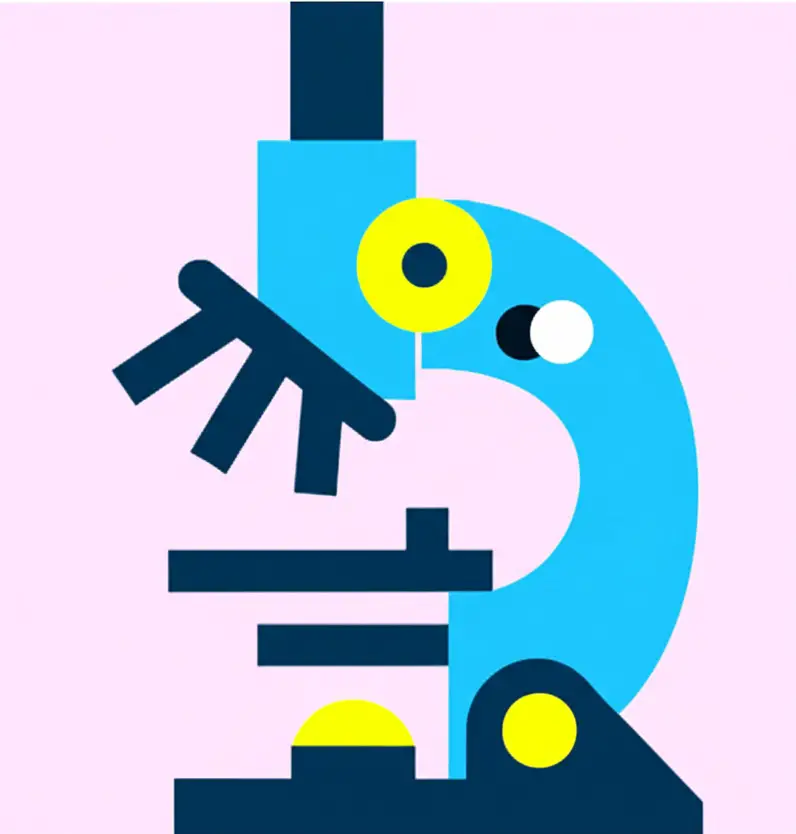
Sourav Pan
Transcript
Genus in the Taxonomic Hierarchy
Taxonomy provides a hierarchical classification system for all living organisms.
The genus level occupies a critical position in this hierarchy, sitting between family and species.
A genus groups closely related species that share specific derived characteristics from a common ancestor.
For example, humans belong to the genus Homo in the taxonomic hierarchy.
The genus level is important for several reasons. It organizes closely related species into manageable groups. It indicates evolutionary relationships between species. And it provides a practical unit for scientific classification and communication.
As we can see, the genus level serves as a crucial intermediate classification between the broader family level and the more specific species level.
Binomial nomenclature is the formal system of naming species of living things.
This system was established by Carl Linnaeus, a Swedish botanist, in the 18th century.
The scientific name in binomial nomenclature consists of two parts.
The first part is the genus, followed by the species.
There are specific rules for writing scientific names.
The genus is always capitalized, while the species is lowercase. Both are written in italics, and together they form the complete scientific name.
Let’s look at some examples of binomial nomenclature.
Panthera leo is the scientific name for the lion.
Homo sapiens is the scientific name for humans.
Quercus alba is the scientific name for the white oak tree.
A single genus can contain multiple related species. For example, the genus Panthera includes several big cat species.
These include Panthera leo, the lion; Panthera tigris, the tiger; Panthera onca, the jaguar; and Panthera pardus, the leopard.
Binomial nomenclature provides scientists with a universal way to identify organisms precisely, transcending language barriers and cultural differences.
Genus naming conventions follow strict rules in scientific taxonomy.
The first rule is that genus names are always capitalized, regardless of where they appear in a sentence.
Second, genus names must be italicized when printed in scientific literature, or underlined when written by hand.
Third, genus names are typically derived from Latin or Greek roots, often describing a characteristic feature of the organisms.
These naming conventions create consistency across the taxonomic system, helping scientists communicate accurately about organisms.
Let’s look at some examples of properly formatted genus names. Notice how each is capitalized and would be italicized in scientific publications.
To date, scientists have documented approximately five hundred and ten thousand genus names across all living organisms, from bacteria to plants and animals.
Exploring well-known examples of genera across different kingdoms of life.
Genera can be found across all major kingdoms of life, including animals, plants, fungi, and bacteria.
These well-known genera illustrate how taxonomists group organisms with shared characteristics into the same genus category.
The role of genus in species identification is fundamental to biological classification.
When identifying an unknown organism, taxonomists navigate through the hierarchical classification system.
The genus level is particularly crucial for identification. It represents a collection of closely related species that share distinctive characteristics.
A single genus can contain numerous species. For example, the oak genus, Quercus, includes approximately six hundred species worldwide.
Let’s look at a practical example of how genus characteristics help identify an unknown species.
The oak genus, Quercus, has several key characteristics. These include woody growth form, spiral leaf arrangement, and their distinctive acorn fruits.
Knowing the genus dramatically streamlines species identification.
With genus knowledge, you can quickly narrow your search to only oak species, focusing on specific traits that differentiate them.
Without genus knowledge, you would need to compare your specimen against hundreds of thousands of flowering plant species, making identification extremely challenging.
Let’s summarize why genus-level knowledge is so important for species identification.
Genus knowledge narrows the potential species pool dramatically, provides distinctive characteristics to look for, creates a manageable identification pathway, and serves as the foundation for taxonomic keys.
Genus and Evolutionary Relationships
Genus classification serves as a window into evolutionary history, revealing the shared ancestry of closely related species.
Evolutionary trees depict the relationships between species. Organisms placed within the same genus share a recent common ancestor.
For example, the genus Panthera includes lions, tigers, and jaguars, while the genus Felis includes domestic cats, wildcats, and sand cats.
Species within the same genus typically share important traits due to their shared evolutionary history.
These shared traits can be morphological, like body structures; ecological, such as habitat preferences; or behavioral, including social organization patterns.
Let’s look at a specific example: the genus Canis, which includes wolves, dogs, and coyotes.
While these species have evolved different adaptations, they share numerous traits that reflect their common ancestry.
Members of the Canis genus share similar skull structures, specialized carnassial teeth, pack hunting behaviors, complex social hierarchies, and similar vocalization patterns.
Genus classification provides valuable insights into evolutionary history, allowing scientists to predict traits and behaviors based on phylogenetic relationships.
This understanding helps researchers in fields such as comparative genomics, conservation biology, and the development of evolutionary models.
Genus classification plays a crucial role in modern agriculture, guiding practices from crop development to pest management.
Looking at important crop genera, we find Solanum, which includes potatoes, tomatoes, and eggplants.
Similarly, the Brassica genus includes cabbage, broccoli, turnips, and canola. These related species often share cultivation requirements.
Genus-based crop development leverages the genetic similarities within a genus. For example, the Triticum genus includes bread wheat, durum wheat, and spelt.
These related species share important agricultural traits like growing conditions, disease resistance profiles, and milling properties, which guides breeding programs.
Genus-specific pest management takes advantage of common susceptibility patterns within a genus.
For example, in the Citrus genus, we see shared patterns of susceptibility to diseases like citrus canker and citrus greening.
Genus classification guides several practical agricultural applications.
In crop rotation planning, farmers avoid planting crops from the same genus in succession to prevent disease buildup.
Breeding programs often transfer beneficial traits between species within a genus, as they share compatible genetics.
And pest management strategies are developed at the genus level, targeting common vulnerabilities shared among related species.
Understanding genus relationships helps farmers optimize yields while reducing pesticide use and breeding time.
Genus classification plays a critical role in medical and pharmaceutical research, providing essential frameworks for scientific advancement.
This taxonomic level is particularly valuable for three key applications: predicting bioactive compounds, understanding pathogens, and developing targeted treatments.
In drug discovery, researchers leverage the predictive power of genus-level relationships. When a species produces valuable compounds, related species in the same genus often yield similar molecules.
Several genera have profound importance in medicine. The genus Penicillium gave us penicillin antibiotics, while Streptomyces produces numerous antibacterial compounds including streptomycin.
Plasmodium species cause different forms of malaria. Plants like Taxus provide cancer treatments, while Digitalis contains cardiac glycosides used in heart medications.
The genus Taxus, or yew trees, demonstrates how related species often produce similar bioactive compounds. Both the Pacific and European yew contain taxanes with powerful anticancer properties.
Similarly, pathogens within the same genus often cause related diseases. Different Plasmodium species all cause malaria, though with varying severity and characteristics.
Genus classification enables targeted bioprospecting in new species, helps predict disease mechanisms, and guides the development of treatments. This taxonomic level continues to be essential for streamlining pharmaceutical research and improving medical interventions.
Unlike some scientific classifications that have clear, objective boundaries, genus definitions can be surprisingly subjective.
Different taxonomists may draw genus boundaries differently based on which characteristics they consider most important.
For example, Taxonomist A might split organisms into two separate genera based on distinctive physical features, while Taxonomist B might group them into a single genus based on genetic similarities.
These disagreements arise because taxonomists may prioritize different characteristics when defining genera.
We can see this subjectivity in action through several well-known examples of taxonomic disagreements.
These subjective decisions reflect the fundamental challenge of imposing discrete categories on the continuous process of evolution.
Overlapping characteristics between genera present one of the most significant challenges in taxonomy.
In an ideal taxonomic system, each genus would have clearly defined, unique characteristics that separate it from all others. However, nature rarely conforms to such neat categories.
Taxonomists often face situations where closely related genera share many characteristics, making classification challenging.
Genera often share several characteristics while maintaining a few unique traits. The overlapping characteristics create ‘blurry boundaries’ between closely related genera.
Let’s examine a specific case study where the boundaries between genera are particularly challenging.
Pine trees (Pinus) and Fir trees (Abies) are two conifer genera that share many characteristics and can be difficult to distinguish.
Both pines and firs are evergreen cone-bearing trees with similar geographical ranges.
To distinguish them, taxonomists must examine specific traits like needle arrangement, cone orientation, and cone persistence.
Similarly, the butterfly genera Vanessa and Polygonia share numerous traits that make classification challenging.
Despite DNA evidence suggesting they should be separate genera, these butterflies share wing patterns, feeding behaviors, and larval host plants, creating significant classification challenges.
To address these boundary issues, taxonomists employ multi-trait analysis.
This approach integrates multiple lines of evidence including morphology, DNA sequences, ecological niches, behavior, biochemistry, development patterns, and geographical distribution.
By examining multiple traits, taxonomists can better resolve the blurry boundaries between genera, though some cases remain challenging and subject to ongoing research and debate.
For many organisms, especially those newly discovered or poorly studied, classification at the genus level can be challenging due to insufficient information.
While higher taxonomic levels like kingdom, phylum, order, and family might be determined with confidence, genus assignment often remains uncertain for newly discovered organisms.
Several factors contribute to these information gaps. Often, scientists have access to only morphological data without genetic information. Sometimes only a single specimen is available, or key diagnostic features might be missing. For extinct organisms, no living specimens can be studied at all.
With incomplete information, taxonomists have several approaches. They may assign an organism to genus incertae sedis, indicating uncertain placement. They might use provisional qualifiers like ‘cf.’ or ‘aff.’ before genus names. Sometimes they place the organism in the most closely related known genus as a conservative approach. Or they may simply wait for more specimens or data before making a formal classification.
Let’s look at some real examples. Duobrachium sparksae, a deep-sea ctenophore discovered in 2015, was only observed via ROV camera, with no physical specimens collected. Dendrogramma, found in 2014, had so few preserved specimens that scientists couldn’t even determine its phylum with certainty. A newly described polychaete worm from 2020 is referred to as cf. Novibrachium, using the cf. qualifier because only a single incomplete specimen was available for study.
Genus classification for such organisms remains provisional until additional research provides more complete information. This highlights the dynamic nature of taxonomic science, where classifications evolve as our knowledge grows.
Modern approaches to genus classification have dramatically transformed how scientists organize and understand biological diversity.
Traditional classification methods relied primarily on morphology and subjective observations of physical traits.
In contrast, modern approaches utilize molecular data and computational methods to create more objective and evolutionarily accurate classifications.
DNA sequencing has become the cornerstone of modern taxonomic classification. By analyzing genetic code directly, scientists can identify precise evolutionary relationships between organisms.
Computational phylogenetics uses advanced algorithms to analyze genetic data and reconstruct evolutionary relationships. These methods create statistically robust phylogenetic trees that reflect true evolutionary history.
Cladistics has become fundamental to modern genus classification. This approach requires that genera represent monophyletic groups – containing all descendants of a common ancestor.
Collectively, these modern approaches have transformed genus classification from a subjective art to a data-driven science, creating taxonomic systems that better reflect the true evolutionary history of life.
The Future of Genus Classification builds upon centuries of taxonomic tradition while embracing cutting-edge technologies.
Future approaches to genus classification will increasingly rely on genomic data, integration of molecular and morphological evidence, computational phylogenetics, and even machine learning algorithms.
Taxonomists face significant challenges, including balancing nomenclatural stability with scientific accuracy, addressing cryptic diversity revealed by molecular studies, and reconciling molecular findings with traditional classification approaches.
Recent discoveries like Homo naledi demonstrate how new findings continue to refine our understanding of even well-studied genera.
Emerging trends include integrative taxonomy that synthesizes multiple data sources, environmental DNA sampling for detecting cryptic diversity, advanced morphometric techniques, and collaborative global initiatives to standardize genus concepts.
For instance, genomic studies in the Solanum genus are reshaping our understanding of tomato family relationships at the genus level.
Despite these changes, the genus category maintains its fundamental importance as a framework for biodiversity studies, ecological research, conservation efforts, and scientific communication across disciplines.
The future of genus classification envisions a dynamic, integrative concept that balances evolutionary history, practical utility, and ongoing scientific progress.
As we continue to discover new species and develop new technologies, the genus concept will evolve while remaining a cornerstone of biological classification.
Study Materials
No study materials available for this video.
Related Videos
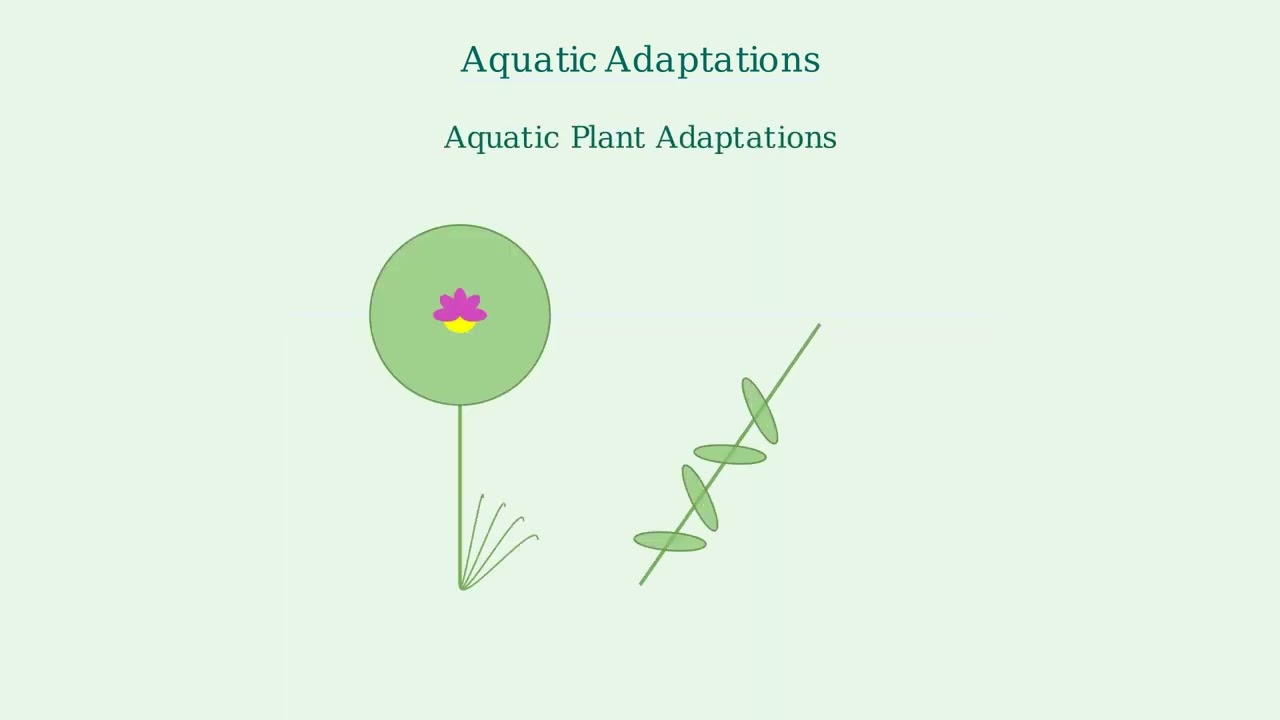
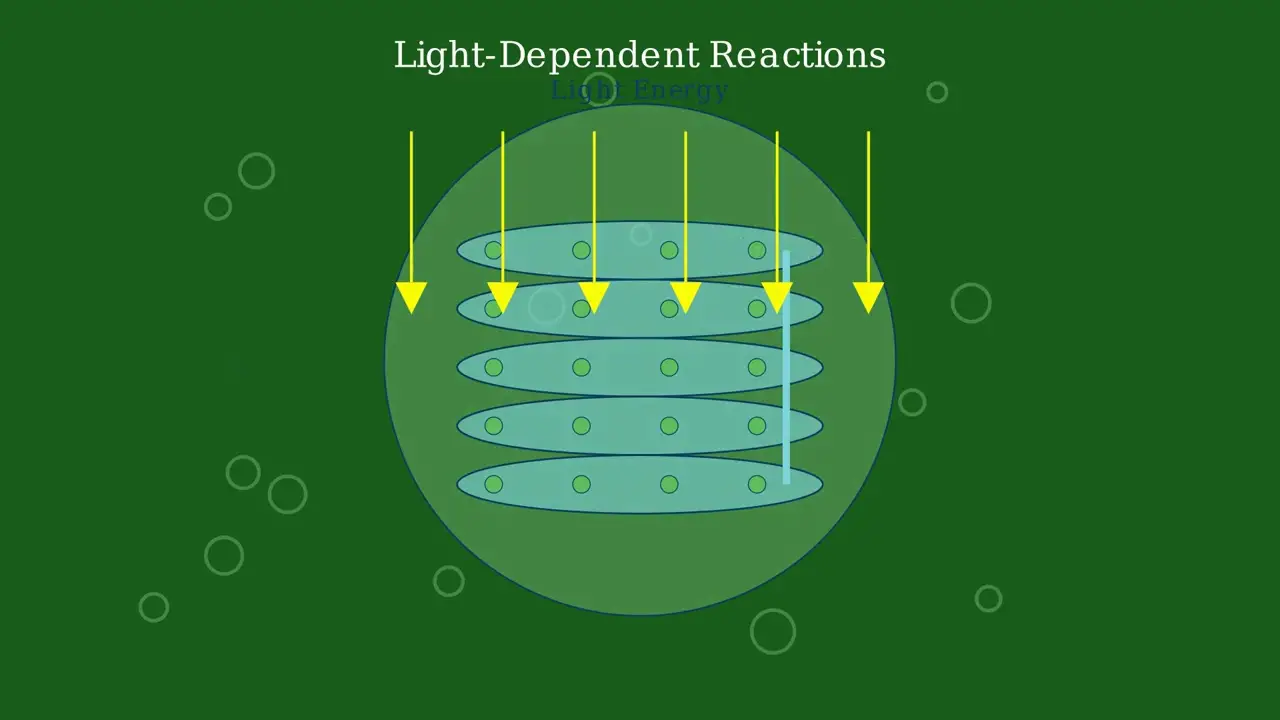
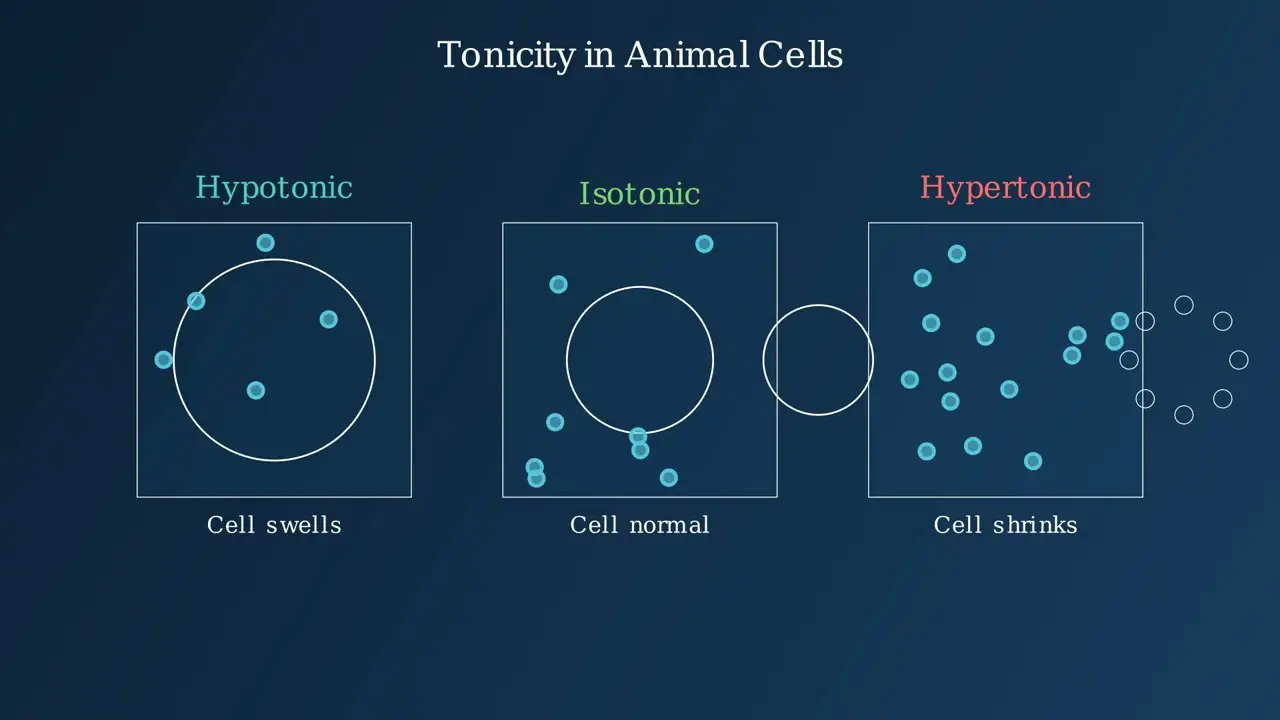
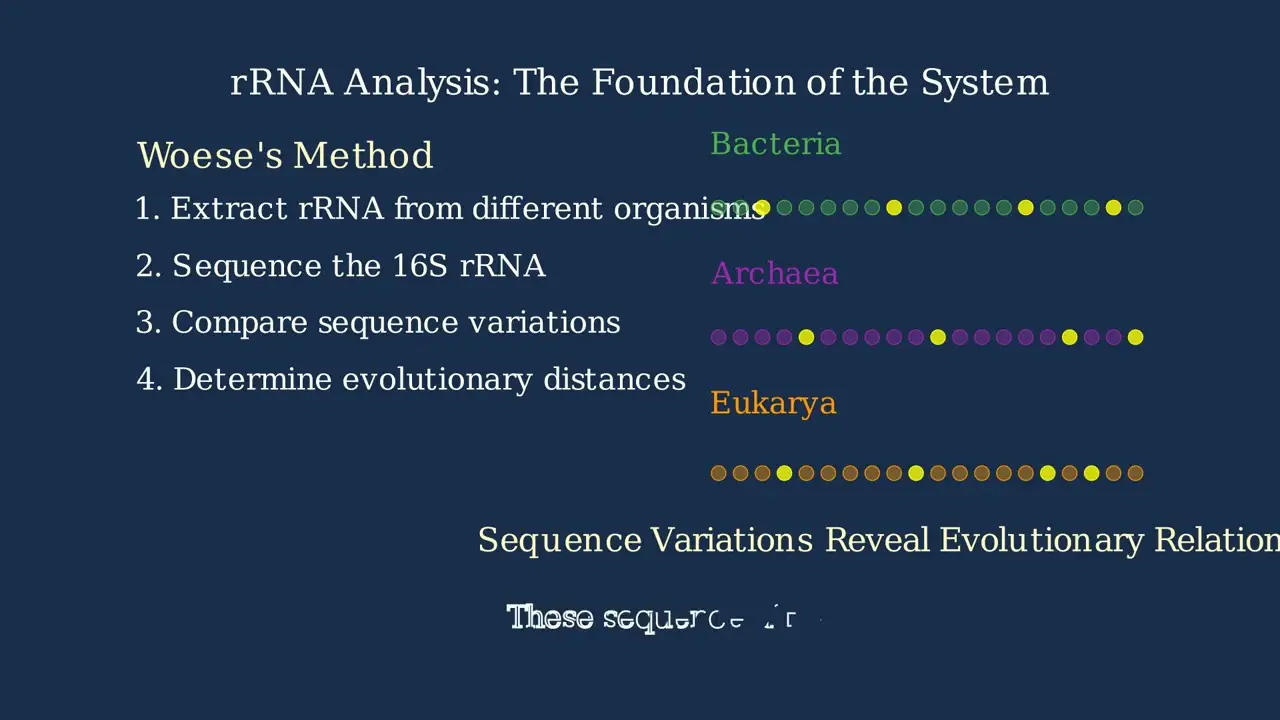
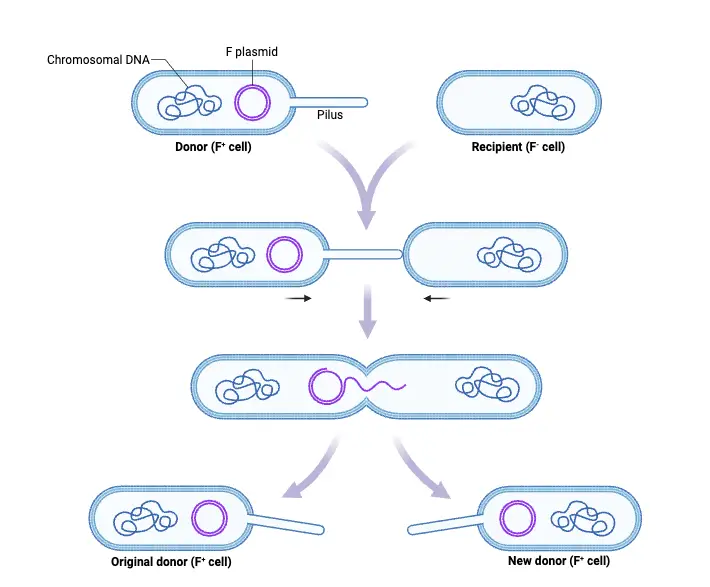
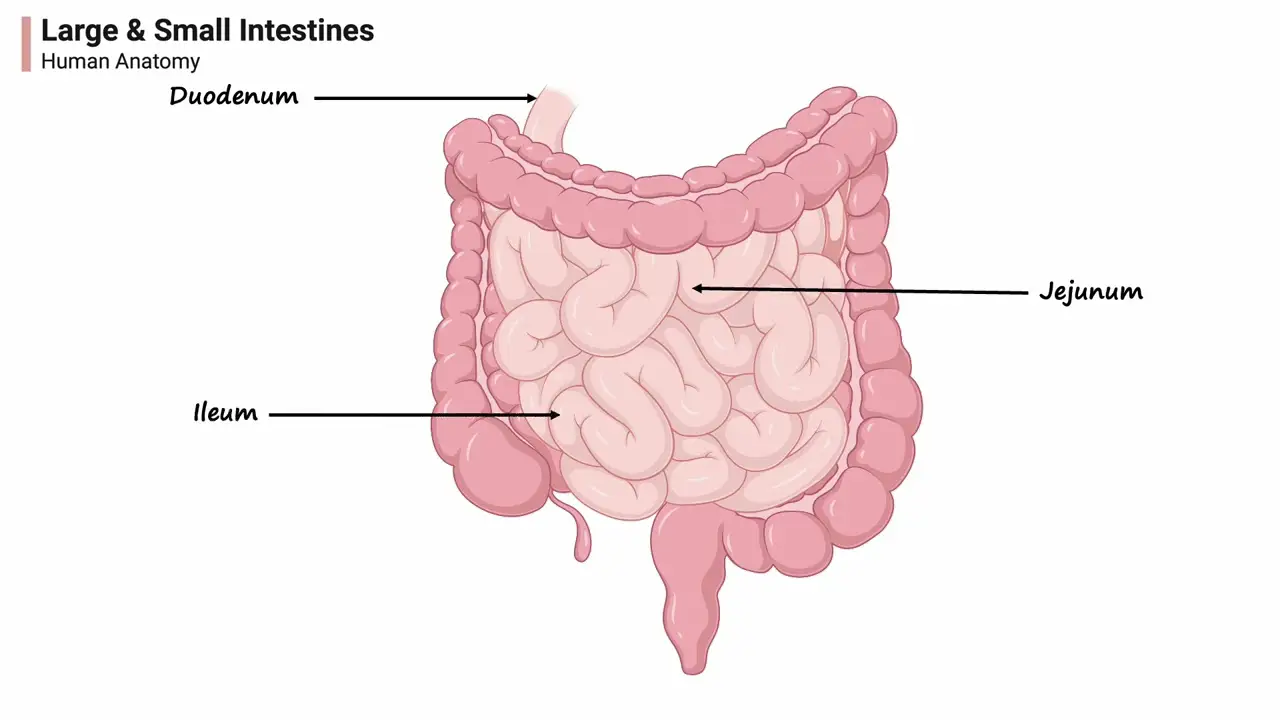
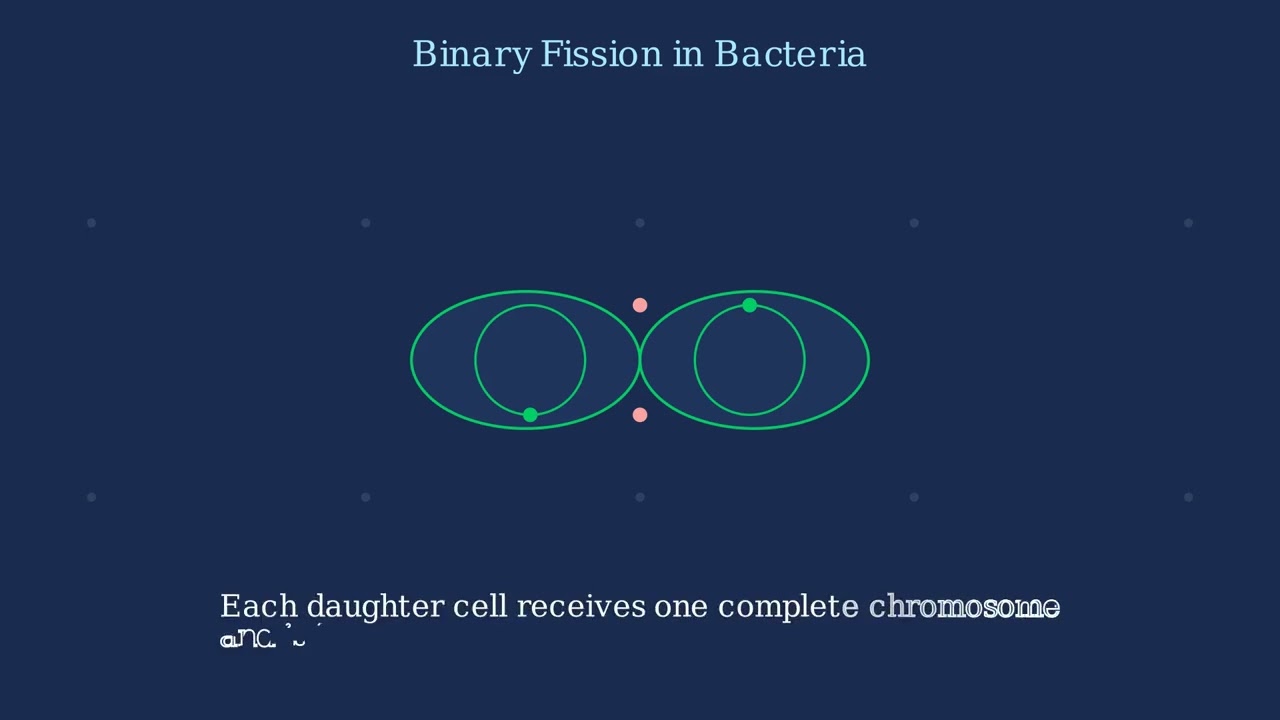
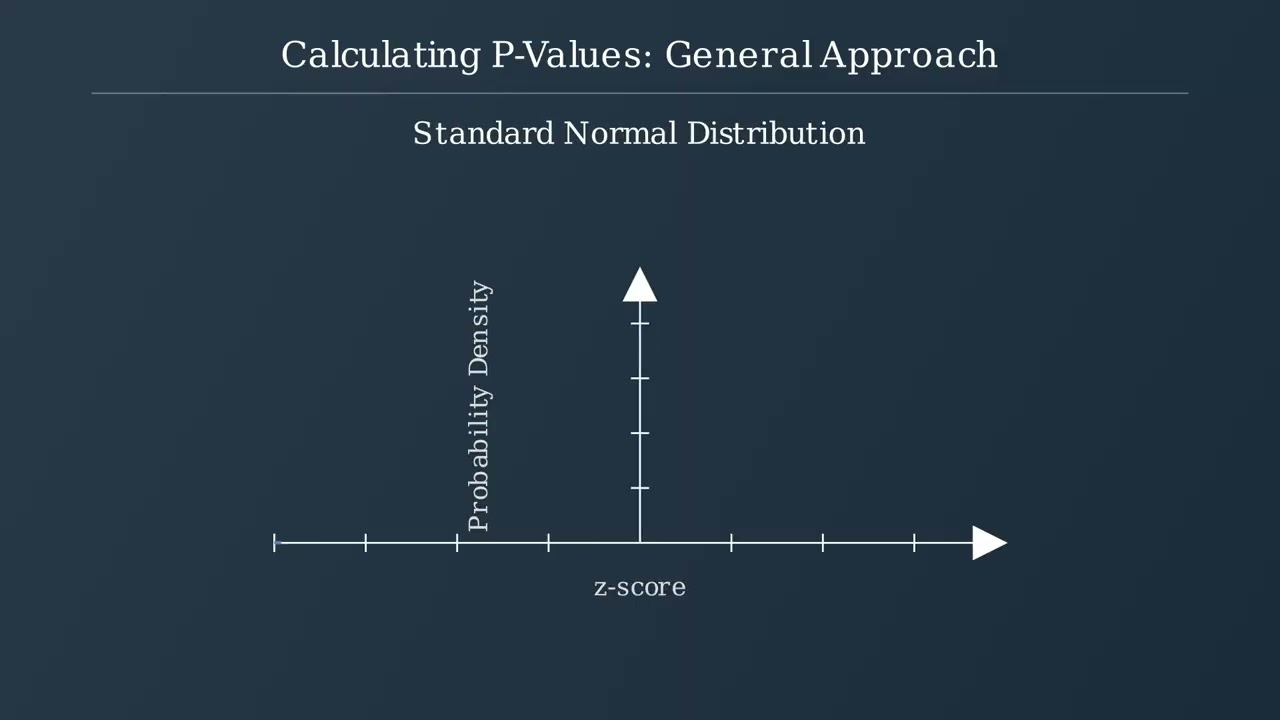
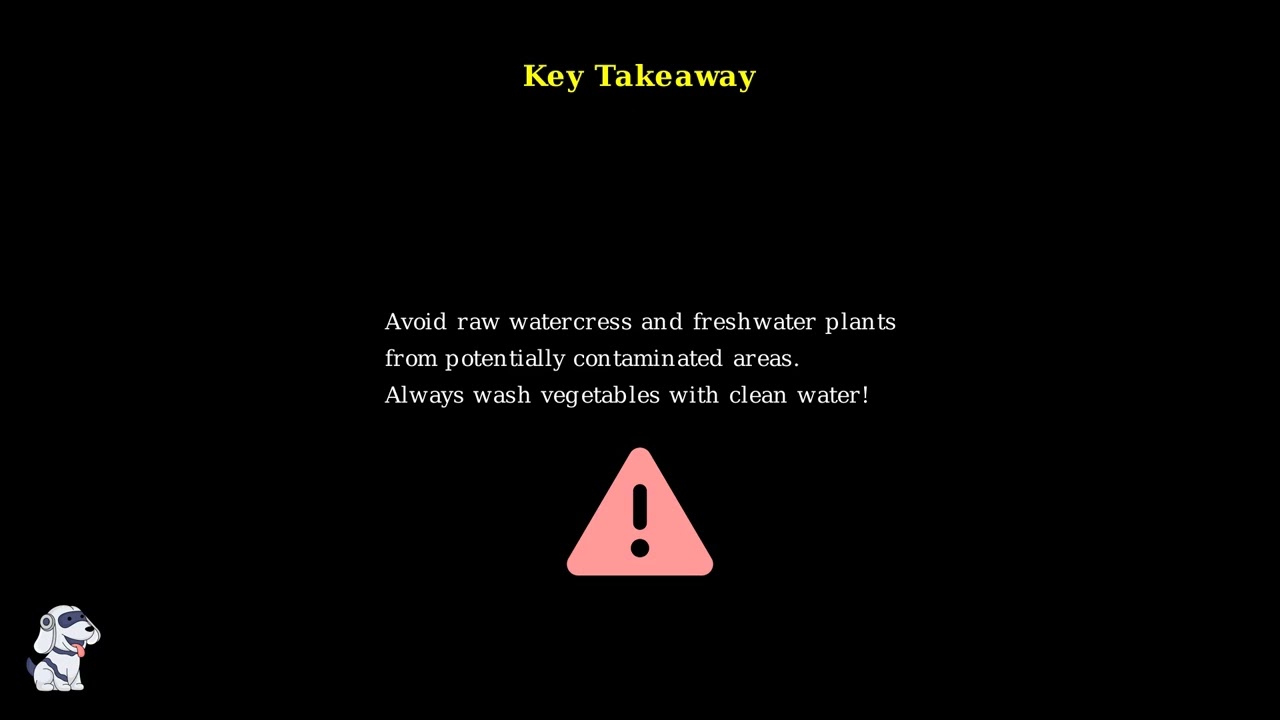