditions, ensuring stable food supplies despite climate variability.
- Improved Shelf Life: Some transgenic crops are modified to delay ripening and reduce spoilage, thereby extending their shelf life. This can be particularly beneficial for fruits and vegetables, which are prone to rapid deterioration. Improved shelf life reduces post-harvest losses and ensures that consumers have access to fresh produce for longer periods.
Advantages of Transgenic Plants
Transgenic plants offer several advantages that significantly contribute to agricultural efficiency, environmental sustainability, and food security. Here are some key benefits:
- Pest and Disease Resistance: Transgenic plants can be engineered to resist pests and diseases, reducing the need for chemical pesticides. This not only lowers production costs but also minimizes environmental pollution and enhances crop health.
- Herbicide Tolerance: These plants can be modified to withstand specific herbicides, allowing for more effective weed control. This results in better crop yields and reduces the need for multiple herbicide applications, which can be harmful to the environment.
- Increased Crop Yield: Genetic modifications can enhance the growth rate, nutrient absorption, and overall productivity of crops. This is essential for meeting the food demands of a growing global population.
- Enhanced Nutritional Value: Transgenic plants can be fortified with vitamins, minerals, and other nutrients to combat malnutrition, particularly in developing countries. For instance, Golden Rice is engineered to contain beta-carotene, a precursor of vitamin A, to address vitamin A deficiency.
- Tolerance to Abiotic Stresses: These plants can be designed to endure harsh environmental conditions such as drought, salinity, and extreme temperatures. This ensures stable crop production even in adverse climates, contributing to food security.
- Reduced Post-Harvest Losses: Some transgenic crops are modified to have a longer shelf life by delaying ripening and reducing spoilage. This leads to less food waste and ensures a more consistent supply of fresh produce.
- Environmental Benefits: By reducing the need for chemical pesticides and herbicides, transgenic plants help decrease the environmental impact of agriculture. This promotes biodiversity and reduces the contamination of soil and water bodies.
- Economic Benefits: Farmers can benefit from lower input costs (less need for pesticides and herbicides), increased yields, and potentially higher market prices for nutritionally enhanced or environmentally friendly crops.
- Production of Pharmaceuticals: Transgenic plants can be used as biofactories to produce pharmaceuticals, vaccines, and other valuable compounds. This biopharming approach can be more cost-effective and scalable than traditional methods.
- Improved Quality and Taste: Genetic modifications can enhance the flavor, texture, and appearance of crops, making them more appealing to consumers and potentially increasing market demand.
Limitations of Transgenic Plants
- Environmental Concerns: There is ongoing debate about the potential environmental impact of transgenic plants. Concerns include the possibility of gene flow to wild relatives, which could lead to the creation of “superweeds” resistant to herbicides or pests, potentially disrupting ecosystems.
- Resistance Development: Over time, pests and weeds may develop resistance to the traits conferred by transgenic plants. For example, insects might evolve resistance to Bt toxin, or weeds might become tolerant to glyphosate, necessitating the development of new transgenic traits or pest management strategies.
- Biodiversity Reduction: The widespread adoption of transgenic crops can lead to a reduction in agricultural biodiversity. Farmers may favor a few high-yielding transgenic varieties, which could displace traditional varieties and reduce the genetic diversity needed to adapt to changing environmental conditions.
- Ethical and Social Issues: The use of transgenic plants raises ethical questions about the manipulation of genetic material. Some people have concerns about the unnatural alteration of organisms and the long-term consequences of such changes. There are also socio-economic issues related to the control of seed patents by large biotechnology companies, which can impact smallholder farmers’ rights and access to seeds.
- Food Safety and Health Concerns: While transgenic plants undergo rigorous safety testing, some consumers remain skeptical about their potential health effects. Concerns include possible allergenicity and the long-term health impacts of consuming genetically modified foods, even though current evidence does not support these fears.
- Regulatory and Approval Processes: Developing and bringing transgenic plants to market involves complex, lengthy, and costly regulatory approval processes. These regulations vary by country and can create significant barriers to the adoption and commercialization of transgenic crops.
- Public Perception and Acceptance: Public opinion on genetically modified organisms (GMOs) is divided. Misinformation and lack of understanding about genetic engineering can lead to resistance against transgenic plants, affecting their acceptance and marketability.
- Economic Barriers: The development and commercialization of transgenic plants require substantial investment. This high cost can limit the availability of transgenic technologies to larger, wealthier farms and companies, potentially widening the gap between large-scale and small-scale farmers.
- Intellectual Property Issues: The patenting of genetically modified seeds by biotech companies can lead to legal and financial challenges for farmers, especially regarding seed saving and replanting. This can affect farmers’ autonomy and traditional agricultural practices.
- Potential Unintended Effects: Genetic modifications can sometimes result in unintended changes to the plant’s metabolism, which might affect the plant’s growth, development, or interaction with the environment in unforeseen ways.
Examples of Transgenic Plants
Transgenic plants are genetically engineered to enhance agricultural sustainability by incorporating specific traits that improve their productivity and resilience. Here are some notable examples:
- Bt Cotton: This transgenic plant contains a gene from the bacterium Bacillus thuringiensis (Bt), which produces a toxin harmful to certain insect pests. By incorporating the Bt gene, cotton plants gain resistance to pest attacks, reducing the need for chemical insecticides.
- Golden Rice: Engineered to address vitamin A deficiency in developing countries, Golden Rice has been modified to produce beta-carotene, a precursor of vitamin A. The beta-carotene gene is introduced into the rice genome, allowing the plant to synthesize this vital nutrient.
- Roundup Ready Soybeans: These soybeans are genetically altered to withstand glyphosate, a common herbicide. The modification allows farmers to use glyphosate to control weeds without harming the soybean plants, thereby improving crop management and yield.
- Flavr Savr Tomato: The Flavr Savr tomato is engineered to delay ripening by suppressing the ethylene gene responsible for this process. This genetic alteration extends the tomato’s shelf life and reduces spoilage during transportation and storage.
- Bt Corn: Similar to Bt cotton, Bt corn contains the Bt toxin gene from Bacillus thuringiensis. This modification grants the corn resistance to specific insect pests, enhancing crop yield and reducing the reliance on chemical pest control methods.
- Rainbow Papaya: Developed to combat the devastating ringspot virus, Rainbow Papaya includes a gene from the virus itself. This genetic modification enables the papaya plants to resist infection, thus safeguarding the crop and ensuring stable production.
References
- de Framond AJ, Bevan MW, Barton KA, Flavell R, Chilton MD. Mini-Ti plasmid and a chimeric gene construct: New approaches to plant gene vector construction. In: Downey K, Voellmy RW, Ahmad F, editors. Advances in Gene Technology: Molecular Genetics of Plants and Animals. Miami Winter Symp. 20th ed. Florida: Elsevier; 1983. pp. 159-170
- Jhansi Rani S, Usha R. Transgenic plants: Types, benefits, public concerns and future. Journal of Pharmacy Research. Aug 2013;6(8):879-883
- Hammond-Kosack K, Jones JD. Responses to plant pathogens. Biochemistry and Molecular Biology of Plants. Jan 2000;1(1):1102-1156
- Lai KS, Yusoff K, Mahmood M. Heterologous expression of hemagglutinin-neuraminidase protein from Newcastle disease virus strain AF2240 in Centella asiatica. Acta Biologica Cracoviensia/Series Botanica. Jan 2012;54(1):142-147
- Mithöfer A, Boland W. Plant defense against herbivores: Chemical aspects. Annual Review of Plant Biology. Jun 2012;63(1):431-450
- Howe GA, Jander G. Plant immunity to insect herbivores. Annual Review of Plant Biology. Jun 2008;59(1):41-66