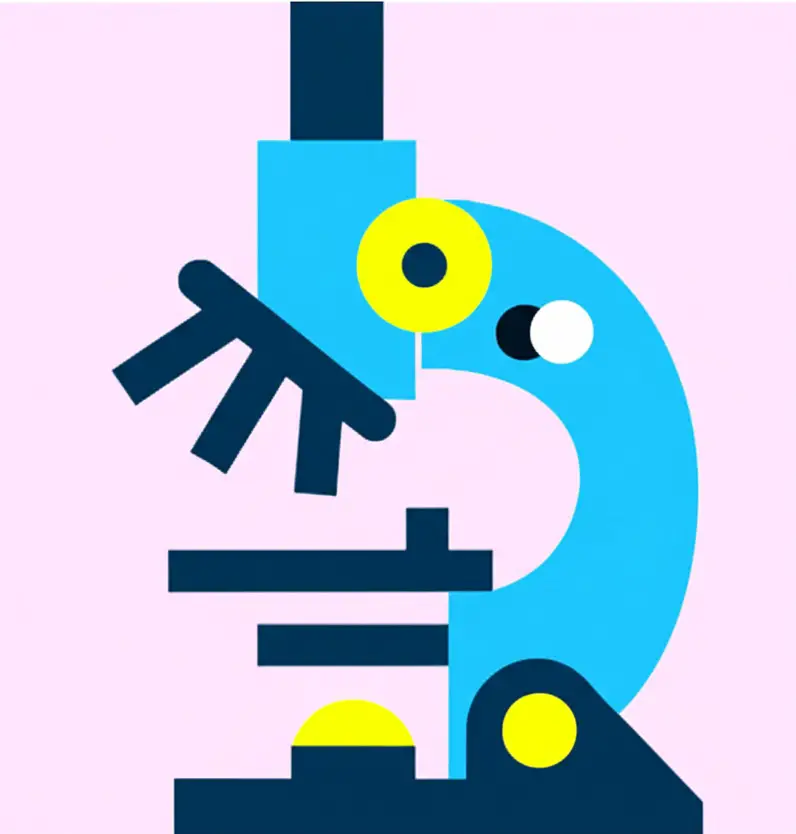
Sourav Pan
Transcript
Introduction to Sterilization
Sterilization is defined as the complete elimination of all forms of microbial life.
This includes bacteria, viruses, fungi, and bacterial spores.
It’s important to understand the difference between sterilization and disinfection.
Sterilization completely eliminates all microorganisms, while disinfection only reduces the number of pathogens to a safe level.
Sterilization is crucial across multiple industries.
In healthcare, it prevents infections during surgeries and medical procedures.
In laboratory settings, it ensures experimental accuracy by eliminating contamination.
And in food processing, it extends shelf life and ensures consumer safety.
Let’s visualize the sterilization process.
During sterilization, all microorganisms including resistant bacterial spores are completely destroyed.
In this course, we will explore seven major physical sterilization methods used across different industries.
Each method has specific applications, advantages, and limitations that we’ll explore in the following sections.
Radiation sterilization employs different types of radiation to eliminate microorganisms.
Gamma rays from Cobalt-60 are high-energy photons that penetrate deeply into materials.
Electron beam uses accelerated electrons, while UV radiation is effective for surface sterilization.
Ionizing radiation damages the DNA of microorganisms through direct and indirect mechanisms.
When gamma radiation from Cobalt-60 interacts with cells, it creates free radicals and directly breaks chemical bonds in the DNA.
These DNA breaks prevent microbial reproduction and cause cell death, effectively sterilizing the material.
Radiation sterilization is widely used for medical supplies, including syringes, implants, and surgical tools.
In the pharmaceutical industry, it’s used for raw materials and some finished products.
For food preservation, radiation extends shelf life of spices, fruits, and certain meat products.
Radiation sterilization offers several advantages and has some limitations to consider.
Advantages include deep penetration ability, no temperature increase, the ability to sterilize sealed packages, and no chemical residues.
Limitations include potential material degradation, specialized equipment requirements, high initial costs, and incompatibility with certain materials.
For medical devices, the typical sterilization dose is 25 kilograys, which provides a sterility assurance level of 10 to the minus 6.
Various equipment is used for radiation sterilization, including gamma irradiation chambers using Cobalt-60, electron beam accelerators, X-ray conversion systems, and UV sterilization cabinets.
Radiation sterilization is highly effective and widely used for terminal sterilization of many products in healthcare and food industries.
Filtration is a physical method of sterilization that separates microorganisms from liquids and gases by passing them through specialized membrane filters.
Membrane filters typically have pore sizes ranging from 0.2 to 0.45 micrometers. These microscopic openings are small enough to trap bacteria and other microorganisms.
When liquids or gases pass through these filters, microorganisms like bacteria are trapped on the filter surface, while the filtered fluid passes through.
However, standard filters with 0.2-0.45 micrometer pores cannot trap viruses, which are typically much smaller.
Filtration methods are widely used across various fields, particularly for sterilizing heat-sensitive materials.
In pharmaceutical manufacturing, filtration sterilizes injectable solutions, biological products, and culture media. Laboratories use it for cell culture media and buffer solutions, while hospitals rely on filtration for IV fluids and water purification.
Filtration sterilization offers several advantages and has some important limitations to consider.
Among its advantages, filtration is ideal for heat-sensitive materials, leaves no chemical residues, and preserves the properties of the filtered product.
However, limitations include filter clogging that requires replacement, the inability to remove viruses with standard filters, and limited volume capacity.
HEPA filters, which stands for High-Efficiency Particulate Air filters, are specially designed for air filtration in critical environments.
These filters can remove 99.97 percent of particles that are 0.3 micrometers or larger from the air, making them essential for cleanrooms, operating theaters, and other controlled environments.
HEPA filtration is critical in pharmaceutical cleanrooms, operating theaters, biological safety cabinets, and industries requiring high-purity air such as aerospace and microelectronics manufacturing.
Sonic and ultrasonic sterilization harnesses the power of sound waves to destroy microorganisms.
Sound waves exist across a spectrum of frequencies. We can use both audible sound, ranging from twenty hertz to twenty kilohertz, as well as ultrasonic frequencies above twenty kilohertz, which are beyond human hearing.
The key mechanism of ultrasonic sterilization is a process called cavitation.
When ultrasonic waves pass through a liquid medium, they create regions of high and low pressure.
In the low pressure regions, microscopic bubbles form as the liquid temporarily vaporizes.
These bubbles rapidly collapse when they enter high pressure regions, creating powerful shockwaves.
These shockwaves damage cell membranes, disrupt cellular function, and ultimately kill microorganisms.
Ultrasonic sterilization systems operate within specific technical parameters.
Frequencies typically range from twenty kilohertz to two megahertz, with higher frequencies providing more precision but less power.
Power density varies from one hundred to one thousand watts per liter, adjusted based on the specific application and required level of sterilization.
Treatment time can vary significantly, from just seconds to thirty minutes, depending on the microorganism type and the degree of sterilization needed.
Ultrasonic sterilization has several important applications across different industries.
In food processing, ultrasonic treatments can inactivate enzymes and microorganisms while preserving nutrients and extending shelf life, all with minimal heat damage.
For medical devices, ultrasonic cleaners are particularly effective at removing contaminants from complex instruments, reaching crevices that are difficult to clean through other methods.
In water treatment, ultrasonic waves can break down contaminants, disrupt bacterial aggregates, and enhance chemical treatments for both industrial and municipal applications.
Let’s examine the advantages and limitations of sonic and ultrasonic sterilization methods.
The advantages include low temperature operation, which preserves heat-sensitive materials, no chemical residues, ability to penetrate complex shapes, environmental friendliness, and compatibility with other sterilization methods.
However, there are important limitations to consider. Effectiveness can be inconsistent, making it typically used as a supplementary rather than standalone method. It has limited penetration in dense materials, may not eliminate all bacterial spores, and the equipment can be costly.
Solar Disinfection, or SODIS, is a water treatment method that uses UV-A radiation and thermal effects from sunlight to inactivate pathogenic microorganisms.
The SODIS process is straightforward. First, fill clear plastic or glass bottles with water.
Next, expose these bottles to direct sunlight for at least six hours, ideally on a reflective surface.
During exposure, UV-A radiation penetrates the water while solar heat raises the temperature, working together to inactivate pathogens.
At a microscopic level, SODIS works through two main mechanisms. UV-A radiation damages the DNA and proteins of microorganisms.
As exposure time increases, pathogens gradually become inactivated. The process may be slow, but after six hours, a significant reduction in viable microorganisms is achieved.
SODIS offers several significant advantages. It’s low-cost, simple to implement, requires no chemicals, is widely accessible, and provides a sustainable solution for water treatment.
However, SODIS also has important limitations. It’s weather dependent, has limited volume capacity, requires at least six hours of sunlight, is less effective on cloudy days, and doesn’t work against all types of pathogens.
SODIS is particularly valuable in resource-limited settings. It serves rural communities where conventional water treatment is unavailable, provides relief during humanitarian crises, and offers a solution during emergency situations.
It’s important to note that SODIS is primarily a disinfection method rather than complete sterilization. It significantly reduces microbial load but does not eliminate all microorganisms. Some pathogens, particularly certain protozoa and bacterial spores, may show resistance to this treatment.
SODIS can achieve up to 99.9% reduction of certain bacteria like E. coli, Salmonella, and Vibrio cholerae. However, it’s less effective against some viruses and protozoa, and is not recommended for heavily contaminated water.
For best results, use clear, not colored bottles, place them on reflective surfaces to maximize exposure, pre-filter turbid water to improve light penetration, and double the exposure time on cloudy days.
Study Materials
No study materials available for this video.
Related Videos
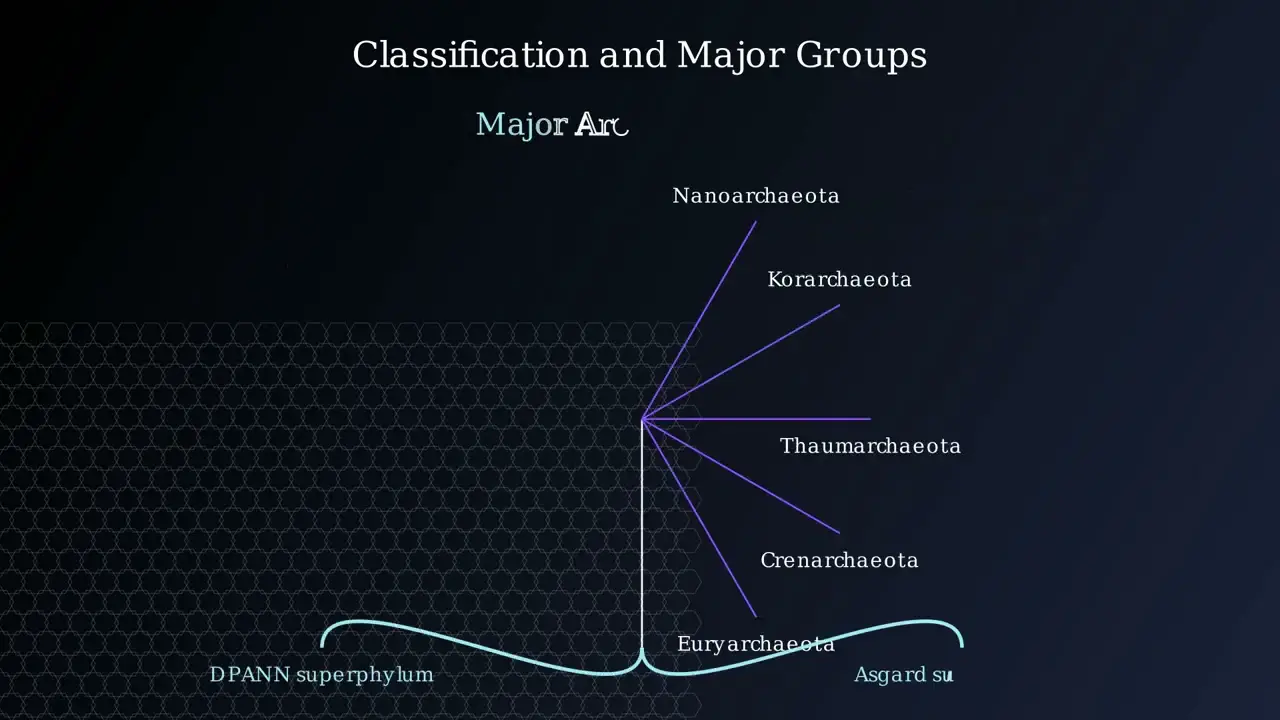
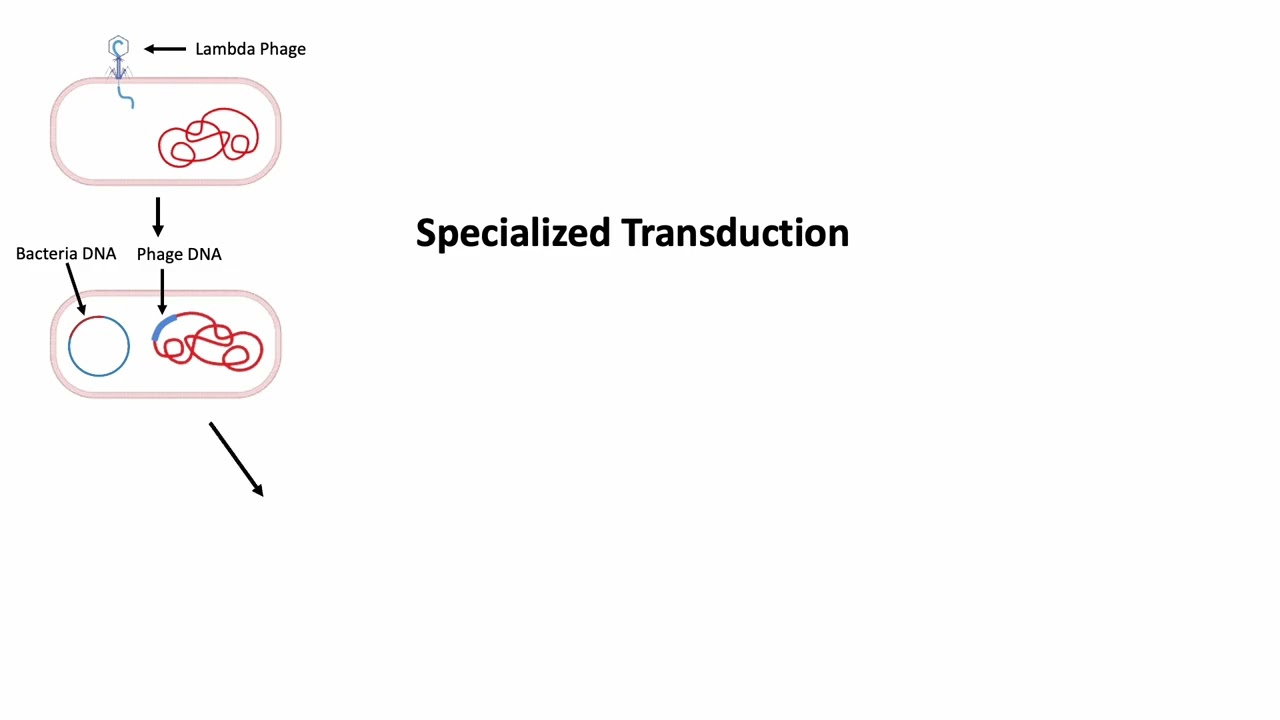
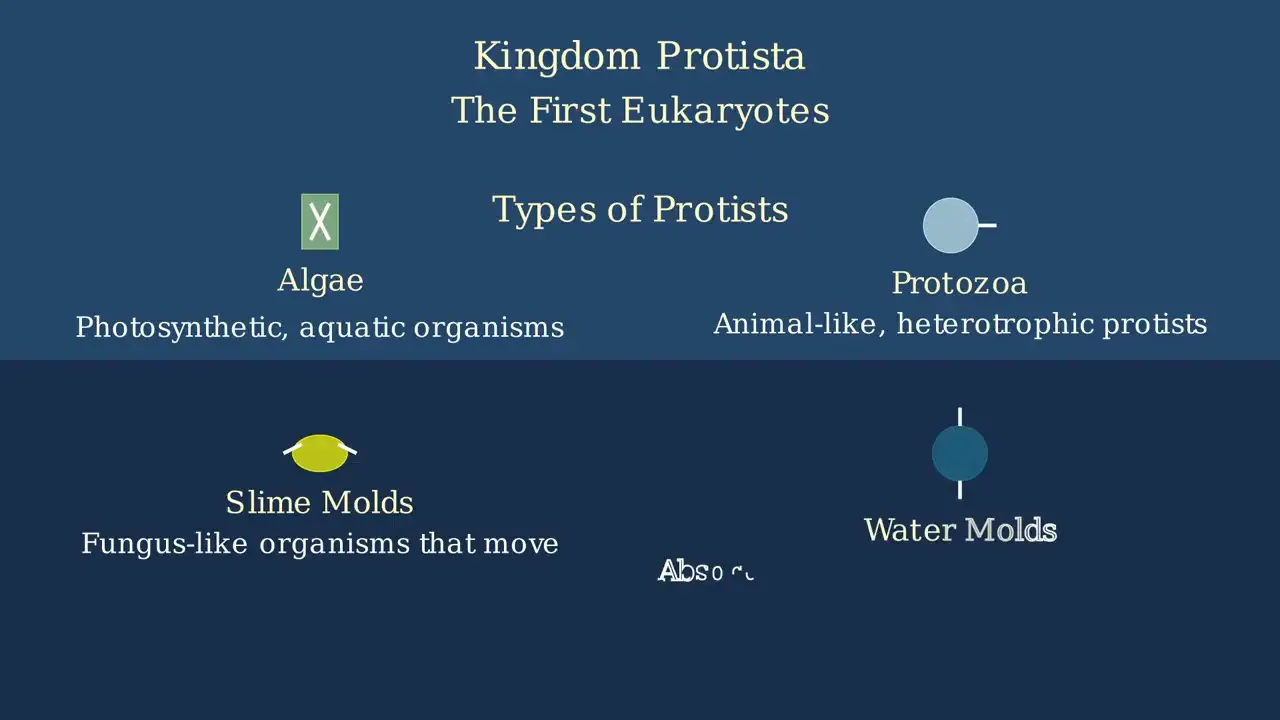
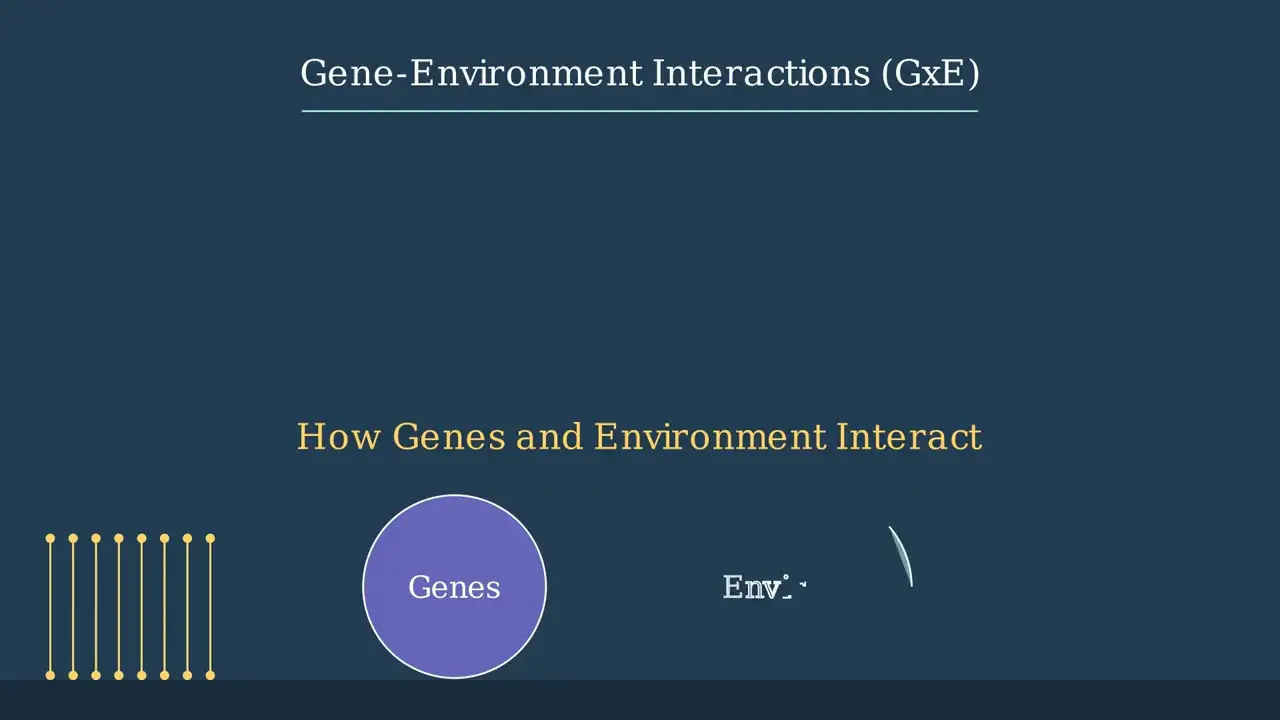
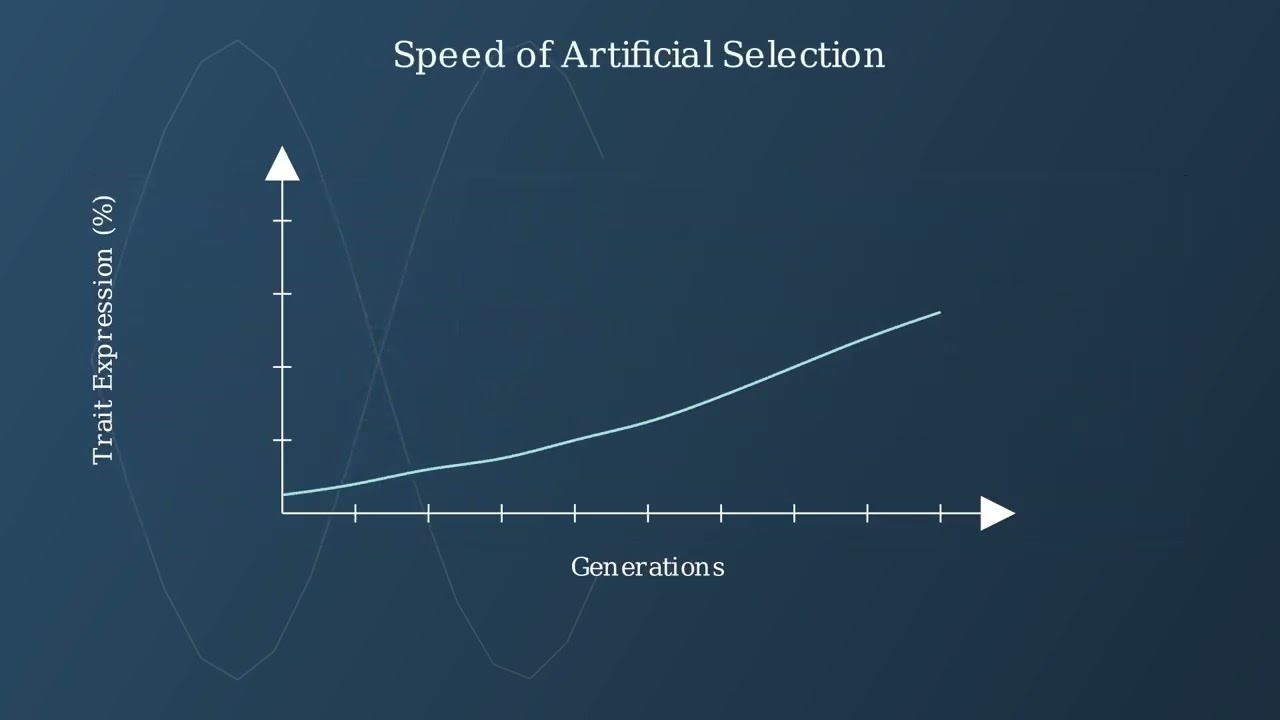
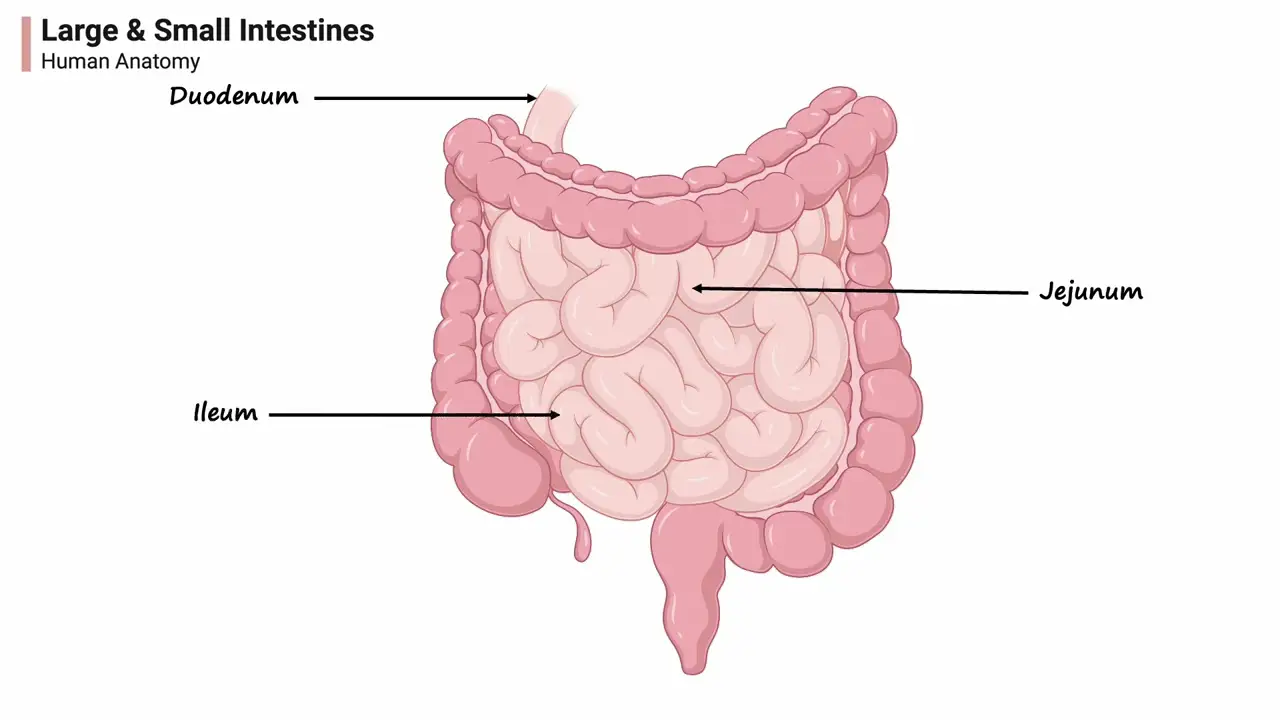
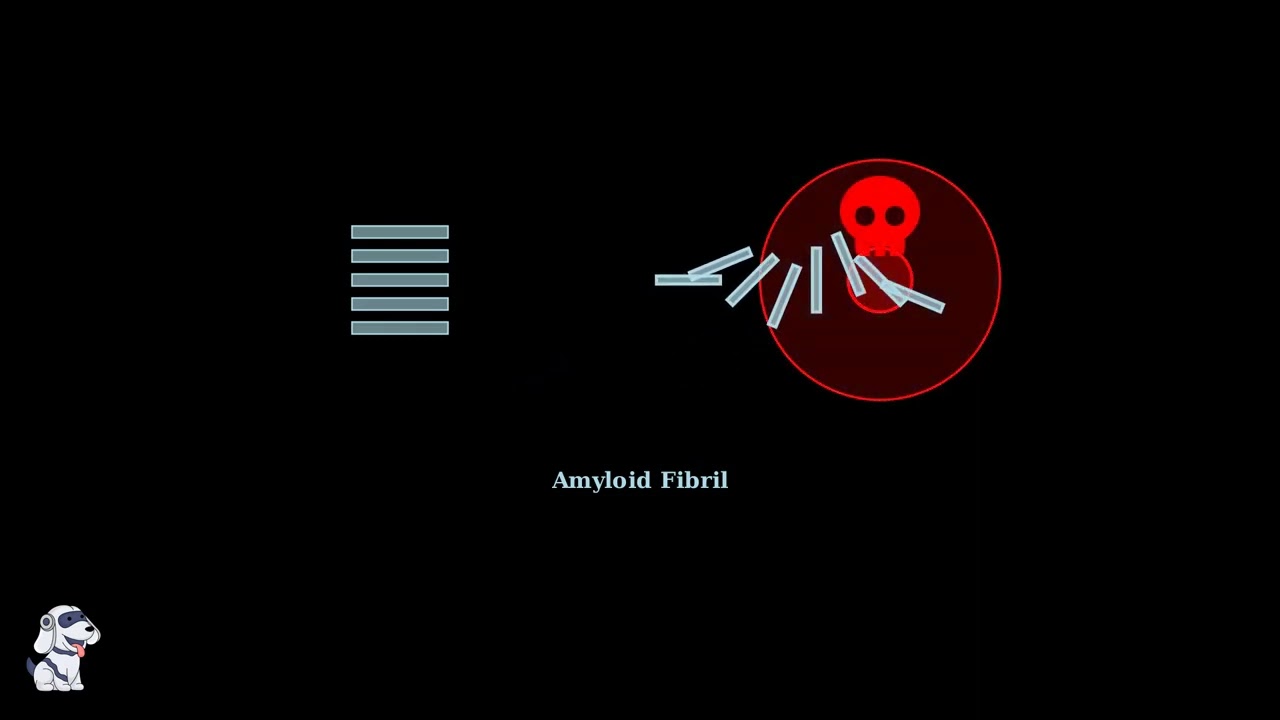
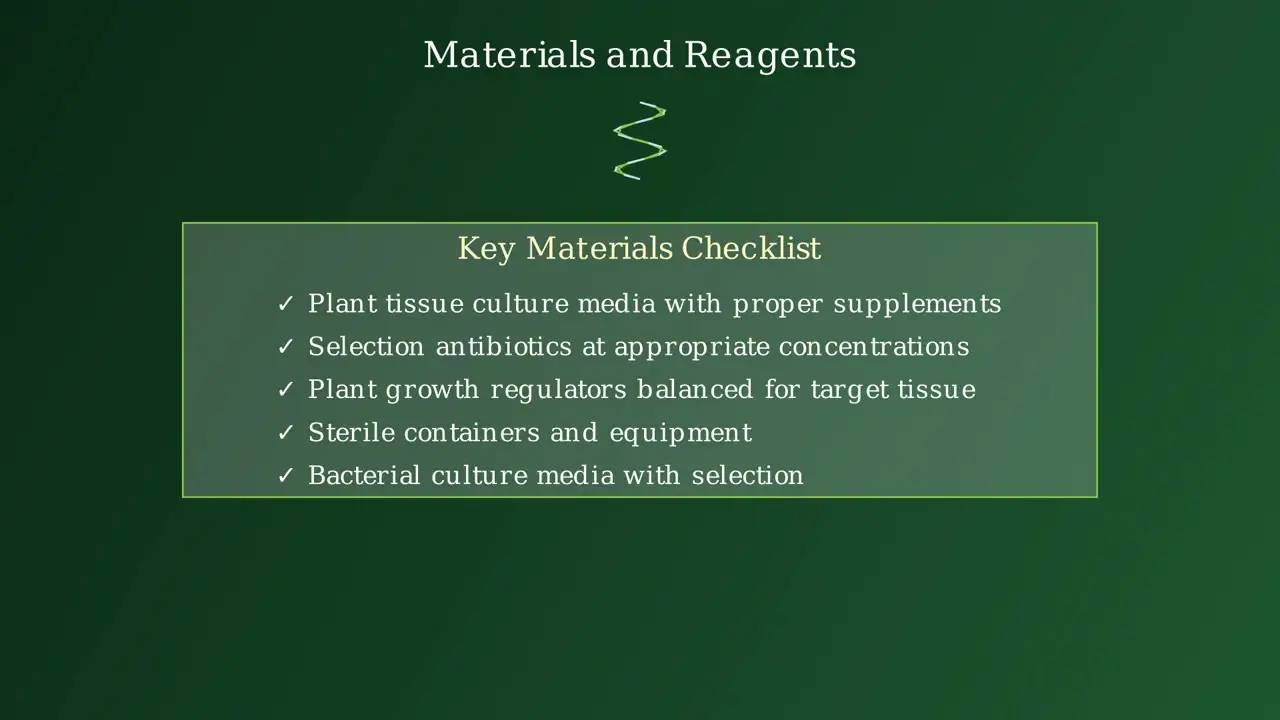
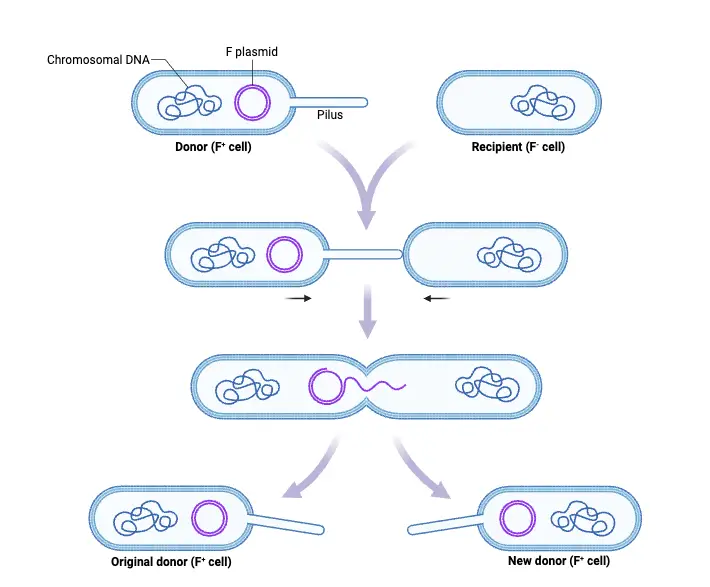