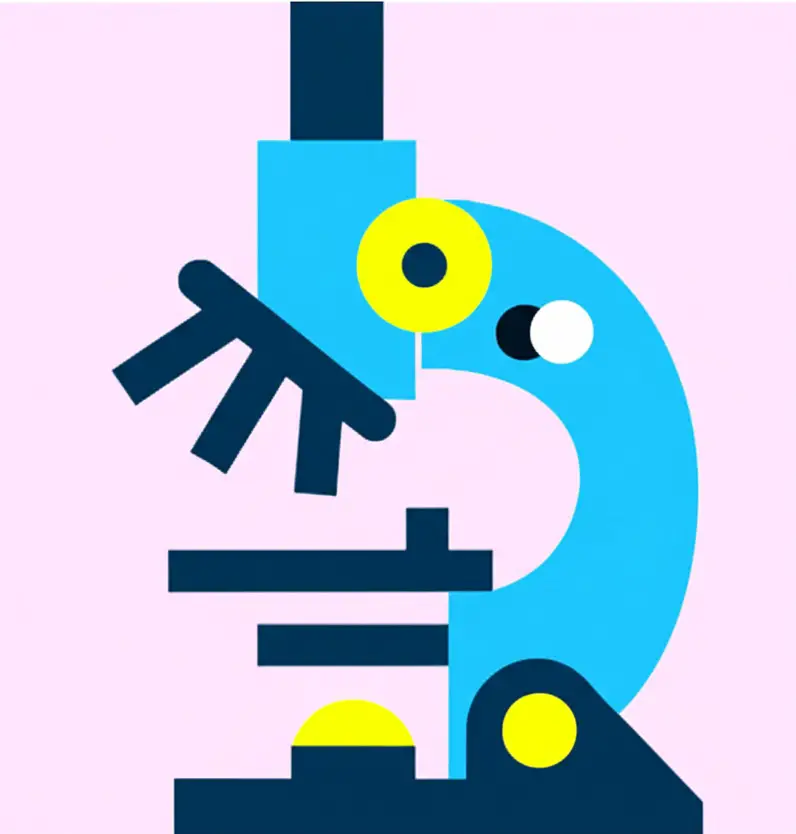
Sourav Pan
Transcript
Introduction to Archaea, a fascinating domain of life.
Archaea represent one of the three fundamental domains of life, alongside Bacteria and Eukarya.
Archaea were first discovered in extreme environments in 1977. Initially classified as strange bacteria, they were later recognized as a completely separate domain. Today, we know they exist in a wide range of habitats from hydrothermal vents to oceans, soils, and even within the human microbiome.
Archaea hold a unique position in the evolutionary tree of life. Genetic studies have revealed that Archaea are more closely related to Eukarya than to Bacteria. This makes them crucial for understanding the evolution of complex life and the nature of the last universal common ancestor, or LUCA.
Despite superficial similarities to bacteria in appearance, Archaea represent a fundamentally distinct form of life. They differ in cell wall composition, membrane structure, genetic mechanisms, and metabolic pathways. The unique biochemistry of Archaea enables them to thrive in environments that would be hostile to most other life forms.
In summary, Archaea represent a fundamental and distinct domain of life that greatly expands our understanding of life’s diversity and evolutionary history. Their unique biology continues to challenge our assumptions about the limits and possibilities of life.
Archaea have unique structural and biochemical features that distinguish them from both bacteria and eukaryotes.
Like bacteria, archaeal cells lack a nucleus and membrane-bound organelles, classifying them as prokaryotes.
Their genetic material consists of a circular DNA molecule that floats freely in the cytoplasm, along with ribosomes for protein synthesis.
What makes archaeal cells truly unique is their membrane structure. Unlike bacteria and eukaryotes, which have lipids with ester linkages, archaea possess ether-linked lipids.
These ether linkages are more resistant to extreme conditions, allowing archaea to survive in harsh environments where other organisms cannot.
Archaeal cells exhibit diverse morphologies, including cocci or spherical forms, rod-shaped cells, and various irregular structures.
Their size typically ranges from 0.1 to 15 micrometers, similar to bacteria but generally smaller than eukaryotic cells.
Let’s compare the key structural features of archaea with bacteria and eukaryotes.
While archaea and bacteria both lack a nucleus, archaeal cell walls differ significantly from bacterial peptidoglycan. They typically contain pseudopeptidoglycan or surface layer proteins.
The most distinctive feature remains their unique ether-linked membrane lipids, which provide stability in extreme environments. Their DNA organization is similar to bacteria, but their genetic machinery more closely resembles eukaryotes in many ways.
These unique structural features help explain how archaea have adapted to thrive in diverse environments and represent a distinct domain of life.
Despite their superficial similarities, Archaea and Bacteria have several key structural and molecular differences.
These differences are significant enough to classify Archaea as a separate domain of life.
The most striking difference is in their membrane composition. Archaeal membranes contain ether-linked lipids with branched hydrocarbon chains, while bacterial membranes have ester-linked lipids with straight chains.
Another key difference is in their cell walls. Bacteria contain peptidoglycan, while Archaea lack this component and instead may have pseudopeptidoglycan or other unique polymers.
The ribosomal structures of Archaea are more similar to those found in eukaryotes, with unique proteins that distinguish them from bacterial ribosomes.
Archaea also have multiple RNA polymerase types similar to eukaryotes, while bacteria have only a single type.
These fundamental differences reflect the separate evolutionary history of Archaea and Bacteria.
These differences led Carl Woese to propose in 1977 that Archaea represent a separate domain of life, distinct from both Bacteria and Eukarya.
Understanding these distinguishing features is crucial for comprehending the diversity and evolutionary relationships among microorganisms.
Archaea possess unique genetic and molecular characteristics that set them apart from both bacteria and eukaryotes.
Their genetic material exists as circular chromosomes, similar to bacteria, but with some important differences.
Unlike bacteria, but similar to eukaryotes, some archaeal species wrap their DNA around histone proteins.
Archaea display an intriguing mixture of bacterial and eukaryotic genetic features.
They possess unique systems for processing their genetic information.
Archaeal DNA replication has more similarities with eukaryotes than with bacteria.
While bacteria initiate DNA replication at a single origin, archaea, like eukaryotes, can have multiple origins of replication.
The proteins involved in archaeal DNA replication are more similar to those found in eukaryotes.
For example, archaea use MCM helicase to unwind DNA during replication, just like eukaryotes, rather than the DnaB helicase found in bacteria.
The transcription machinery in archaea shares features with both bacteria and eukaryotes, but is generally more similar to eukaryotes.
While bacteria have a single RNA polymerase, archaea have multiple types, similar to the RNA polymerase I, II, and III found in eukaryotes.
In protein synthesis, archaea use methionine as the initiator amino acid, just like eukaryotes.
Their translation factors also more closely resemble those found in eukaryotes.
Even the structure of archaeal ribosomes shares more similarities with eukaryotic ribosomes than with bacterial ones.
One of the most striking similarities between archaea and eukaryotes is the presence of histone proteins in many archaeal species.
These histone proteins help package and organize the DNA, forming structures similar to the chromatin found in eukaryotes.
While not all archaeal species have histones, they are present in many archaeal lineages.
Archaeal histones are structurally similar to the core histones found in eukaryotes.
However, they typically form tetramers rather than the octamers seen in eukaryotes.
Archaeal histones wrap about sixty base pairs of DNA, compared to the one hundred and forty-seven base pairs wrapped by eukaryotic nucleosomes.
The presence of histones in archaea supports theories about their evolutionary relationship to the ancestors of eukaryotic cells.
The genetic and molecular characteristics of archaea have significant implications for our understanding of evolution.
The similarities between archaeal and eukaryotic genetic processing machinery support the eocyte hypothesis.
This hypothesis suggests that eukaryotes arose from within the archaeal domain, specifically from a lineage related to the TACK superphylum of archaea.
These molecular similarities challenge the traditional three-domain system of classification and point to a closer relationship between archaea and eukaryotes than previously thought.
Now we’ll explore methanogens, a unique group of Archaea that produce methane gas as a byproduct of their metabolism.
Methanogens have a unique cell structure and are strictly anaerobic, meaning they can only survive in environments without oxygen.
The metabolism of methanogens is remarkable. They can convert carbon dioxide and hydrogen into methane and water, generating energy in the process.
This process involves a complex pathway called methanogenesis, which occurs in several steps using unique coenzymes found only in methanogens.
These archaea contain enzymes and coenzymes that are not found in any other organism, such as coenzyme F420, which gives methanogens their characteristic blue-green fluorescence.
Methanogens thrive in various anaerobic environments. They’re abundant in wetlands and marshes, where they produce the methane bubbles seen in muddy areas.
They’re also found in the digestive systems of animals like cows and termites, contributing to these animals’ ability to digest plant material.
In deep sea sediments, methanogens function under high pressure in oxygen-free conditions.
And in landfills, they contribute to the production of landfill gas, which is mostly methane.
Methanogens have important practical applications in several fields. In biogas production, they convert organic waste into usable energy in anaerobic digesters.
In wastewater treatment, methanogens break down organic material in anaerobic digesters, reducing sludge volume while producing methane that can be captured and used as fuel.
These applications are becoming increasingly important as we search for sustainable energy sources and waste management solutions.
The unique metabolism of methanogens makes them fascinating subjects for both basic research and biotechnological applications.
The classification of Archaea has undergone significant revisions as molecular techniques have advanced.
Archaea is divided into several major phyla based primarily on 16S ribosomal RNA sequencing and other molecular markers.
Modern classification of Archaea relies on molecular techniques. The 16S ribosomal RNA gene has been particularly important, along with whole genome sequencing and protein marker analysis.
New archaeal groups continue to be discovered through environmental sampling and metagenomics. This process involves collecting samples from diverse environments, extracting DNA, and using bioinformatic analysis to identify novel lineages.
Archaea play crucial roles in global biogeochemical cycles, particularly in carbon, nitrogen, and sulfur cycling.
In the carbon cycle, methanogenic archaea are responsible for producing over ninety percent of all biological methane. These archaea convert carbon dioxide and hydrogen to methane in anaerobic environments like wetlands, rice paddies, and animal digestive tracts.
In the nitrogen cycle, ammonia-oxidizing archaea belonging to the phylum Thaumarchaeota oxidize ammonia to nitrite in oceans and soils. These archaea often outnumber bacteria in marine environments and play a dominant role in nitrification processes. Some archaea also participate in anaerobic ammonia oxidation, or anammox, contributing significantly to the global nitrogen budget.
In the sulfur cycle, various archaeal groups participate in both oxidative and reductive processes. At hydrothermal vents, thermophilic archaea oxidize reduced sulfur compounds like hydrogen sulfide. In marine sediments, other archaeal groups contribute to sulfate reduction. These processes are particularly important in deep-sea environments where archaea are key contributors to elemental cycling.
Archaea are globally distributed and highly abundant in diverse ecosystems. In oceans, they comprise twenty to forty percent of the total microbial biomass and are found from surface waters to the deepest trenches. In soils, they inhabit virtually all types of terrestrial environments, from agricultural fields to forests. In sediments, they play critical roles in both freshwater and marine systems. Importantly, archaea form complex ecological networks with bacteria and eukaryotes, contributing to ecosystem stability.
Despite often being overlooked, archaea are critical contributors to global biogeochemical cycling. They regulate greenhouse gas emissions, including methane and nitrous oxide, which have significant implications for climate change. In extreme environments, archaea are often the dominant microorganisms, stabilizing ecosystem functions under harsh conditions. Their contributions to ecosystem services must be considered in climate models and biodiversity conservation efforts.
Study Materials
No study materials available for this video.
Related Videos
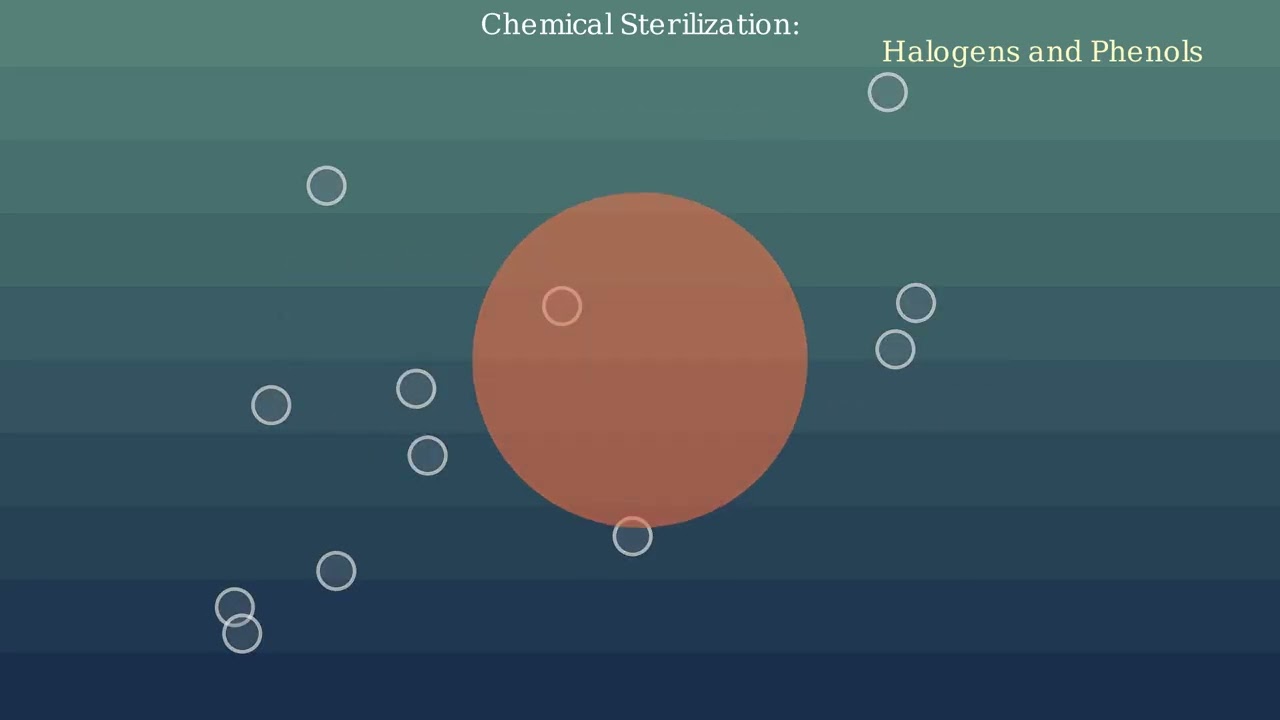
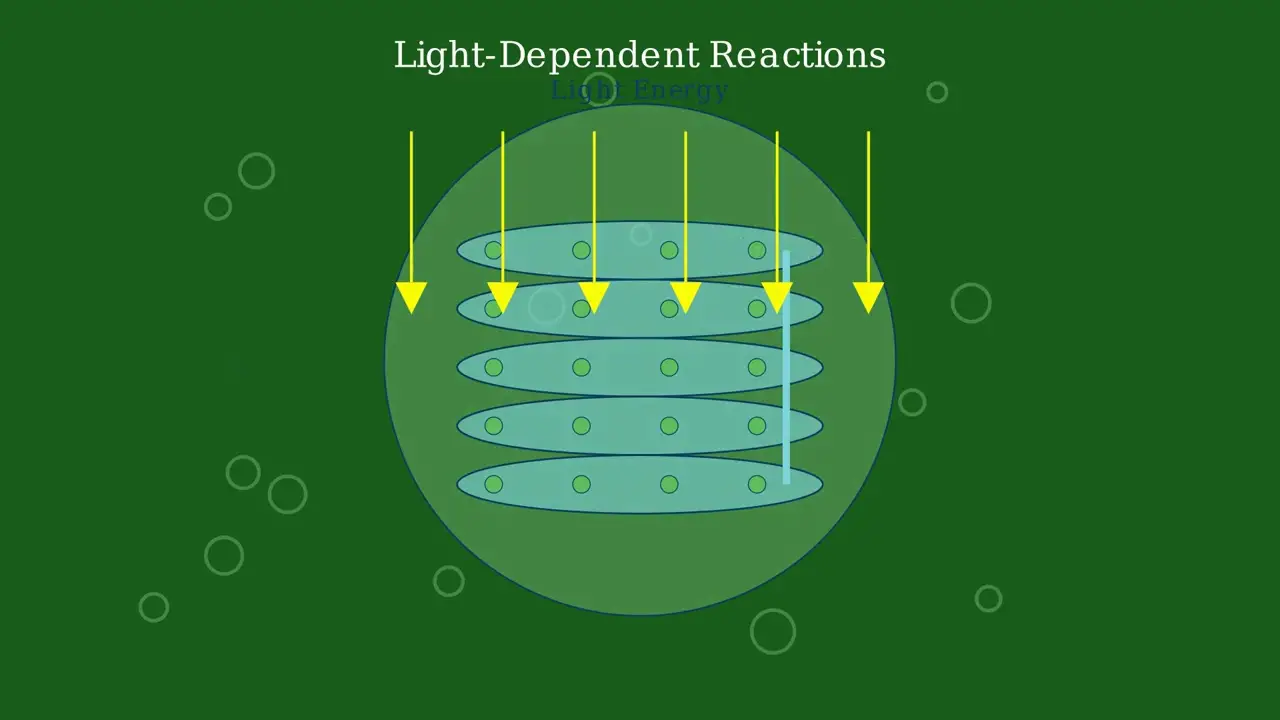
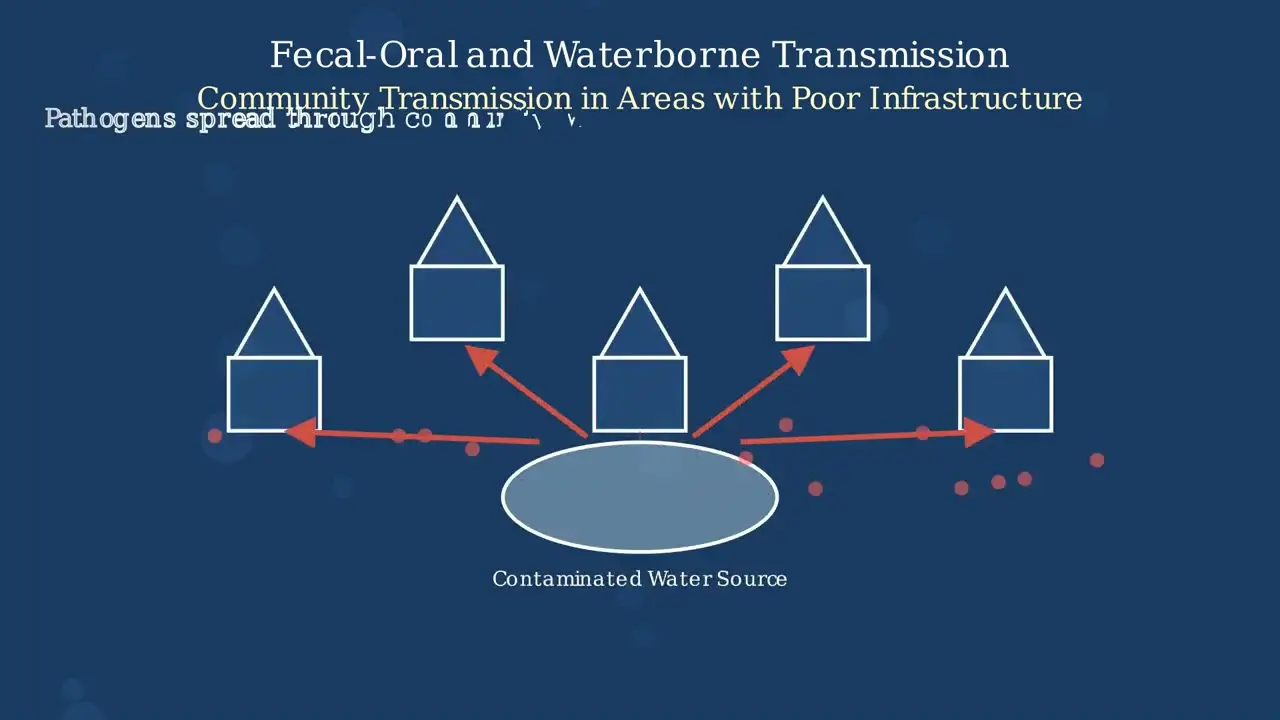
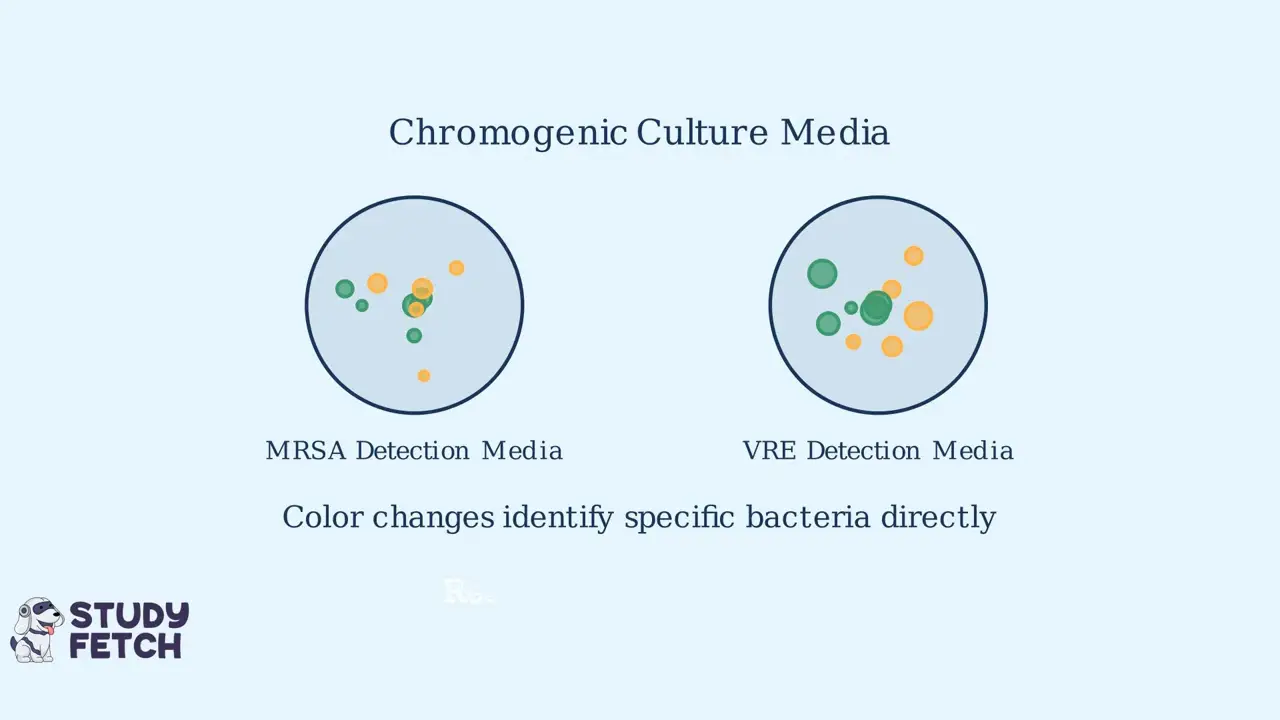
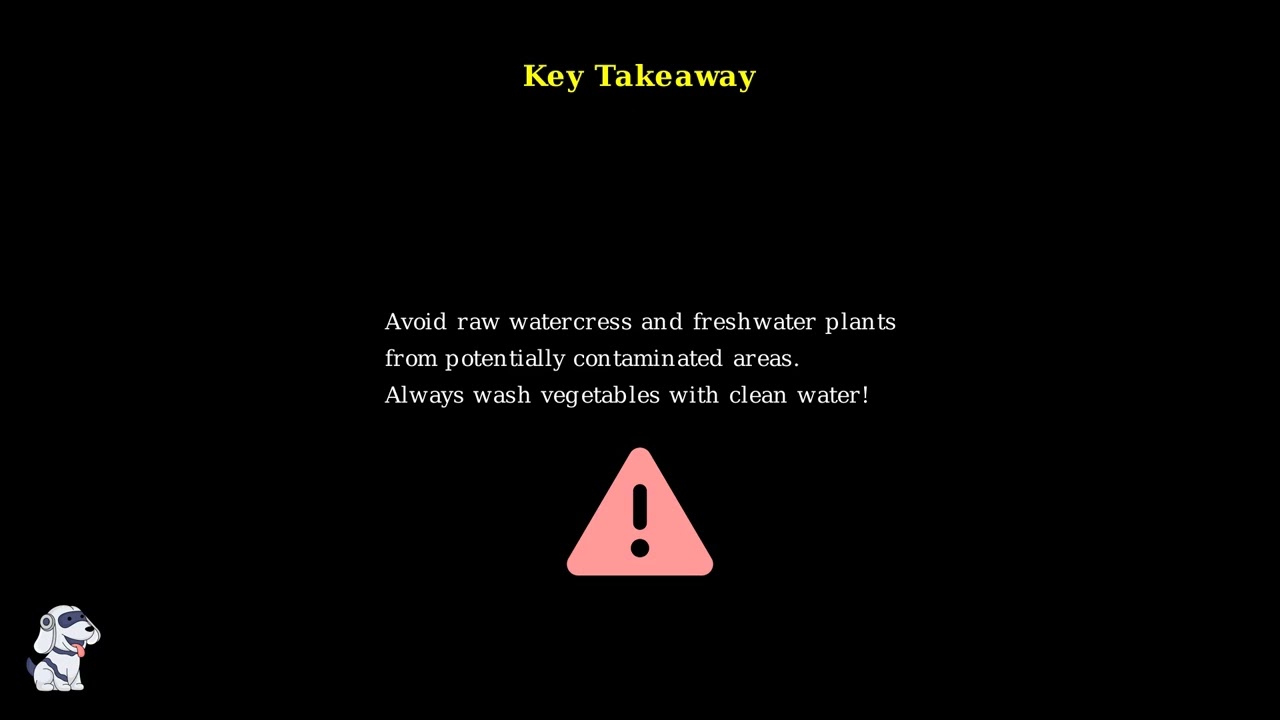
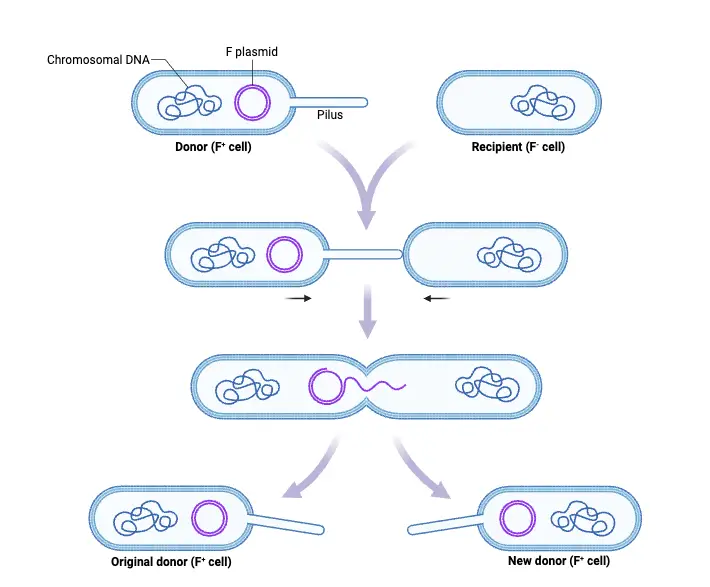
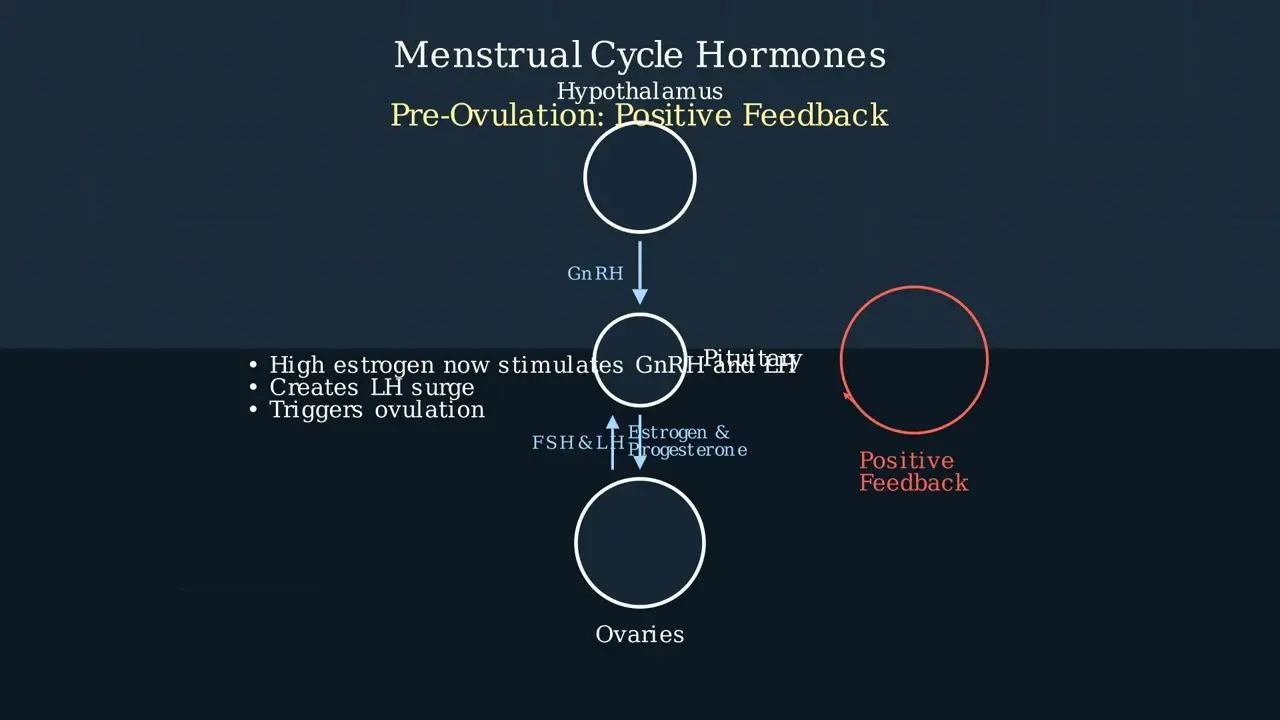
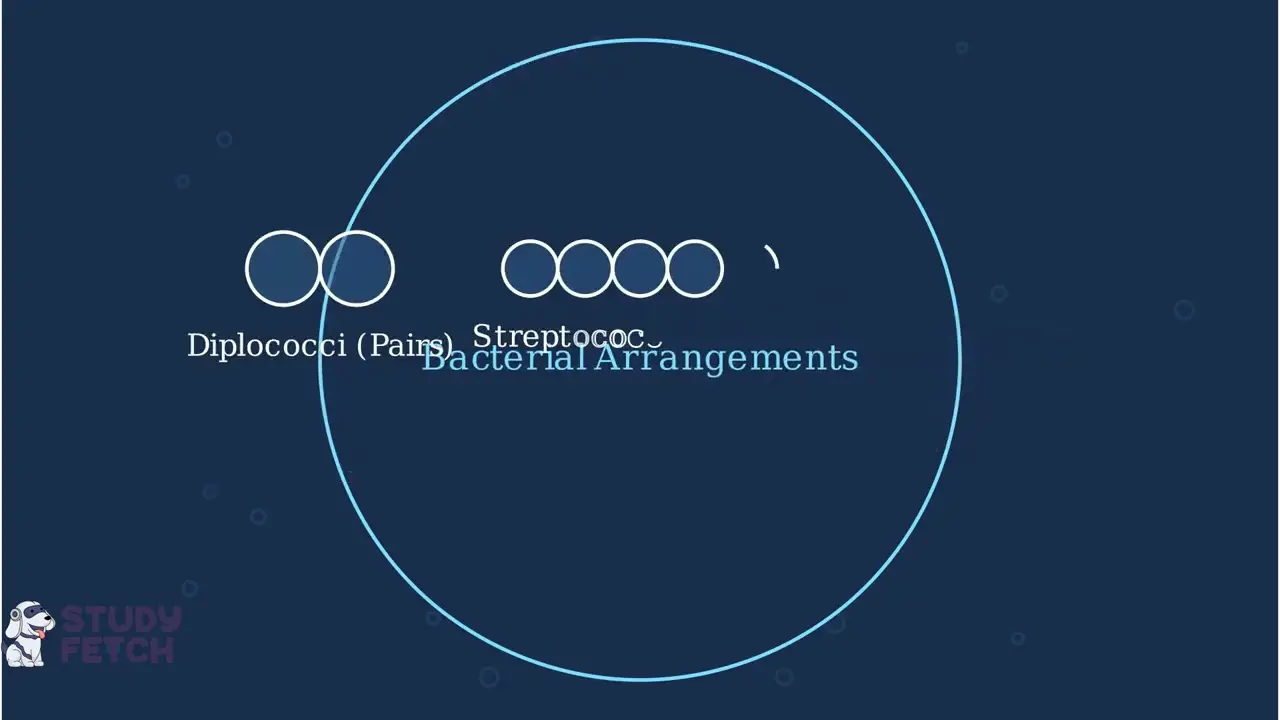
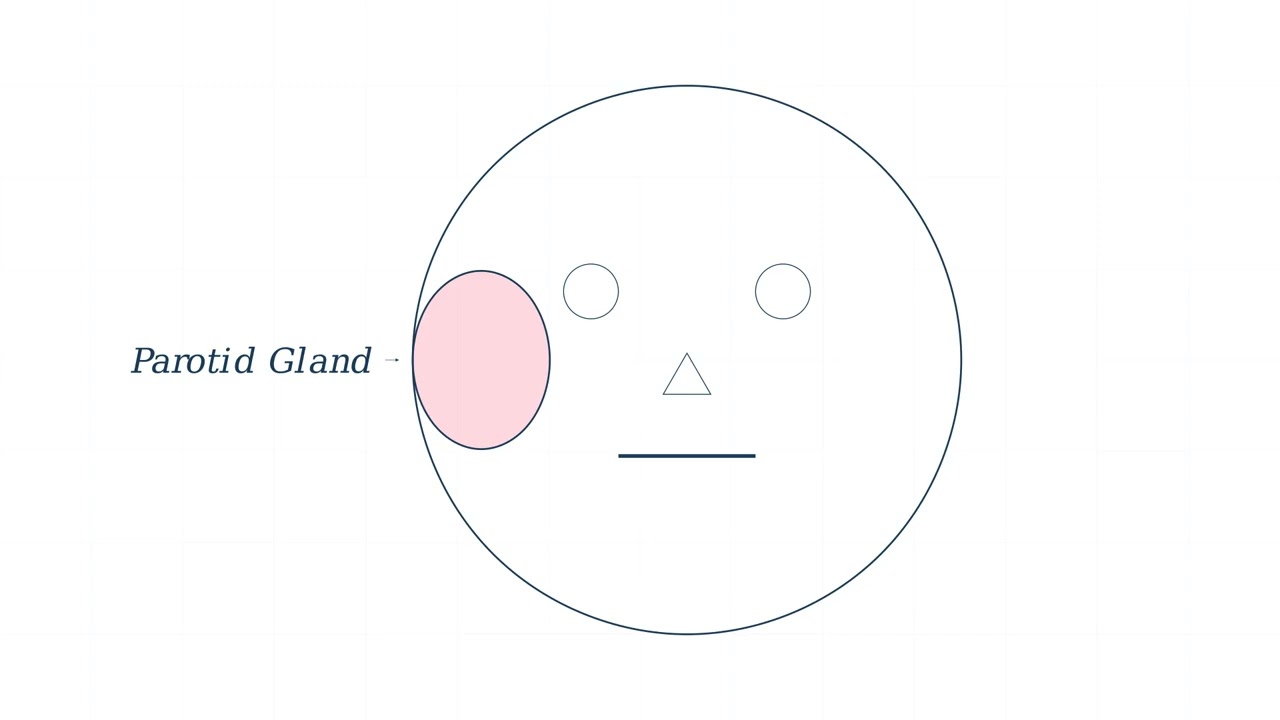
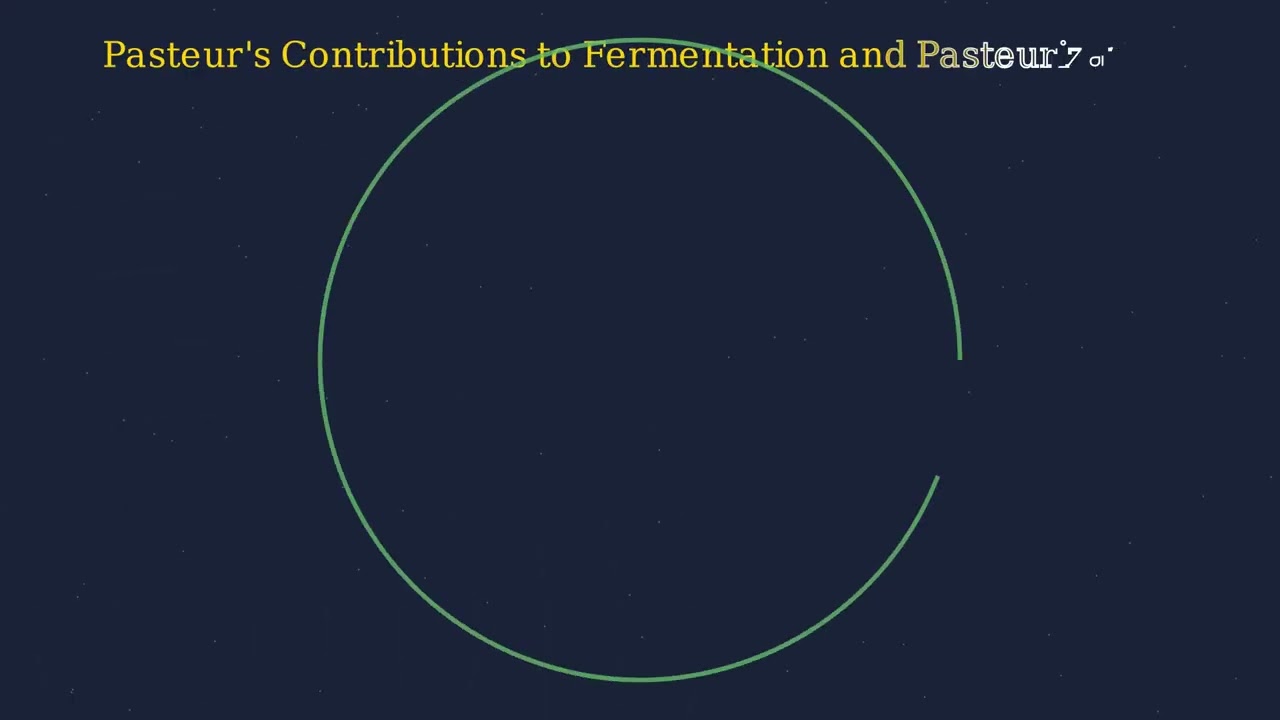